1 Introduction
Polymeric thin films on polymer supports are a technologically important class of materials with applications in solid-state photonics, microelectronics, biotechnology and membranes [1–4]. With tremendous developments in nanoscience, the control of the thickness in nanometer scale is a main issue in membranes. In the fabrication of practical membranes, the basic requirement is obtaining a minimum resistance to flow (i.e. high permeance) through the membrane coupled with a maximum selectivity. The permeance can be generally improved through a composite membrane, where a thin dense top layer is supported by a porous sub-layer. Since the thickness of the top selective layer determines the separation performance in a composite membrane, the resistance to flow can be reduced by the formation of the selective layer in nanometer thickness. Moreover, thin-film formation at the nanometer scale can be prepared by well-controlled nanometer-sized materials, such as dendrimers, having many peripheral functional groups. A variety of strategies has been developed for attaching dendrimers to the surface of a substrate [5–11]. We introduced a simple method to prepare organic films on a polymeric support [12–16] and prepared the films to be a macromolecular electrolyte by incorporating metal salts.
We chose a macromolecular (or polymer) electrolyte as a selective layer material to characterize the effect of nanometer thickness layer on the polymeric support. Macromolecular electrolytes containing silver ions are of interest because of their potential application to facilitated transport membranes for the separation of olefin/paraffin mixtures. It has been understood that silver salts confined to polymers containing polar groups such as oxygen, nitrogen, and sulfur, form reversible complexes with olefin molecules. Olefin molecules donate π electrons from the occupied 2p orbitals to the empty s orbitals of silver ions to form σ bonds. Back-donation of electrons from the occupied d orbitals of silver ions into the empty π*–2p antibonding orbitals of olefin molecules results in π bonding [17]. Such reversible and specific interactions of silver ions with olefin molecules [18] leads to carrier-mediated transport in addition to normal Fickian transport in the solid [19–21] and liquid [22–25] states. This paper summarizes the results on macromolecular electrolyte thin films on polymer supports by using dendrimers, star shaped polymers and brushes, prepared by a simple method developed in our laboratory.
2 Dendritic thin films
2.1 Dendrimer layers on polymer films [12]
Thin layers of amine-terminated Starburst polyamidoamine (PAMAM) dendrimers were prepared on plasma-treated poly(dimethylsiloxane) (PDMS) substrates. The surface of the PDMS substrate was modified in the presence of maleic anhydride (MAH) by a simple plasma technique. The chemical composition of the surface was mostly succinic anhydrides (SA) [15, 26–27], which were subsequently allowed to react with the amine groups in PAMAM dendrimers (D). Dendrimers in methanol solutions were cast onto the plasma-modified PDMS films (PDMS-SA), and was reacted in a 120 °C oven for an hour, to obtain dendrimer layers chemically attached on PDMS films (PDMS-SA-D) (Fig. 1).

Scheme of the formation of dendrimer layers on polymer support.
The dendritic thin films were characterized by XPS, ATR FT–IR spectroscopy and gas (propane, propylene) transport properties. Pure gases (propane [99.99%] and propylene [99.99%]) were used for the permeation experiments, and pure gas permeation properties were measured by a soap-bubble flow meter. The feed pressure was maintained at 275.6 kPa, and the permeated pressure was atmosphere. The unit of gas permeance is the GPU, where 1 GPU = 1 × 10–6 cm3 (STP)cm–2s–1cmHg–1 [28]. With increasing the amount of dendrimers on the top layer, two anhydride bands at 1850 and 1782 cm–1 for PDMS-SA-D composite films decreased, while two bands for amides at 1640 and 1550 cm–1 increased, as evidenced by FT–IR (Fig. 2). The contact angles for all treated PDMS surfaces were determined using a contact angle meter. The contact angle of deionized water on surface-modified PDMS films was measured by the static drop method at room temperature. Each reported contact angle value is the average of at least six measurements. The contact angle of pristine PDMS film is ca. 104°, and the values of PDMS-SA-Ds decreases monotonically with dendrimer loading, and reached to ca. 40° for the composite film of Fig. 2d, suggesting that the surface becomes hydrophilic. When a small amount of PAMAM dendrimer is loaded on PDMS membrane, the permeance of gas hardly changes because of the low coverage of the surface, but it decreases sharply with increasing amounts of loaded dendrimer. The significantly decreased permeance implies that the permeance of the dendrimer layer is much lower than that of PDMS.
