1 Introduction
Heavy metal fluoride glasses are well known as promising materials for IR-transmitting fibers and rare earth laser hosts 〚1〛. Among several families of compounds leading to stable glasses, fluoroindate glasses have received special attention due to their improved optical and mechanical properties compared to the most studied fluorozirconate glasses 〚2, 3〛. The major drawback is the still high thermodynamic tendency to crystallization and in this context fluorophosphate glasses have been considered. The addition of small quantities of selected phosphates to the glass compositions leads to much more stable glass samples with loss in some of the favorable optical properties. The optimization of the compromise between the loss in optical quality in the IR and improvement in mechanical properties is the glassmaker’s challenge depending on specific applications 〚4〛. We have thus studied the stabilization of fluoroindate glasses by the addition of scandium polyphosphate.
In this sense, the structural approach of the fluorophosphate glasses has been studied. We could note the existence of two different kinds of structures depending on the ratio of polyphosphate/fluor. These considerations are discussed by 31P NMR and Raman spectroscopy.
2 Experimental
2.1 Scandium polyphosphate synthesis
Phosphoric acid 85%, analytical purity grade, (Mallinckroft) and scandium oxide spectral and analytical purity grade (Rosreactiv and Fluka) were used as raw materials. 〚Sc(PO3)3〛n was prepared according to a previously published method 〚5〛 with some modifications concerning the heating scheme. Crystalline phosphoric acid obtained by freezing with liquid nitrogen, and Sc2O3 were ground together under constant cooling until a fine powder was obtained. It was heated at low temperature (120–210 °C) until water-vapor evolution was over and then transferred into a furnace. Heat treatment was performed for 2 h at 600 °C and then for 3.5 h at 1000 °C.
2.2 Glass preparation
Glasses were prepared by using BaF2 (an Aldrich product), a scandium polyphosphate previously described and an indium fluoride synthesized by a conventional technique using NH4F·HF and In2O3 (an Aldrich product), as described elsewhere 〚6〛. Syntheses were carried out by melting starting materials in platinum tubes in an electric furnace for 30 min at 1100 °C. Compositions containing more than 30% of 〚Sc(PO3)3〛n were melted using an induction furnace. After heating, the melts were quenched to room temperature between brass pieces. Typical nominal glass compositions are given in Table 1. For the most stable compositions, the melt was cooled into a mold pre-heated at Tg temperature and samples with up to 3-mm thickness were obtained.
Glass compositions (in mol%) and their characteristic temperatures.
Samples | InF3 | BaF2 | 〚Sc(PO3)3〛n | Tg | Tx | Tp | Tx – Tg | F/O |
V17 | 50 | 40 | 10 | 401 | 486 | 506 | 85 | 2.56 |
V18 | 40 | 50 | 10 | 418 | 492 | 502 | 74 | 2.44 |
V19 | 30 | 60 | 10 | 421 | 471 | 480 | 50 | 2.33 |
V20 | 20 | 70 | 10 | 410 | 461 | 470 | 51 | 2.22 |
V21 | 10 | 80 | 10 | 396 | 437 | 445 | 41 | 2.11 |
V22 | 10 | 70 | 20 | 480 | — | — | — | 0.94 |
V23 | 10 | 60 | 30 | 500 | — | — | — | 0.55 |
V24 | 10 | 50 | 40 | 560 | — | — | — | 0.48 |
2.3 Characterizations
Characteristic temperatures were determined from Differential Scanning Calorimetry (DSC – TA 3100 Equipment) scans obtained from powdered samples in aluminum pans under N2 atmosphere at 10 °C min–1. Raman scattering spectra were obtained with a Renishaw micro-Raman apparatus by using the 6345-Å line of a He–Ne laser in the excitation. High-resolution 31P NMR spectra were obtained at room temperature, at a radio-frequency of 161.9 MHz (9.4 T). The Magic Angle Spinning (MAS) technique was implemented with a Varian wide-body 7-mm double resonance probe, in a Varian Unity INOVA spectrometer using 19F high power decoupling. Direct 31P-NMR and cross polarization 19F–31P experiments were carried out. Spinning frequencies up to 7 kHz were used. Side bands in the spectra were identified by varying the MAS frequency. Spectra were recorded with radio-frequency π/2 pulses of 4-μs length, recycle times up to 400 s and contact times up to 7 ms in cross polarization experiments. Chemical shifts were measured with respect to the 85% solution of phosphoric acid with accuracy better than ±1 ppm.
