1 Introduction
Nowadays, we are confronted to a number of environmental problems such as pollution and decrease in natural resources. As it is, petroleum reserves will be exhausted within half a century. Moreover, domestic waste such as plastic packing materials are increasing day after day. The synthesis of new biodegradable cellulose-based plastic films may constitute a solution to both problems. Cellulose is the most abundant natural polymer on Earth and, as the main constituent of plants [1], is readily renewable. Cellulose is a homopolymer of β-D-glucose with various degrees of polymerisation, depending on its origin. A large proportion of the numerous hydroxyl groups are engaged in intra and extramolecular hydrogen bonds. In addition, some hydroxyl groups are hydrogen bonded to water molecules naturally trapped in cellulose structure. Cellulose is a fibrillary structure displaying both crystalline and amorphous domains (Fig. 1 ). Cellulose chemical modifications occur more easily in amorphous regions than in crystalline ones [2]. Homogeneous derivatization needs either activation [3] or solubilization [4–15] of the polymer before its modification [16,17].
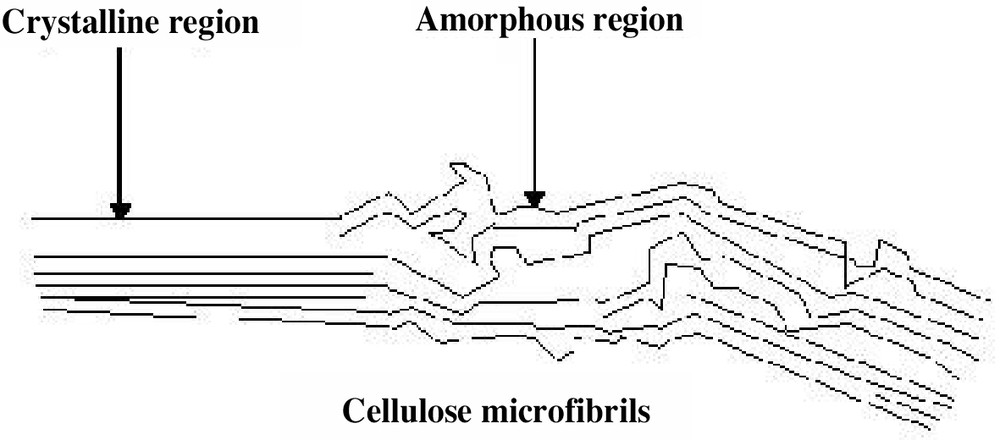
Cellulose microfibril morphology.
We are particularly interested in the synthesis of cellulose fatty esters that are potential biodegradable plastics. Acylation of cellulose with short-chain carboxylic acids is well documented [18,19], but esterification with fatty acid derivatives has been less studied because of the poor reactivity of these products. The first attempts to heterogeneous acylation have been carried out by classical activation [20–22], or, more recently, in our laboratory, by microwave irradiation [23]. Fatty acid esters of cellulose have been synthesized in homogeneous media such as LiCl/DMAc with classical heating [24,25], but achievement of these reactions needs long reaction times.
In the present work, we synthesized cellulose esters in homogeneous conditions by using microwave irradiation to decrease the reaction time from several hours [25] to one minute. In addition, we studied the effect of acylating agents and catalyst amounts on the degree of substitution (DS). We also studied the influence of purification method, the mechanical and thermal properties and the biodegradability of the synthesized cellulose esters.
2 Experimental
2.1 Material
Except LiCl, kept in vacuo, all reagents were stored at room temperature, without special precautions: sodium hydroxide 98% (Carlo Erba Reagenti); 0.1 M Normadose™ hydrochloric acid, anhydrous lithium chloride 99% (Prolabo); pure microcrystalline cellulose ≈ 20 μm (Aldrich); N,N-dimethyl-4-aminopyridine (DMAP) 99%, lauroyl chloride 98% (Acros). All solvents were used without further purification: chloroform 99.9% (solvents, documentation, syntheses); methanol 99.99% (Fisher); N,N-dimethylacetamide (DMAc) 99% (Aldrich); absolute ethanol (Prolabo). Deuterated chloroform 99.8% containing 0.03% TMS (Eurisotop) was stored at 4 °C. The microwave reactor was a Synthewave 402® P = 300 W (Prolabo).
