1 Introduction
The efficient delivery of biologically active compounds into cells has been accomplished by the use of a wide variety of vesicles. Liposomes are a particular kind and one of the most developed types of vesicles, constituted by amphipatic molecules containing one or more hydrophobic chains, one hydrophilic region and a linker between these two. The cavity of the liposomes can be filled with drugs or other therapeutic agents and it is entirely enclosed by a membrane constituted by bilayer amphipatic molecules.
Cationic liposomes are good candidates as gene carriers in cell biology, due to their ability to bind DNA through electrostatic interactions [1]. Endocytosis seems to be the principal pathway by which liposomes enter cells [2,3] and it was reported that the pH of endocytotic vesicles is slightly acidic. Moreover, pH-sensitive liposomes have been extensively studied as vehicles for cytoplasmic delivery of drugs and nucleotides [4–7] due to their ability to bind different amount of DNA at various pH. It is believed that these liposomes would be destabilized in the endocytotic vesicle and fuse eventually with the endosome membranes, thereby releasing more easily the entrapped contents into the cytoplasm before they are destroyed in lysosomes [8] This pH-dependent membrane fusion was recently shown [9].
Cationic liposomes are also used as non-viral delivery systems in the gene therapy of glioma [10–12]. In the context of this research strategy, pH-dependency and transfection efficiency in rat C6 glioma cells line of seven novel liposomes [13] were studied. The corresponding lipids (Fig. 1) have a polar head group consisting of a tertiary amine as a common feature and one or two pentadecylcarbamate or 8-heptadecenylcarbamate esters hydrophobic chains. In particular, three of these lipids (MORF-1, MORF-2 and MORF-3) bear a morpholine group, while other two (PIPR-1 and PIPR-2) have a piperazine moiety in their backbone. The latter two have also a secondary amine group in their polar head that can protonate at a certain pH value. Another lipid MM54 has some similarities with the commercially available DOTAP, one of the most commonly used lipids in mixture with DOPE in transfection experiments. Another lipid (DC-Amy) has a β-Amyrine moiety in its hydrophobic backbone, while the linker and the polar head group are the same as in the commercial DC-Chol.
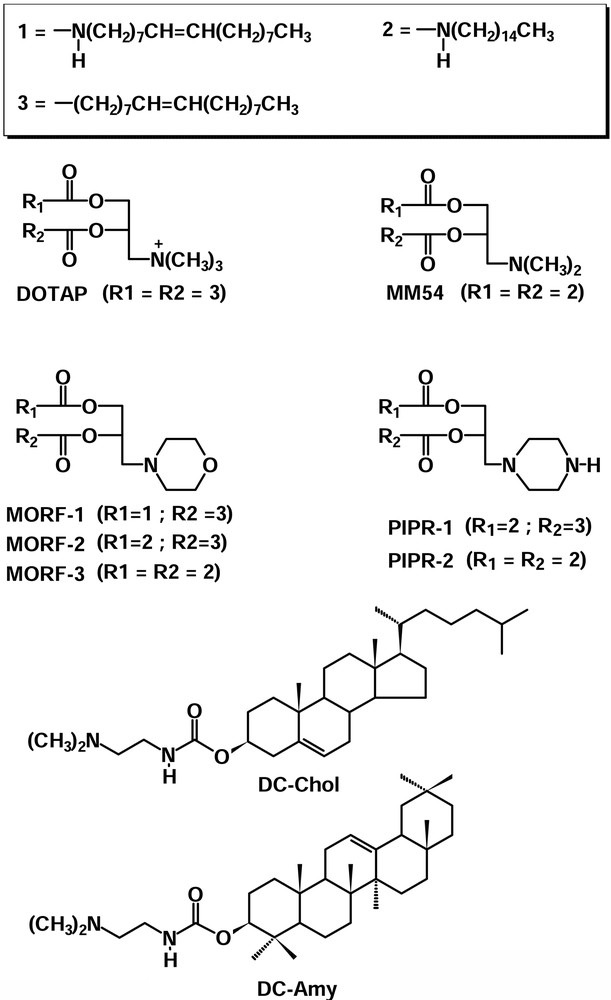
Structural formulas and abbreviations for the novel and commercial cationic lipids.