3 Results and discussion
Transparent and homogeneous small glass plaques were obtained. Samples have been identified as ‘glasses’ by considering together the visual inspection, the amorphous character to the X-rays and the observation of transition temperatures in DSC curves. The vitreous domain determined in this way is displayed in Fig. 1.
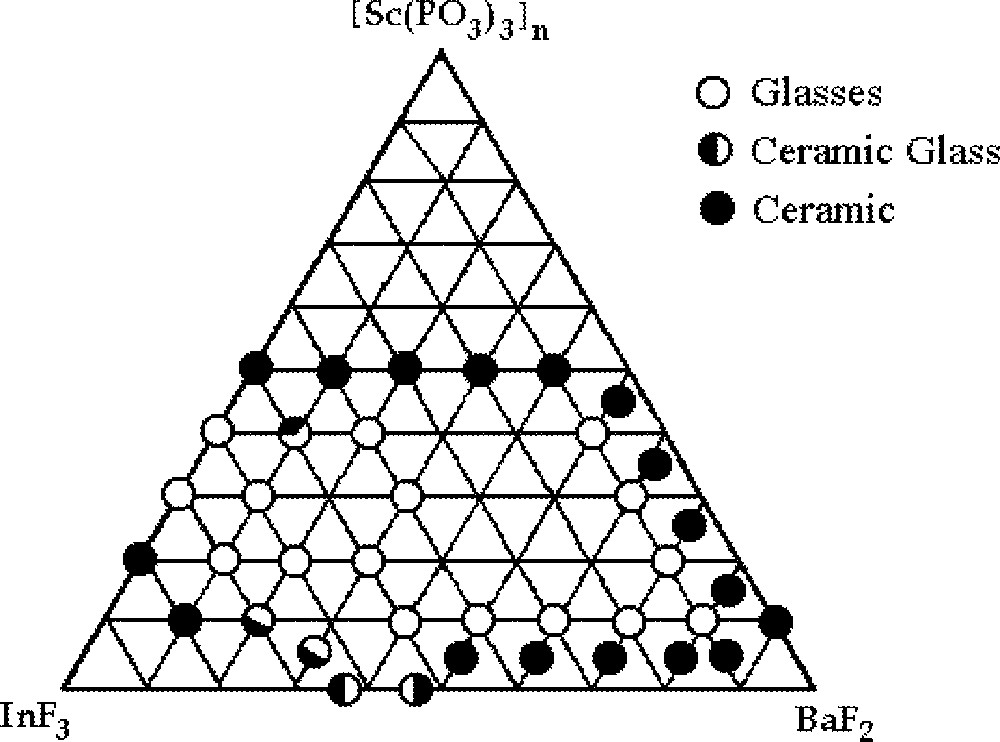
Glass-forming area for the ternary system InF3–BaF2–〚Sc(PO3)3〛n
The characteristic glass temperatures (Tg, Tx and Tc) could be identified and Table 1 gathers the results. A large glass forming area can be observed for the polyphosphate containing glasses. Samples up to 3-mm thickness could be easily obtained by slow cooling for stable compositions. Samples with up to 80% mol BaF2 were obtained. For samples with concentrations above 15% of Sc(PO3)3, it was not possible to determine Tx and Tp using DSC equipment, due to limitation in temperature of our apparatus. Experimentally, we have observed that the addition of scandium polyphosphate to the binary system, InF3–BaF2, has led to glass samples displaying higher thermal stability.
It must be pointed out that a similar behavior was observed before concerning the related indium polyphosphate. Based on DSC and vibrational spectroscopy results, we could suggest that stabilization against crystallization was achieved with the formation of fluorophosphate units bridging 〚InF6〛3– groups supposed to exist in fluoroindate glasses 〚7〛. The same kind of stabilization would be operative here due to the chemical similarity between indium and scandium polyphosphates. Further insights into the structure of these new glasses could be suggested from thermal analysis and vibrational spectroscopy, as stressed hereafter.