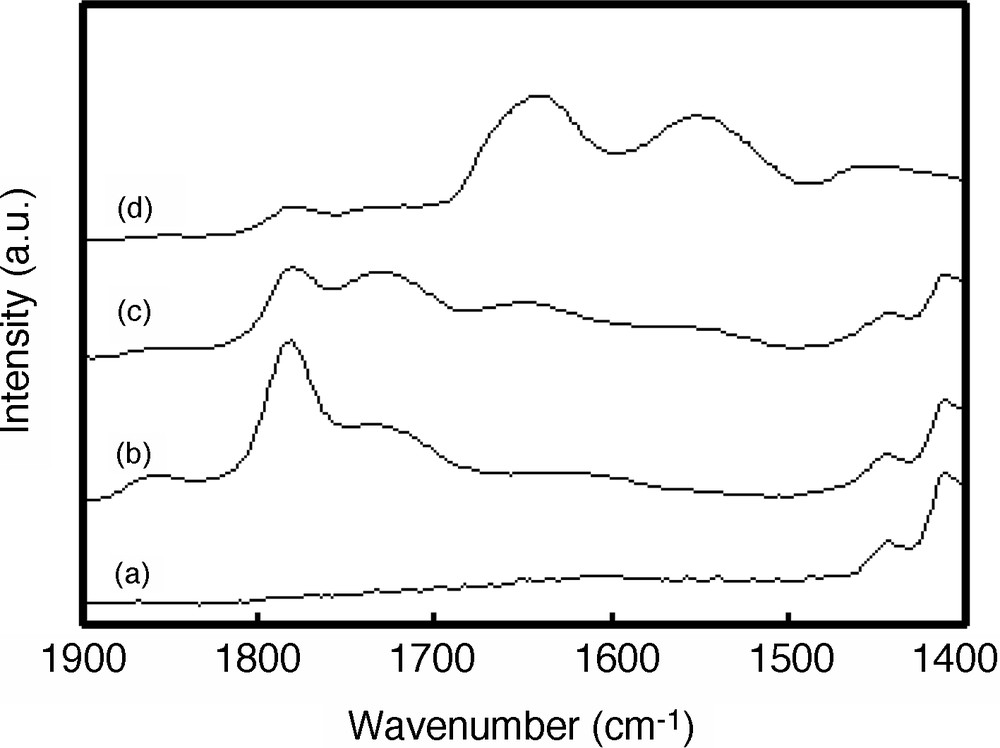
ATR FT–IR spectra of PDMS-SA-D composite films. Spectra are shown for films of (a) pristine PDMS, (b) PDMS-SA, and PDMS-SA-D deposited with (c) 3.1 × 10–11 and (d) 3.1 × 10–9 mol cm–2 of dendrimers.
The polar groups in PAMAM can interact coordinative with silver ions, forming macromolecular electrolytes. The propylene permeance was increased through the dendrimer composite membrane from 5 to 34 GPU with increasing silver ion content. This enhanced propylene permeability seems to be primarily due to the loading capability of silver salts by the dendrimer molecules and the rapid reversible reaction between silver ions and propylene. In comparison, the propane permeance through the macromolecular electrolyte film decreased with increasing silver ion content, suggesting that the film is densified upon silver coordination. Propane is unable to form complexes with silver ions and permeates only through Fickian transport. Thus, the ideal separation factor for propylene/propane is more than 340 at high silver concentrations. The dissolved silver salts induce a decrease in the mobility of polymeric chains, and this reduced mobility hinders the gas permeance [29]. The reduced mobility is due to the role of silver ion as a cross-linking point, which was confirmed by the increasing glass transition temperature for other silver-coordinated system [29].