2.2 Spectroscopic measurements
NMR spectra were performed with a DPX-400 MHz Bruker apparatus. IR spectra were performed with a Spectrum 1000 FT–IR spectrometer.
2.3 Cellulose solubilization
We have used a method modified from Sjöholm et al. [14] in order to perform cellulose solubilization: LiCl (80 g) was dissolved into 1 l DMAc at 50 °C (1 h). 20 g cellulose were submerged with bidistilled water (150 ml) and preserved at 4 °C during 1 h. Then it was filtered and washed three times with methanol during 30 min followed by three washings with DMAc during 30 min. After this, cellulose was added to the LiCl/DMAc solution. The medium was heated at 50 °C and stirred for 12 h.
2.4 Cellulose acylation
DMAP (0.7 to 5.3 g; 0.6 to 4.3 mmol; 0.1 to 0.7 equiv.) and lauroyl chloride (2.8 to 20 ml; 12 to 87 mmol; 2 to 14 equiv.) was added to 50 ml of cellulose solution (1 g; 6.2 mmol) and the medium was activated in the microwave reactor, the reaction time varying from 1 to 2 min and the power output varying from 120 to 300 W. The final temperature was between 140 and 165 °C. The product was precipitated by addition of water (100 ml), followed by methanol (250 ml) and filtered. Two methods were used to purify the solid. It was purified by a repeated solubilization–precipitation process using the chloroform/methanol system (precipitation in water and methanol, filtration, dissolution in a minimum of chloroform, precipitation with methanol, filtration, dissolution in a minimum of chloroform, precipitation with methanol, filtration), or by Soxhlet extraction with methanol (precipitation in water and methanol, filtration, 8 h Soxhlet extraction with methanol). IR (KBr): 3345 cm–1 (hydroxyls), 2893 cm–1 (alkyl chains), 1741 cm–1 (carbonyls).
2.5 Ester characterization
2.5.1 Casting
Cellulose esters (≈ 2.5 g) were dissolved in 30 ml chloroform and then the solvent was evaporated at air.
2.5.2 DS (degree of substitution) determination
Volumetric method [22]. Esters were saponified with 0.25 M NaOH alcoholic solution for 16 h at 40 °C. The resulting solution was titrated with 0.1 M hydrochloric acid. Two equivalent volumes were noticed on the resulting curve, the first (V1) corresponding to the neutralization of excess NaOH and the second (V2) corresponding to the neutralization of the sodium laurate.
- nfa = fatty acid amount
- V1 = first equivalent volume
- V2 = second equivalent volume
- Mgu = glucose unit molecular weight = 162.14 g mol–1
- Mfa = fatty acid molecular weight = 200.32 g mol–1
- Mw = water molecular weight = 18.02 g mol–1
- me = ester mass.
NMR method. The spectra were performed on esters dissolved in CDCl3. The different signals can be classified in three parts: carbohydrate protons between 2.75 and 5.50 ppm, methylene protons between 1.20 and 2.32 ppm and methyl protons at 0.89 ppm.
- ICH3 = methyl protons integration
- IC = carbohydrate protons integration.
2.6 Mechanical properties
Uniaxial tensile tests were performed on ten identical samples. The apparatus consisted in two jaws, one fixed and the other with a vertical move of 500 mm min–1.
2.7 Thermal analysis
Thermogravimetric analyses were performed using a Setaram B 85 microbalance (precision ±10 μg). The heating rate was set at 5 °C min–1 until a limit of 300 °C was reached.
Differential thermal analyses were performed with a DTA 1600 Labsys apparatus. The heating rate was set at 5 °C min–1 until a limit of 300 °C was reached.
2.8 Biodegradability
For each value of DS, a sample of plastic film of known mass was transferred into a flask containing 10 ml of phosphate buffer. The medium was inoculated with Chromobacterium violaceum, a Gram negative strain isolated in a previous work [22]. The flasks were incubated at room temperature on a rotary shaker (150 rpm) for 6 months. After centrifugation and desiccation, the residual solid was weighted and the loss of weight converted into a biodegradation ratio. No weight loss was measured in a control flask containing only sterile water and an identical amount of material.
Bacterial screening: esters were submerged in 20 different media composed of various sampling from the environment (Table 1) mixed with water and supplements ((NH4)2SO4, K2HPO4, KH2PO4, trisodium citrate, MnCl2 and B1 vitamin). These tests were kept outdoor for one year. Three out of the 20 plastic samples have been modified, displaying increased flexibility. The bacteria present in the corresponding media were isolated and plastics were incubated with them during eight months at room temperature.