First we discuss the results obtained for the samples where the content in 〚Sc(PO3)3〛n was kept constant at 10% mol (samples V21–V17). Starting with the sample V21 (80% mol BaF2–10%mol InF3), Tg is observed at 396 °C, Tx at 436oC and Tp at 444 °C. Both the glass transition and the crystallization temperatures shift to higher temperatures as InF3 is substituted for BaF2. For the sample V20 containing 20% InF3, Tg = 409 °C, Tx = 461 °C and Tp = 469 °C. For 30% InF3 (sample V19) Tg = 420 °C, Tx = 470 °C and Tp = 479 °C. A second exotherm appears at higher temperature around 500 °C for this last sample. With increasing substitution of InF3 for BaF2, only the higher exotherm is observed (501 °C) and the Tg value decreases to 418 °C for the sample with 40% InF3 (sample V18). Finally, for the sample V17 with 50% InF3, Tp = 506 and Tg is still shifted to lower temperatures (400 °C). Fig. 2 shows Tg values as a function of the InF3 content. The maximum observed for samples with 〚InF3〛 40–50% mol may be related to specific structural features discussed below from the vibrational spectroscopy data.

Evolution of Tg as a function of InF3 content.
Now we turn for the samples where InF3 content is kept constant at 10% (samples V21 to V24). The substitution of 〚Sc(PO3)3〛n for BaF2 leads to a drastic increase in characteristic temperatures. Tg = 480, 500 and 560 °C for the samples with 20, 30 and 40% mol 〚Sc(PO3)3〛n respectively. Crystallization temperatures could not be observed for these samples, due to the temperature limits of our experimental setup.
Interesting enough these two series for glass samples constant 10%mol InF3 and constant 10%mol 〚Sc(PO3)3〛n constitute in fact two families of fluorophosphate glasses with different structural features inside the same vitreous domain, as it will be discussed above, where structural information is obtained from Raman spectra.
Fig. 3 shows spectra obtained for the samples where the 〚Sc(PO3)3〛n is kept constant at 10%mol. Starting with sample V17 (50%InF3) a strong peak is observed at 500 cm–1. With decreasing InF3 a continuous red shift is observed. For sample V20, this peak appears at 489 cm–1.
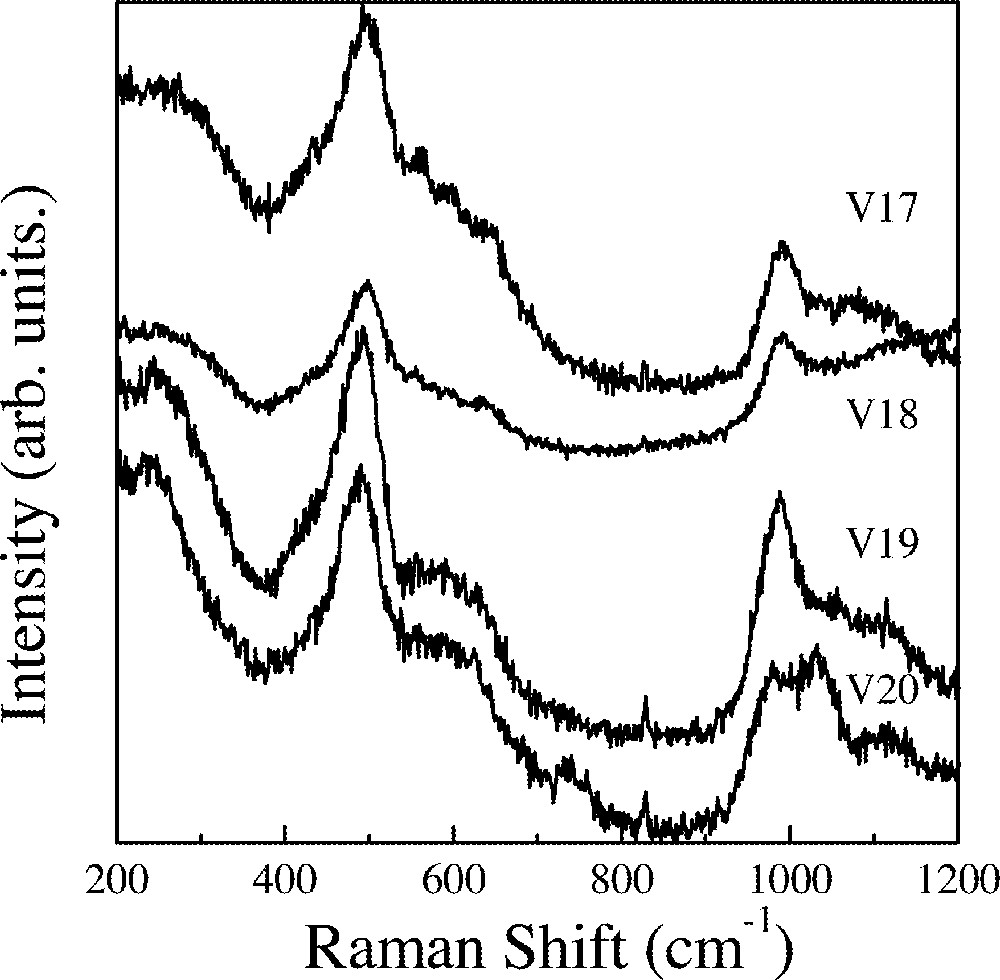
Raman spectra of the V17 to V20 glass samples.