2.2 Nanoparticles and their patterns [13]
Dendrimer has been used as a template for the nanoparticle formation and/or a protective agent for the agglomeration of metal particles [30–34]. Many metal nanoparticles were prepared in the presence of dendrimers by photoreduction and by the addition of chemical reductants. Metal nanoparticles chemically bound on the surface of polymer films in the presence of dendrimers were prepared by the simple modification of dendritic thin films preparation. PAMAM dendrimer was used as a stabilizer for the formation of nanoparticles as well as an anchor to attach the dendrimer/metal nanoparticles to the surface of polymer substrates. Polymer substrates such as PDMS and poly(ethyleneterephthalate) (PET) films were plasma-treated in the presence of MAH in order to introduce reactive succinic anhydride groups on the surface of polymer substrate [12, 15, 26–27]. By preloading dendrimers coordinated with suitable metal ions and then reducing them into metal nanoparticles by the UV exposure, dendrimer/metal nanoparticles attached to the polymer films were obtained (Fig. 3). The metal particles were effectively formed by photoreduction. The absorbance spectra of AgBF4-dendrimer (G3, PAMAM), and HAuCl4-dendrimer solutions in aqueous solution after UV irradiation are shown in Fig. 4a and b, respectively. The absorption band at 410 and 520 nm are plasmon bands of silver and gold nanoparticles, as shown in Fig. 4c(1), and 4c(2), respectively. Gold nanoparticles were attached on the surface of polymer (Fig. 5). The plasmon peak is also observed after the dendrimer metal nanoparticles are attached on PET surface. Fig. 5c showed the similar range of plasmon band at 520 nm, meaning that the nanoparticles attached on the PET films have similar size of those obtained in solution. The contact angles of water of pristine PET and PDMS films were ca 71° and 104°, respectively. They decreased to the range of 30–40° with the loading amount of gold/dendrimer particles, meaning that the surface became hydrophilic, which is analogue to the results in dendrimer loaded on the polymeric films (vide ante). Gold/dendrimer particles were maintained even after sonication in deionized water for 30 min, demonstrating the strong adherence of gold particles on the polymer substrate surface. Metal nanoparticles in the presence of dendrimer are of particular interest for their potential applications such as catalyst, since the metal/dendrimer particle immobilized on polymer substrates have an advantage of preventing aggregation.
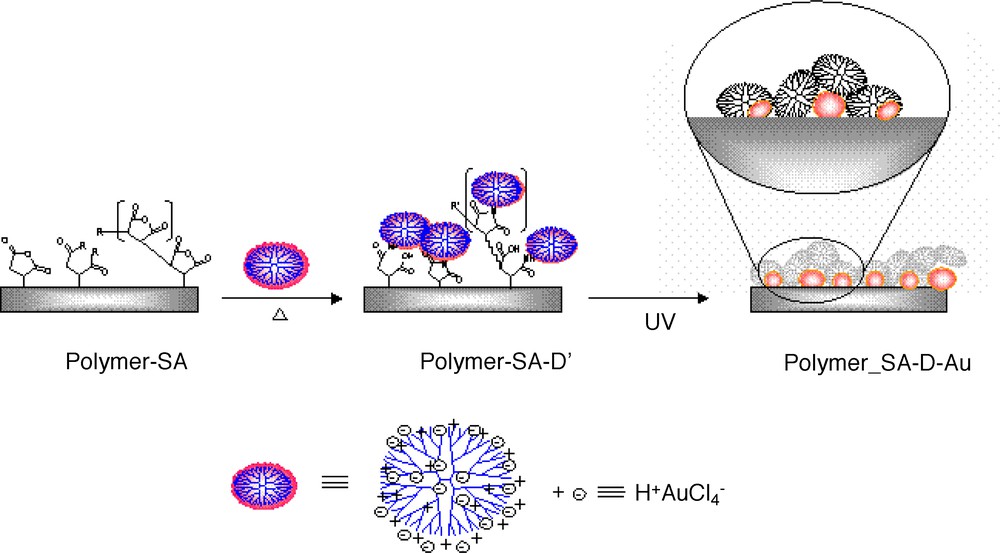
Scheme of the gold nanoparticles attached on polymer film.

TEM micrographs of (a) Ag and (b) Au particles in the presence of G3 dendrimers after UV irradiation. (c) UV–vis spectra of aqueous solutions of (1) AgBF4 dendrimer (G3) and (2) HAuCl4 dendrimer after UV irradiation.
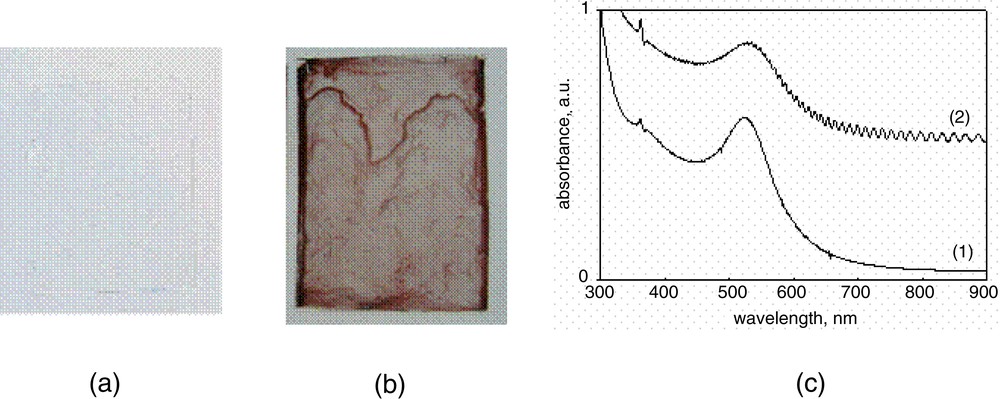
(a) Pristine PDMS film; (b) gold/dendrimer nanoparticles attached on PDMS film; (c) UV–vis spectra of (1) the sample of Fig. 4b and (2) the sample of Fig. 5b.