2 Materials and methods
Lipids and the corresponding liposomes are indicated in the text with the same acronyms.
Liposomes were prepared by dissolving the corresponding lipids and an equimolar amount of DOPE (1:1 molar ratio) in ethanol, followed by injection in sterile water under vigorous stirring [14] to reach a final concentration of 1 mg ml–1.
The pGL3-Control Vector plasmid (PROMEGA), containing a modified coding region for firefly (Photinus pyralis) luciferase, was used for all lipofection experiments. Plasmids were extracted and purified by using the EndoFree Plasmid Purification Kit (QUIAGEN).
Complexation of DNA with the liposomes was obtained by incubating pGL3 and liposomes in DMEM (Dulbecco's Modified Eagle's Medium) for 30 min at room temperature. Liposome and DNA concentrations are expressed as molarity.
Liposomes and liposome/DNA complexes dimension was measured by light scattering experiments performed using a classic set up equipped with a correlator (BI 9000 AT, Brookhaven), operating with a logarithmic sample time spacing.
Rat C6 glioma cell line was cultured in DMEM supplemented with 10% FCS, 2mM L-glutamine and antibiotics (100 U ml–1 penicillin and 100 mg ml–1 streptomycin) at 37 °C in a humidified atmosphere containing 5% CO2. Cells were plated in black Wallac 96-well plates for tissue culture at a density of 4 × 104cells/well and grown overnight up to 80% confluence. Cells were washed with DMEM (FCS free) and the complexes were added. Transfection was allowed to proceed for 4 h at 37 °C, in a humidified atmosphere containing 5% CO2. At the end of the transfection process, the lipoplex solution was replaced with complete fresh culture medium.
The transfection efficiencies were measured by means of the luciferase assay monitored with a Wallac 1420 Victor2 multilabel counter (PerkinElmer) after 48 h from transfection.
Agarose gel electrophoresis and buffer solutions were prepared by standard procedures.
3 Results and discussion
As previously stated, lipids bear a polar head group consisting of a tertiary amine and, in some cases, even of a secondary one (PIPR-1 and PIPR-2). The amine groups can easily protonate at acidic pH, while at alkaline pH the polar head groups are neutral, giving rise to neutral lipids. These characteristics emphasize the behaviour of these novel lipids as suitable compounds for the preparation of pH-dependent liposomes.
In particular, three of these lipids (MORF-1, MORF-2 and MORF-3) bear a morpholine group while other two (PIPR-1 and PIPR-2) have a piperazine moiety in their backbone. The latter two have also a secondary amine group in their polar head that can protonate at a pH value higher than the tertiary amino group. At lower pH values, the morpholine derivatives can accommodate on their polar heads only one proton, while the piperazine derivatives can accommodate up to two. As a consequence, at acidic pH values, the latter lipids can accomplish a higher affinity towards DNA than that of the morpholine derivatives. Another lipid MM54 has some similarities with the commercially available DOTAP, one of the most commonly lipid used in mixture with DOPE in transfection experiments. The major difference between DC-Chol and DC-Amy is the presence of a β-Amyrine moiety in the hydrophobic backbone of DC-Amy, while the linker and the polar head group are the same of DC-Chol.
Liposomes size was measured by light scattering experiments and results are reported in Table 1. PIPR-1 and PIPR-2, bearing a piperazine moiety in their polar heads, have a mean diameter smaller than that of MORF-1, MORF-2 and MORF-3. This evidence indicates that the modification of the polar head, replacing a morpholine instead of a piperazine moiety, has a slightly appreciable effect on the liposome size. MM54 forms liposomes bigger than that prepared by its analogous DOTAP and this is ascribable either to a different functionalization of the head group or to a different side chain of the lipid moiety (carbamate instead of ester linkage). The former topic can lead to a different charge distribution on the liposome surface that can influence also its dimension, while the latter can lead to a different spatial conformation due to a modified stereochemical hindrance. Moreover, MM54 bears a tertiary amino group that can be differently protonated in aqueous solution upon varying the pH, while DOTAP cannot, owing to the presence of a quaternary ammonium nitrogen. The size of liposomes prepared by DC-Amy and DC-Chol is quite similar. This may be due to a to a similar structure of the two lipids.