These bands can be assigned to vibrational modes of the octahedral units 〚MF6〛 (M = In,Sc). The red shift could be related to the interactions between phosphate and 〚(In,Sc)F6〛 groups.
A broad band extending from 540 to 700 cm–1 is also observed for these three samples. They could be tentatively assigned either to ν4 vibrational modes of isolated phosphate groups or the symmetric stretching of P–O,F bonds in P(O,F)4 units. A strong band peaking at 990 cm–1 is observed for the three samples and could also be assigned either to isolated phosphate groups or fluorinated P(O,F)4 units, as it was done before 〚7〛. A weaker and broader component extending to higher frequencies is observed for the three samples. The spectrum obtained for sample V19 also displays a second high frequency band peaking at 1030 cm–1.
Fig. 4 shows spectra obtained for samples where the InF3 content is kept constant that is, sample V21 with 10% 〚Sc(PO3)3〛n, sample V23 with 20% 〚Sc(PO3)3〛n, and sample V24 with 30% 〚Sc(PO3)3〛n. The first observation relates to the relative decrease in intensity of the characteristic 〚(In,Sc)F6〛 peak at around 500 cm–1 at expenses of the increase in intensity of the phosphate related bands. New weak bands appear at 338 and 534 cm–1 and they could be tentatively assigned to InOF vibrational modes. In the preparation of InF3, we have been observing that the incomplete fluorination of In2O3 may be identified by the appearance of these oxyfluoride typical bands in Raman spectra. The increase in the ratio O/F would lead to the preferential formation of these species.
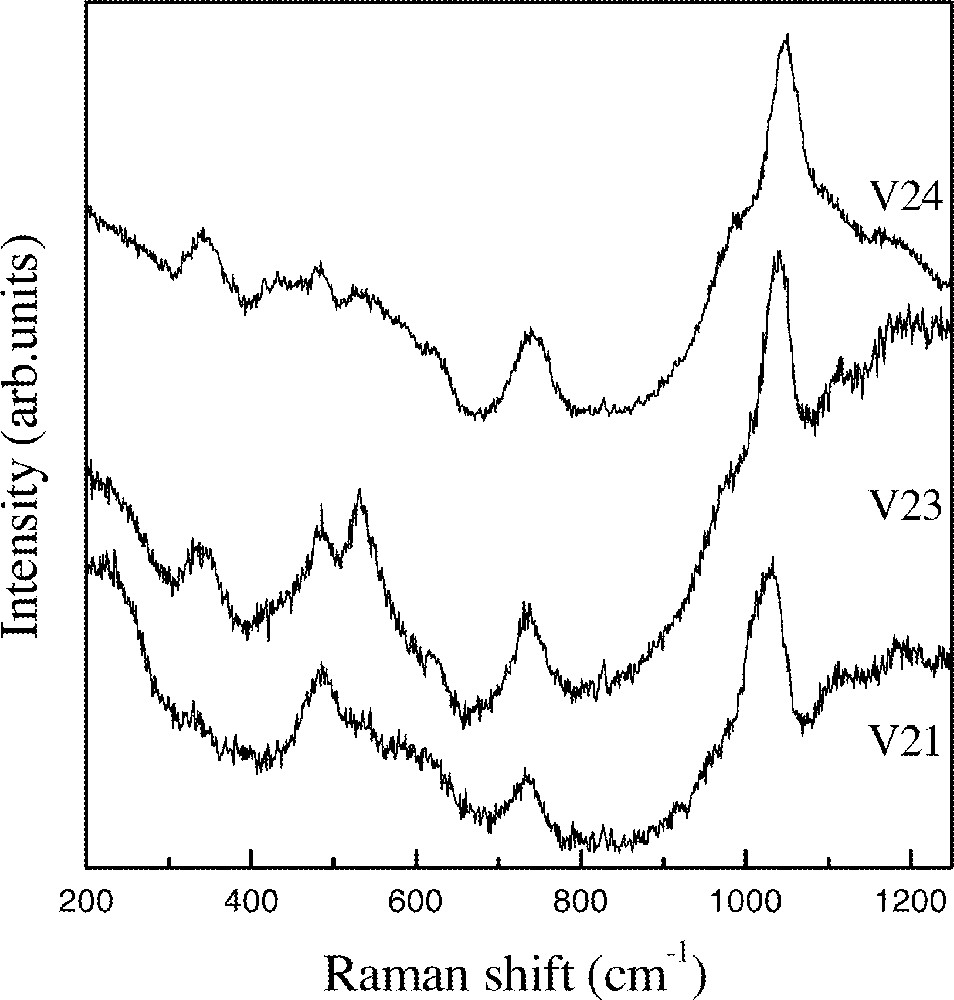
Raman spectra of V21 to V24 glass sample.