Bacterial screening; origin of samples
Entry | Medium | Modification of mechanical behaviour |
1 | hen house soil | + |
2 | compost 1 | – |
3 | decomposed wood | – |
4 | garden soil 1 | – |
5 | dry leave pile | + |
6 | dead leaf 1 | – |
7 | pond water | – |
8 | wet leave pile | – |
9 | leave pile | – |
10 | garden soil 2 | – |
11 | track soil | – |
12 | forest soil 1 | – |
13 | compost 2 | – |
14 | dead leaves 2 | – |
15 | bark | – |
16 | rivulet water | – |
17 | compost 3 | + |
18 | dead fern | – |
19 | forest soil 2 | – |
20 | garden soil 3 | – |
3 Results and discussion
3.1 Synthesis
We synthesized fatty esters of cellulose with various DS (degree of substitution). For this purpose, we used the LiCl/DMAc system as a solvent, lauroyl chloride as an acylating agent, DMAP (N,N-dimethyl-4-aminopyridine) as a catalyst and microwaves as an activator (Fig. 2 ).

Cellulose acylation.
Two methods were used for cellulose solubilization. The first method was an adaptation of Regiani et al.’s [2] method, using microwave irradiation instead of conventional heating. Cellulose and LiCl were kept one night in a desiccator and DMAc (N,N-dimethylacetamide) was added. Then, the mixture was activated by microwave irradiation between 6 and 19 h (T = 120 °C). The second method was modified from Sjöholm et al. [14]. Cellulose was washed with bidistilled water (1 h), with methanol (3 × 30 min) and with DMAc (3 × 30 min). After this, cellulose was added to a 8% (w/v) solution of LiCl in DMAc and stirred overnight at 50 °C.
Then, the dissolved cellulose was esterified with lauroyl chloride. We used a defined medium to optimise microwave irradiation: 1 g of cellulose dissolved in 50 ml of LiCl/DMAc (8% (w/v)), 18 ml (13 equiv.) lauroyl chloride and 0.9 g (1.2 equiv.) DMAP. Reaction times (t) and power outputs (P) were varied from 120 W for 60 s to 300 W for 120 s. For each value of these two parameters, no change in DS was observed (all DS measured were about 2.7). In conclusion, the DS is independent on the conditions used. Weight increase (WI) was determined for each experiment on the purified ester by using the formula: (me: final ester mass, mc: initial cellulose mass) (Table 2). A maximum was reached for 60 s at 300 W. Increasing reaction time beyond one minute resulted in temperature increase and cellulose degradation, as attested by a brown colour and a drop in WI (Table 2); moreover, the plastic films then produced are more brittle than those produced at lower temperature. For these reasons, in the following study we chose to irradiate the reaction medium for 60 s at 300 W.