Mean diameters (nm) of the novel synthesised pH-dependent liposomes measured by dynamic light scattering compared to that of DOTAP and DC-Chol
Liposome | Mean diameter (nm) |
PIPR-1 | 145 |
PIPR-2 | 122 |
MORF-1 | 240 |
MORF-2 | 214 |
MORF-3 | 420 |
DC-Chol | 146 |
DC-Amy | 120 |
DOTAP | 125 |
MM54 | 290 |
In order to study the complexation behaviour of the novel pH-sensitive liposomes with DNA, lipoplex solutions were prepared at different buffered pH and run in 1% TBE agarose gel containing ethidium bromide. To better investigate the pH-dependence of these lipids, PIPR-2 was chosen as a model lipid due to the possibility to form up to a diprotonated species in solution, causing a linear variation of DNA complexation by varying the pH. Moreover, a charge ratio 2:1 was chosen to evidence the lack of DNA complexation at alkaline pH, even in excess of liposome. Fig. 2a shows the DNA complexation behaviour of PIPR-2 (PIPR-2:DNA 2:1) at various pHs. The pH-dependent cationic liposome shows a better affinity with DNA at acidic pH values, owing to the increasing charge on the polar head group, leading to stronger interactions. Fig. 2b shows the complexation behaviour of PIPR-2 at different liposome:DNA charge ratio. A better complexation is observed at a higher charge ratio (Fig. 2b) where the amount of lipoplex present in the well is greater and only a little amount of uncomplexed DNA migrates through the gel.
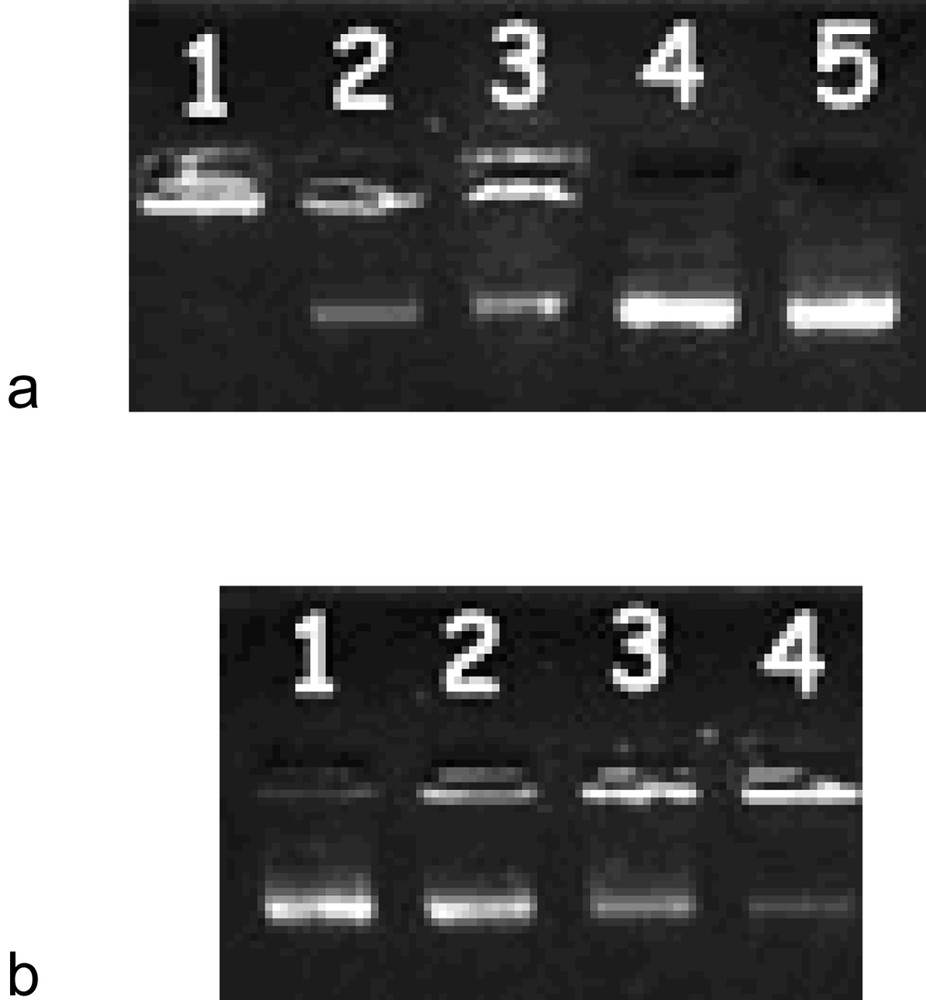
Electrophoresis in 1% agarose gel with 0.5 μg ml–1 ethidium bromide. (a) PIPR-2:DNA (2:1) lipoplex at different pHs. Lanes 1–5 correspond to pH = 3, 5, 7, 9 and reference DNA, respectively. (b) PIPR-2:DNA (2:1) lipoplex prepared in DMEM at different molar ratios. Lanes 1–4 correspond to 1:1, 2:1, 3:1 and 4:1 charge ratios, respectively.