In the middle range of the spectra also a new band appears between 730 and 740 cm–1, which is the characteristic region of vibrational modes related to P–O–P bonds in condensed phosphates 〚8〛.
A clear evolution is also observed in the high-frequency region. The second peak already observed for sample V20 increases in intensity and is blue-shifted with the increase in 〚Sc(PO3)3〛n content. For sample V21 it appears at 1031 cm–1, for sample V23 at 1040 cm–1 and for sample V24 at 1049 cm–1.
The energy position for this high-frequency peaks is clearly related to the In/Sc atomic ratio. In our previous work this band was observed at 994 cm–1 for samples in the system In(PO3)3–InF3–BaF2. The observation of the same energy position for the samples V17, V18 and V19 suggest the same structural unit. The appearance of the second peak for the sample V19 and the increase in intensity and blue shift with the increase in 〚Sc(PO3)3〛n content suggest a different nature for the structural units present in these glasses. It appears together with the P–O–P band at 730–740 cm–1 and so it must related to polyphosphate like units. As it is well known for polyphosphate glasses, the blue shift must be linked to the increase in the P–O bond order as the polymerization degree increases 〚9〛.
P–F bonds are difficult to be identified due to the overlap between frequencies due to P–O and P–F bonds. In the literature, some authors claim to observe P–F vibrational modes at 830 cm–1. A small feature is indeed observed at exactly this frequency for all our samples but its rather weak intensity may lead to precipitate conclusions. In order to clarify these aspects, NMR spectra of 31P is performed.
Fig. 5 shows the 31P NMR spectra obtained for the set of glasses and a polycrystalline sample of 〚Sc(PO3)3〛n. Four peaks were partially resolved in the NMR spectrum of 〚Sc(PO3)3〛n at –35.7 ppm, –38.2 ppm, –45 ppm and –47 ppm, which can be attributed to different Q2 sites in the polyphosphate chain. The weak line at –11.8 ppm corresponds to a small amount of Q1 units, contributing with less than 8% of the total of phosphorous sites observed by NMR. This figure corresponds to phosphate chains with more than 26 tetrahedra in the 〚Sc(PO3)3〛n sample. On the other hand, the NMR spectra of glasses shown in Fig. 4 display a well-defined trend as a function of the fluorine content. Glasses with the lower F/O value, such as V23, exhibits a NMR spectrum composed of a broad resonance line centered at –11 ppm. From this value of isotropic chemical shift, it can be inferred that phosphates units in these glasses appear mainly as Q1 units 〚7〛. As the F/O ratio increases, a second resonance can be resolved around –1 ppm. In glasses with the highest F/O values, as V17 or V18, only the –1-ppm resonance is detected. This line can be associated with Q0 sites.