Microwave activation optimisation
Entry | t (s) | P (W) | WI (%) |
1 | 60 | 120 | –2 |
2 | 60 | 180 | 124 |
3 | 60 | 240 | 129 |
4 | 60 | 300 | 149 |
5 | 70 | 300 | 125 |
6 | 80 | 300 | 130 |
7 | 90 | 300 | 132 |
8 | 120 | 300 | 92 |
Furthermore, we analysed the influence of lauroyl chloride and DMAP amounts by using these reaction conditions: 1 g of cellulose solubilized in 50 ml LiCl/DMAc 8% (w/v), lauroyl chloride amounts between 5 and 14 equiv per glucose units and DMAP amounts between 0.05 and 0.5 equiv per mol lauroyl chloride. The catalyst amount did not influence the DS, but the molar yield (mole number of esterified glucose units/mole number of initial glucose units × 100) was dependent on DMAP, especially for the lowest lauroyl chloride amounts (Table 3). When 0.5 equiv DMAP were used, we could observe a sharp yield increase and WI between 2 and 5 equiv of lauroyl chloride and a stabilization at 80% and 180% respectively (Figs. 3 and 4 , grey curves). Due to a low esterification level of cellulose for lower lauroyl chloride quantities, the product could not precipitate with methanol, leading to negative WI. Fig. 5 (grey curve) shows that DS weakly raised when 2 to 10 equiv of lauroyl chloride were used and stabilized close to 2.5. A conventional microwave oven gave appreciatively the same results. [26]
DMAP amount effect on cellulose acylation
Entry | DMAP amount (equiv.)a | Lauroyl chloride amount (equiv)b | Yield (%) | WI (%) | DSc |
1 | 0.05 | 5 | 51 | 50 | 1.8 |
2 | 0.1 | 41 | 20 | 1.7 | |
3 | 0.3 | 67 | 109 | 1.9 | |
4 | 0.5 | 82 | 148 | 1.8 | |
5 | 0.05 | 8.1 | 63 | 119 | 2.2 |
6 | 0.3 | 70 | 141 | 2.2 | |
7 | 0.5 | 88 | 194 | 2.1 | |
8 | 0.05 | 10.2 | 50 | 69 | 2.1 |
9 | 0.1 | 62 | 123 | 2.3 | |
10 | 0.3 | 73 | 157 | 2.2 | |
11 | 0.5 | 82 | 180 | 2.2 | |
12 | 0.05 | 14 | 74 | 160 | 2.2 |
13 | 0.1 | 69 | 164 | 2.5 | |
14 | 0.3 | 80 | 177 | 2.2 | |
15 | 0.5 | 72 | 173 | 2.6 |
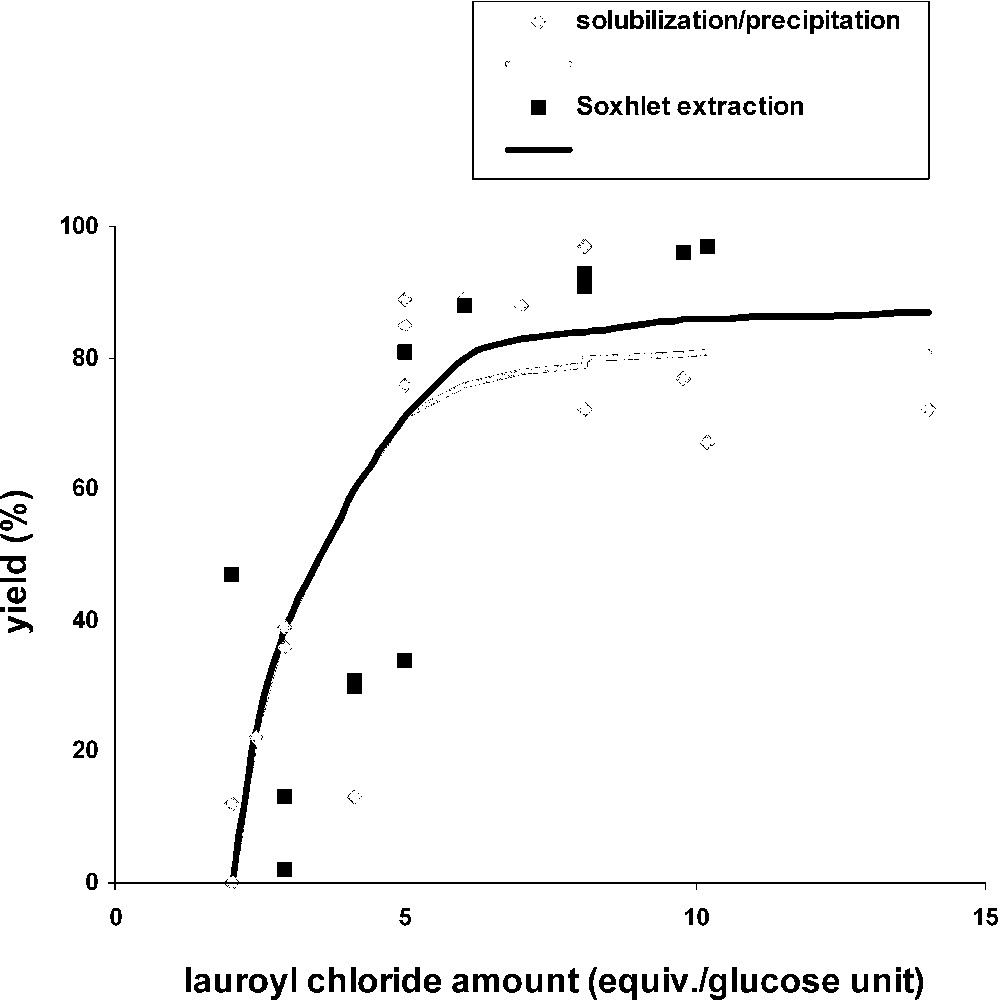
Yield versus lauroyl chloride amount (cellulose solution [1 g], DMAP [0.5 equiv/lauroyl chloride], microwave irradiation [1 min, 300 W]), comparison between the two purification methods.