A gold nanoparticle pattern attached to polymer substrates formed by UV irradiation with a photomask (Fig. 6). This patterning technology can be useful in developing new imaging or nanowire materials.
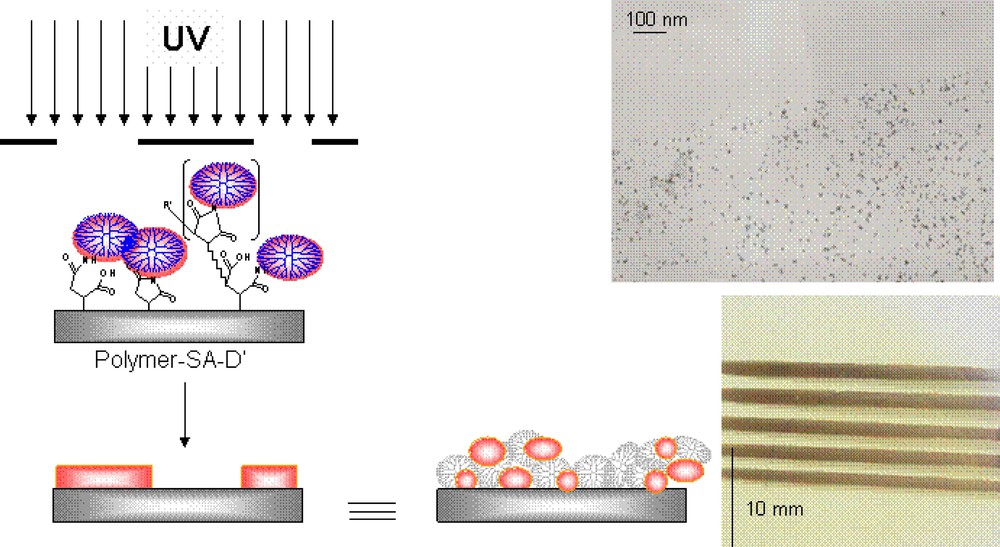
Gold/dendrimer image pattern on a PET substrate obtained by UV exposure through a photomask and TEM micrograph of the surface.
3 StarPEO on polymer films [15]
Organic thin films of nanometer scale can be prepared by using nanometer sized star shaped polymers, which have well-controlled structures like dendrimers. Star shaped polymers are branched polymers with several arms of approximately equal lengths joined together at one end of each chain to a central core. Star shaped poly(ethylene oxide) (PEO; 60 arms, starPEO) was covalently bound to the substrate surface by using the photoreactive azidophenyl groups. To introduce azidophenyl groups on a PDMS surface, a succinic anhydride group was attached by glow-discharge treatment [26], and subsequently immersed in an aqueous solution containing p-azidoaniline hydrochloride. The azidophenyl groups cause the facile elimination of molecular nitrogen from the azido group upon UV irradiation and the formation of phenyl nitrene [35], which is a highly reactive species in a triplet state and undergoes the formation of a covalent bond with neighboring atoms. The azido phenyl groups on the surface will react with starPEO to make the starPEO immobilized PDMS by UV irradiation (Fig. 7).

Scheme of the formation of starPEO on the surface of PDMS film.
The surface characterization for the modified films was carried out by ATR FT–IR and tapping mode atomic force microscopy. The thickness of the immobilized starPEO layer was estimated to be ca. 36 nm and the surface roughness was ca. 4.7 nm.
The gas permeation properties of the starPEO-immobilized PDMS membranes were investigated for pure propane and propylene. The permeance of gases was found to decrease by ca. 30% in the starPEO-immobilized PDMS membranes. It is known that PEO with low lattice energy silver salt, such as AgBF4, became a solid polymer electrolyte, i.e., silver salts are dissolved in the polymer through the coordinative interaction, between silver ions, which can be used as a facilitate olefin transport membrane. The ideal separation factors for propylene/propane were increased with the loading amount of silver ions because of the facilitation action of silver ions.