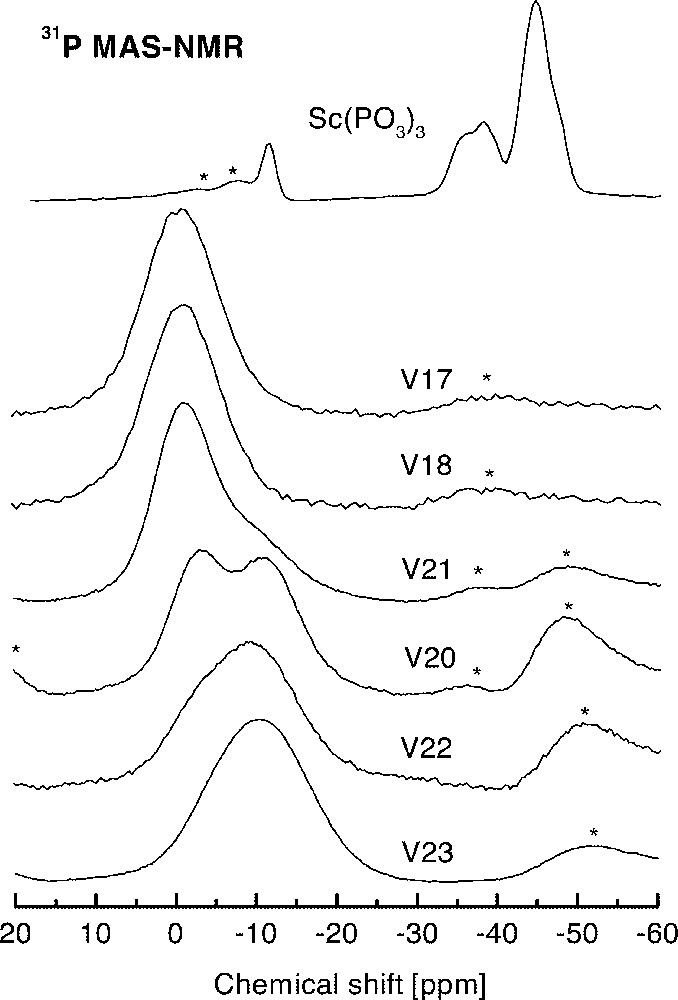
High-resolution 31P NMR spectra of polycrystalline 〚Sc(PO3)3〛n and six glasses of several compositions in the system InF3–BaF2–〚Sc(PO3)3〛n (see Table 1 for individual compositions). Asterisks identify spinning sidebands.
Regarding to the possibility of formation of P–F bonds in glasses with the highest F content, it should be noted that P–F bonds have many similarities to P–Ob–P bonds in which the oxygen atom bridges two adjacent phosphate tetrahedra. This fact results from similarities between P–Ob and P–F bond lengths, and between the electronegativity and covalent radii of F and Ob 〚10〛. Because of these reasons, the 31P chemical shift for Q0(1F) and Q1 units are similar, the latter being more shielded than the Q0 resonance 〚10〛. In the set of glasses, the observed deviation of the chemical shift with the increment of F concentration is in the opposite direction with respect to that expected for P–F bonds. Therefore, the association of the resonance at –1 ppm with Q0(1F) or Q1(1F) environments seems very unlikely.
On the other hand, cross-polarization NMR experiments between 19F and 31P were performed, in order to check the line attributions discussed above. In these experiments, a growing of 31P magnetization is developed after a π/2 pulse is applied on 19F nuclei, while 31P and 19F spins are spin-locked, due to the existence of a dipolar coupling between both species 〚11〛. It is expected a faster growing of the 31P NMR signal for phosphorous bound to fluorine, due to a smaller interatomic distance and stronger dipolar coupling with respect to the case of non-bounded P–F pairs. Fig. 6 shows the evolution of the 31P cross polarization signal as a function of the contact time with the 19F reservoir for the two peaks of the V20 glass. There are no substantial differences between the dynamics of polarization transfer between both sites. In order to quantify the polarization dynamics of both lines, it was performed a fitting of the well-known expression for the magnetization of the polarized specie, considering a characteristic contact time TPF between 31P and 19F reservoirs and the 19F spin-lattice relaxation time T1ρ in the rotating frame 〚11〛. The time constant TPF describes the initial building up of 31P polarization, depending on the P–F distance as r6, while T1ρ describes the long time decrease due to the relaxation of 19F magnetization. The resulting times are shown in Table 2, indicating that there are no substantial differences between both TPF, as would be expected if one of these lines would be associated to P–F bonds. These results are in agreement with the assumption of PO4 units interacting with non-bounded F neighbor atoms.

Intensity of the peaks at –1 ppm (squares) and –10 ppm (circles) in the 19F–31P cross-polarization spectra of glass V4 as a function of the contact time.
Characteristic times describing the 19F–31P cross-polarization dynamics of the NMR signals in V4 glass. TPF: contact time between 19F and 31P reservoirs. T1ρ: 19F spin–lattice relaxation time in the rotating frame.