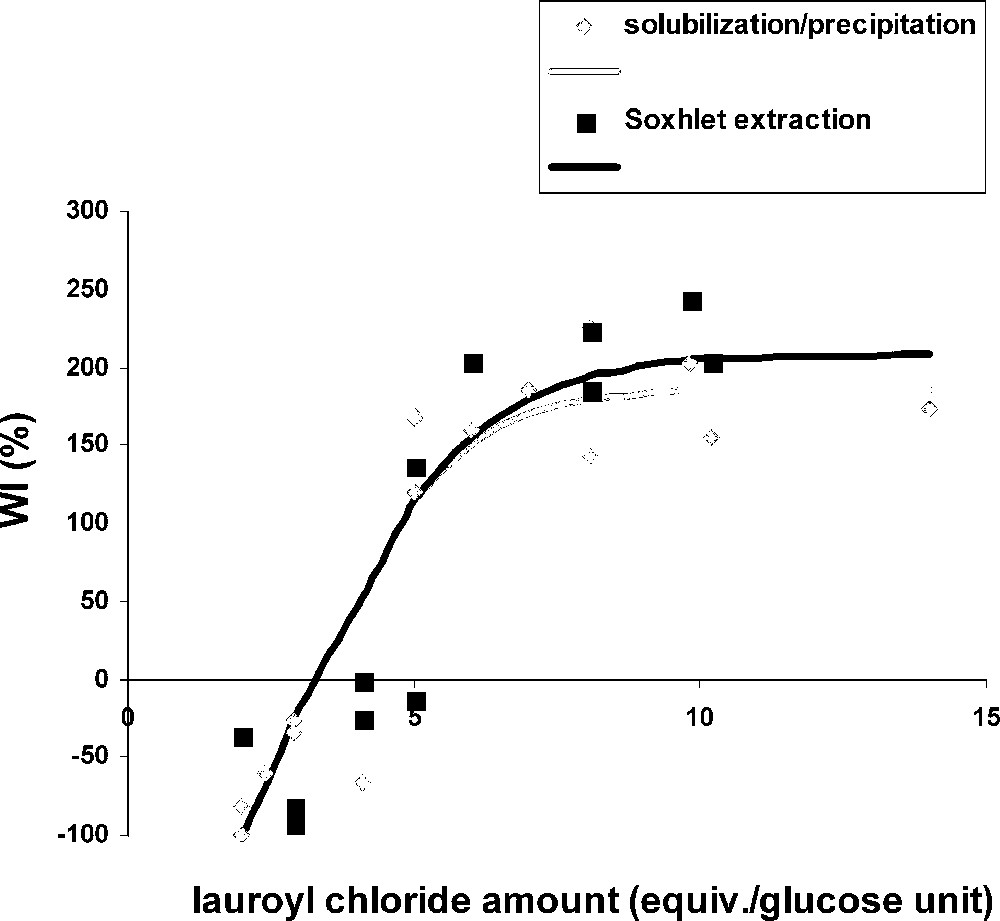
WI versus lauroyl chloride amount, comparison between the two purification methods (cellulose solution [1 g], DMAP [0.5 equiv/lauroyl chloride], microwave irradiation [1 min, 300 W]).

DS versus lauroyl chloride amount, comparison between the two purification methods (cellulose solution [1 g], DMAP [0.5 equiv/lauroyl chloride], microwave irradiation [1 min, 300 W]).
This study was achieved with esters purified by the dissolution–precipitation method. We compared these esters with esters obtained with another purification method.
Figs. 3–5 compare results obtained by the two purification methods. There was no significant difference between these two series, with respect to yield, WI and DS. We can conclude from these results that the solubilization/precipitation method is as efficient as the Soxhlet extraction for cellulose fatty esters purification, and also, easier and faster to use.