Introduction of photoreactive molecules such as azidophenyl groups on the surface of the polymeric support helps the formation of thin starPEO film from the materials having no reactive functional groups. Thus, this method enables to immobilize any kind of chemical, especially one without any reactive functional groups, and readily to control the amount of immobilization. The discovery that PEO-attached surfaces exhibit low degree of protein adsorption, cell adhesion and bacterial adhesion also makes this immobilization of starPEO on polymeric substrates a promising, useful approach for producing biocompatible and/or antibiotic materials.
4 PEG brushes on polymer films [14]
Polyethyleneglycol (PEG) was immobilized on a surface of a polymer (polysulfone, PSf) membrane by a photoreactive modification method to make PEG brushes (Fig. 8). PEG was photochemically activated by the reaction of a chain-terminal amine group of PEG with a carboxyl group of 4-azidobenzoic acid in a buffer solution. The azidophenyl-derivatized PEG can be photochemically immobilized on the membrane surface by UV irradiation to make PEG brush membranes due to the high reactivity of azidophenyl groups. The branched PEG brush would be also possibly obtained because the phenyl nitrene group at a terminal end can react with not only the membrane surface but also adjacent other PEG backbone. The formation of a branched polymer brush is a general trend preparing polymer brushes by azidophenyl group at a chain terminus [36].
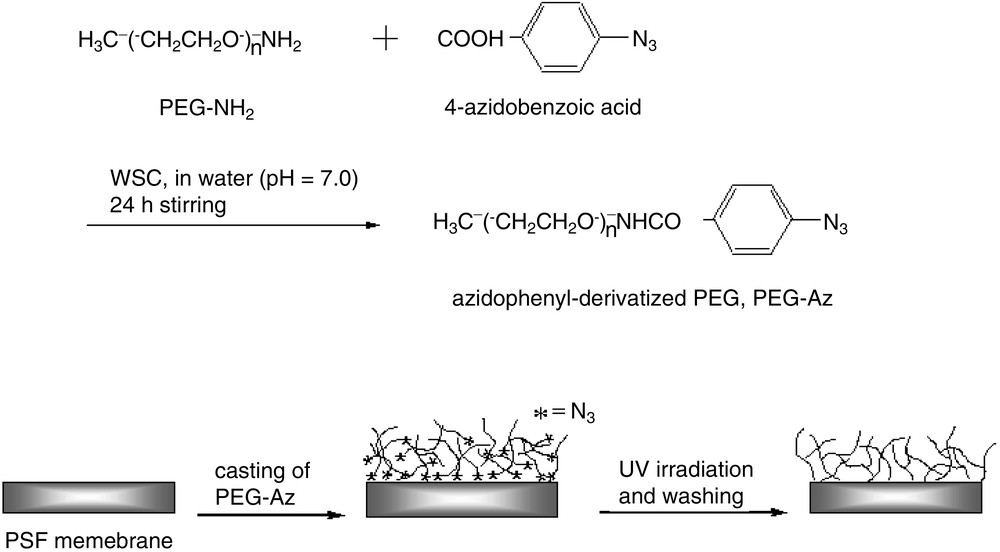
Synthesis scheme of photoreactive PEG and the surface immobilization scheme of azidophenyl-derivatized PEG on PSf membrane.
The estimated thickness of the PEG brush was ca. 20.8 nm by gravimetric change of membrane before and after immobilization. The immobilization of PEG on the PSf microporous membrane caused ca. 30 % decrease in gas permeance of propylene and propane compared with that of a pristine PSf membrane, which is a general trend for the case of the surface-modified membranes. For the PEG-immobilized brush membranes, both propylene and propane permeances decrease, to some extent, with the loading amount of AgBF4. The selectivity of propylene/propane increased with increasing loading amount of silver salt. The high selectivity came from the facilitation action of the propylene carrier, that is, silver complexes in thin PEG matrix.
5 Conclusions
Organic thin films of nanometer scale thickness on the polymeric support have been prepared by using the well-controlled materials such as dendrimers, star shaped macromolecules. Organic thin films become macromolecular electrolytes upon incorporation of silver salts, which were characterized by propylene and propane permeances. The former is high because of the carrier action of silver ions where the latter is low, resulting on high gas selective properties. The metal ions in macromolecular electrolytes were readily reduced to form metal nanoparticles adhered on a polymer support by UV irradiation and a metal nanoparticle pattern with a photomask. It is also possible to control the size of metal nanoparticles on the surface of the polymeric films by dendrimer. Thin organic films on polymeric support can provide a route to entirely new application.
Acknowledgements
This research was supported by the Ministry of Science and Technology of Korea through the ‘Center for Nanostructured Materials Technology’ under ‘21st-Century Frontier R&D Programs’ (JW and JJ) and the ‘Creative Research Initiative Program’ (YSK).