NMR Peak | TPF 〚ms〛 | T1ρ 〚ms〛 |
–1 ppm | 0.67 ± 0.06 | 10 ± 1 |
–10 ppm | 0.79 ± 0.05 | 11 ± 1 |
This preliminary work suggest different structural features for the two families of glasses studied in the system InF3–BaF2–〚Sc(PO3)3〛n. For the first family, where 〚Sc(PO3)3〛n is kept constant at 10% mol, octahedral 〚InF6〛 units have been identified. Interactions between these octahedra and phosphate groups are evidenced by the red shift observed for the characteristic A1g vibrational mode. As proposed before, the structural unit could be described by phosphate-linked 〚InF6〛 octahedra (Fig. 7). The high F/O ratio suggests fluorine substituting for oxygen atoms in the phosphate tetrahedra.

Structural unit for the family of glasses studied in the system InF3–BaF2–〚Sc(PO3)3〛n where 〚Sc(PO3)3〛n is kept constant at 10% mol, described by phosphate-linked 〚InF6〛 octahedra. The high F/O ratio suggests fluorine substituting for oxygen atoms in the phosphate tetrahedra.
The other family where InF3 content is kept constant at 10% mol is better described by a model considering condensed phosphates (Fig. 8).
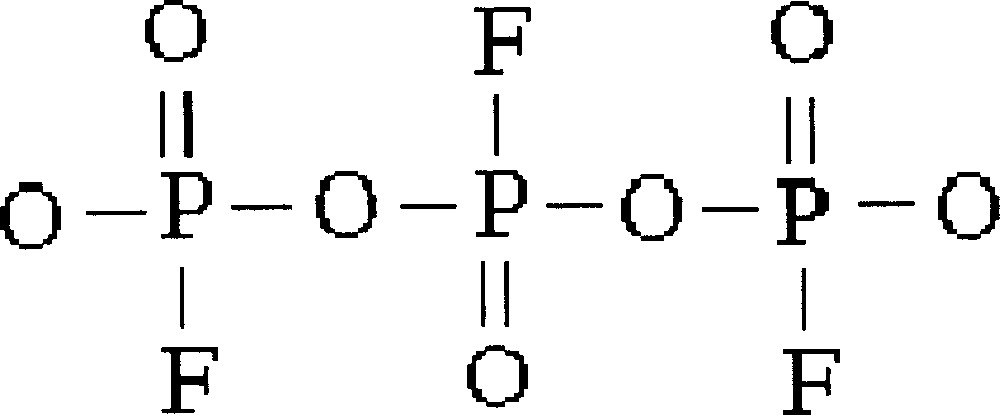
Structural unit for the family of glasses studied in the system InF3–BaF2–〚Sc(PO3)3〛n, where InF3 content is kept constant at 10% mol, described by a model considering condensed phosphates.
The value for n and the extent to which fluorine substitutes for oxygen atoms in the tetrahedra depends on the F/O ratio.
4 Conclusion
Appropriate cryo-technique have been used for 〚Sc(PO3)3〛n synthesis. The addition of scandium polyphosphate was observed to stabilize glasses against crystallization and thermal analysis and vibrational spectroscopy suggest that at least two families of glasses could be described in the fluorophophate system. The first one includes fluorinated phosphate units linking octahedral 〚InF6〛 groups. The second one is better described by a simple polyphosphate chain where the fluorination depends on the F/O ratio. From the high-resolution 31P NMR study of fluorophosphate glasses, it can be concluded that phosphate groups appear only as Q0 and Q1 units. For low-fluorine concentrations, PO4 groups seems to be coordinated to Sc, as indicated by the agreement between the central value of the chemical shift in glasses with the small Q1 peak observed in polycrystalline ScPO4. For glasses with higher fluorine concentration, a peak from Q0 units is progressively resolved in the NMR spectra. On the other hand, the existence of P–F bonds seems to be unlikely, considering the disagreement between the observed chemical shifts and the expected trends for Q0(1F) or Q1(1F). Also, the attribution of the observed NMR lines is compatible with the similarity between the TPF times in the cross polarization dynamics of both sites.
Acknowledgements
The financial support of the Brazilian sponsoring agencies Fapesp, CNPq and CAPES is gratefully acknowledged.
Vous devez vous connecter pour continuer.
S'authentifier