3.2 Thermal and mechanical properties
We analysed and compared the mechanical properties of three different plastic films with DS = 1.7, 1.9 and 2.4. These esters were prepared with homogeneous solution of cellulose (8 g) by microwave irradiation (1 min, 300 W) with 3, 4 and 8 equiv of lauroyl chloride respectively and with 0.5 equiv of DMAP per lauroyl chloride, the purification was performed by the dissolution–precipitation method. Each result was the average of ten assays. The tested samples consisted of 8 × 2 cm strips whose thicknesses were comprised between 150 and 200 μm. The distance between the two jaws was about 3 mm, and speed of the upper bit was 500 mm min–1. The elastic modulus E (Young Modulus) decreases from 19 to 9 MPa with increasing DS (Fig. 6 ), meaning that plastics are more deformable when cellulose is strongly substituted. The failure strain level, with bearing on ductility rises with DS from 90 to 360% (Fig. 7 ). On the other hand, plastic resistance is maximum for DS = 1.9 (tensile failure stress: σr = 18 MPa) (Fig. 6). In comparison with commercial polyethylene (PE) packaging products, Young modulus and failure strain level are much larger (E ≈ 300 MPa and εr ≈ 1100%) for PE than cellulose esters. So cellulose esters are more deformable and less ductile. Tensile failure stresses are close to PE, so the cellulose ester films are as resistant as polyethylene.

Young modulus and tensile failure stress versus DS (vertical move of 500 mm min–1).

Failure strain level versus DS (vertical move of 500 mm min–1).
We also analysed the thermal properties of the same three cellulose esters (DS = 1.7, 1.9 and 2.4). Thermogravimetric analyses were performed on about 12 mg of cellulose esters. Degradation temperature increases with DS from 150 °C (DS = 1.7 and 1.9) to 160 °C (DS = 2.4). Glass transition temperatures were determined by differential thermal analysis. They lie between 100 and 180 °C and increase when DS drops (Table 4).
Plastic film thermal analysis
DS | Td | Tg |
1.7 | 150 | 180 |
1.9 | 150 | 170 |
2.4 | 160 | 100 |
3.3 Biodegradability
Biodegradation studies revealed that plastic films with DS > 1 underwent very modest weight losses. On the other hand, a loss of 15% in weight was observed for DS = 0.7. This biodegradation rate is somewhat higher than those reported by Glasser et al. [27]. According to these authors, the biodegradability of cellulose lauric esters with a DS = 0.3 was 5%. The difference with our results lies undoubtedly in the fact that we used a microorganism, whereas Glasser et al. [27] used a purified cellulolytic enzyme. The microorganism we used undoubtedly produced a set of enzymes working in synergy. It is noteworthy that a significant modification in mechanical behaviour of the residual solid was observed, the material increased in brittleness.
To obtain better biodegradation results on high DS cellulose esters, we proceeded to a bacterial screening. It consisted in taking, from the environment, bacteria that could degrade the material. Twenty cellulose ester samples (DS = 2.3) were submerged in 20 different media (Table 1) and left outdoor during one year. Three samples showed visible mechanical behaviour modifications (films became more flexible). Then, two bacterial strains were isolated from these three media; we submerged the three plastics (1, 5, 17) in distilled water inoculated by the corresponding bacterium. Eight months later, live bacteria were found in the media and the mechanical behaviour of the corresponding films was modified (Table 5). The films were washed, dried and weighted. In spite of their apparent partial degradation, no loss in weight was observed because bacteria were clung to the plastic film. This preliminary study on cellulose esters biodegradability is very encouraging.
Film mechanical behaviour after an eight-month incubation with bacteria
Samples | Mechanical behaviour before degradation | Mechanical behaviour after eight month |
1 | Resistant, elastic | Flexible, brittle |
5 | Flexible, not brittle | |
17 | Gelatinous, very slack, brittle |
4 Conclusion
We synthesized cellulose esters with a large range of DS by perfecting and optimising a new, rapid and reproducible method using solubilization in LiCl/DMAc solvent and microwave irradiation with controlled power. Reaction time was reduced from several hours to one minute. Thermal and mechanical properties and biodegradability of plastics were analysed. On this last point, encouraging results were obtained. The plastic with DS = 1.9 showed the best mechanical properties E = 16 MPa, εf = 320% and σf = 18 MPa.
Acknowledgements
We thank the ‘Conseil régional du Limousin’ for financial support. We also thank Dr R. Zerrouki for helpful discussion, Dr M. Guilloton for help in manuscript, Dr C. Petit and J. Rozier for mechanical analyses, Drs. E. Champion and C. Penaud for thermal analyses.