These evidences merely concern the acid-base properties of these novel molecules and show how they can differently bind to DNA at different pH values during complex formation, and not during internalisation and transfection processes.
On the other hand, the pH-dependency of some recently-reported liposomes and polymers [15-33] shows that the acid-base properties of such compounds inside the endosome are relevant in terms of buffering the decreased pH after lipoplex internalisation and/or assuming better fusogenic properties that allow a better DNA release from the endosome itself.
The intracellular delivery mechanism of such novel pH-dependent liposomes and their pH-dependency into the cell (DNA complexation and release by varying the endosomal pH) is beyond the aim of the present study and surely requires a deeper study and the aid of more sophisticated techniques, like confocal fluorescence microscopy and atomic force microscopy.
For these reasons, in order to normalize and standardize the lipoplex preparation, complexes were allowed to form in DMEM for transfection experiments.
In order to study the in vitro transfection efficiency of these novel vectors toward a glioma cell line, and to investigate their in vivo transfection by using an animal model (rat model) in a next paper, a suitable C6 cell line was chosen and studied.
Fig. 3 shows the transfection efficiency of different lipoplexes in C6 glioma cells reported as a function of liposome:DNA charge ratio. Transfection efficiencies of lipoplexes formed by the morpholine derivatives and PIPR-1 are very low compared to that of commercial DC-Chol and DOTAP. Considering the morpholine liposomes, the chemical modifications of the linker moiety and/or of the hydrophobic chains seem to have no enhancing effect on the transfection efficiency, suggesting that only the polar head group may play an important role in this process. PIPR-2 shows remarkably higher transfection efficiency at a liposome:DNA (4:1) charge ratio, than that of DC-Chol and DOTAP at the same ratio. On the contrary, the transfection efficiency of DC-Amy is much lower than that of DC-Chol at all considered charge ratios. This unexpected anomaly will deserve a deeper investigation because the hydrophobic moiety of this lipid is similar to that of DC-Chol. Finally, the transfection efficiency of MM54 at the 2:1 charge ratio is higher than that of DOTAP, while the transfection at 3:1 charge ratio is comparable. Considering that the hydrophobic part of MM54 is very similar to that of MORF-3 and that the polar head group is constituted by a tertiary amine in both cases, it seems reasonable to think that the higher transfection of MM54 can be ascribed to a smaller steric hindrance of its head group.

Transfection efficiency of different lipoplexes in C6 glioma cells reported as a function of liposome:DNA charge ratio. The transfection efficiency was evaluated by luciferase luminescence assay and expressed as counts per second (CPS).
In the case of PIPR-2 and MM54, the transfection efficiency at various charge ratios is dependent on the amount of complexed DNA, as can be derived from Figs. 2 and 3. Finally, it was not possible to unambiguously determine why these two liposomes have a greater activity owing to the complexity of transfection mechanism. Moreover, to complicate the transfection pattern, the liposome/DNA complex formation is almost always accompanied by a microcrystalline aggregate formation of discrete size (from 1000 to 5000 nm as measured by light scattering experiments), observed in all lipoplexes considered herein. These findings did not allow us to determine unambiguously the reasons of such different transfections. Hence, lipoplexes dimension, phase transitions and degradation of DNA inside cells are all factors that will be taken into account in more details in a future study, in order to better understand the translocation and transfection mechanisms of these novel pH-dependent liposomes.
Acknowledgements
This work was supported by grants from the Italian Ministry of Instruction, University and Scientific Research (MIUR).