1 Introduction
Friedel–Crafts acylation of aromatic substrates is widely employed for the synthesis of aryl ketones, intermediates of relevant industrial importance for the production of drugs, fragrances, and pesticides. The acylating acids are acyl halides, and homogeneous, Lewis-type acids (typically, metal halides) are the catalysts employed [1]. The latter are however used in large amounts, often more than the stoichiometric requirement; also, recovery of the metal halides at the end of the reaction is not possible, and huge amounts of waste streams are co-generated. Therefore, the environmental impact of these processes makes highly desirable the development of new technologies that employ heterogeneous, recyclable catalysts, and of reactants that generate more environmentally friendly co-products [2].
With this purpose, several different types of solid acid catalysts have been investigated for the acylation of aromatics, but best performances have been obtained with medium-pore and large-pore zeolites [3–13]. Specifically, H-ZSM5 is active and selective in ring acylation of low-molecular-weight aromatics (phenol and toluene), while H-Beta and H-Y zeolites are claimed as optimal catalysts for the acylation of anisole, xylenes and 2-methoxynaphthalene. A significant improvement in this field is the process developed by Rhodia, in which anisole and veratrole react with acetic anhydride, yielding the corresponding ketones with high yield [13–16]. A high specificity to the para isomer is observed, due to reaction mechanism rather than to an effect of shape-selectivity. In general, however, the use of acylating agents other then halides, e.g., anhydrides or acids, is limited to the transformation of aromatic substrates highly activated towards electrophilic substitutions.
Heterogeneous catalysts that have been less investigated for the acylation of aromatic substrates are acid-treated montmorillonite-type clays [17]. These clays are low-cost, widely available materials, and synthetic organic chemists have largely used them in a variety of acid-catalysed reactions [18–21]. The aim of the present work was to study the catalytic performance of montmorillonite acid clays in the reaction of 1,3-dihydroxybenzene (resorcinol) with benzoic acid. The latter is the optimal acylating agent from an environmental point of view, since the benzoylation only co-produces water. Benzoic acid is less reactive than anhydrides; in the present case, however, the high charge density of the aromatic ring in resorcinol makes benzoic acid the best candidate for the electrophilic reaction. The desired product of the reaction is 2,4-dihydroxybenzophenone (2,4-DHB), which is an intermediate for the production of 4-O-octyl-2-hydroxybenzophenone, a UV-light absorbent for polymers. 2,4-DHB is typically synthesized by reaction between resorcinol and benzyltrichloride, with co-production of HCl and of spent metal chlorides.
2 Experimental
Montmorillonite samples belonging to the K series were supplied by Süd-Chemie; FULCAT 22B was formerly supplied by Laporte (now by Rockwood Appl. Ltd). Dehydration treatments were carried out equilibrating clays K5 and K30 at 100, 200 and 400 °C in air; samples were finally cooled in a controlled atmosphere to avoid water re-adsorption.
Catalysts were characterized by means of X-ray powder diffraction (Philips PW 1050/80). Surface areas and pore volumes were measured by N2 isotherm adsorption at liquid N2 temperature using Sorptomatic 1900 Carlo Erba. Sample acidity was evaluated by pyridine adsorption. A thin self-supported wafer of clay was activated at 550 °C in a dynamic vacuum (residual pressure < 10–4 mbar) for 2 h; then IR spectrum was recorded (PerkinElmer FTIR 1750). Adsorption was done contacting the sample with 5 torr of pyridine for 5 min at room temperature. Then the sample was heated at 200 and at 400 °C under dynamic vacuum condition, and at each temperature the IR spectrum was recorded.
The catalytic tests were carried out in a batch-wise three-neck flask, with mechanic stirring and internal thermocouple. The reaction mixture contained 0.1 mol of resorcinol, 0.1 mol of benzoic acid and 3.5 g of catalyst. The reaction temperature was 145 °C. Products and unconverted reactants have been analysed by gas chromatography (Carlo Erba GC 6000 Vega Series 2 instrument), equipped with a FID detector and a non-polar column HP-5.
3 Results and discussion
3.1 Characterization of samples
Table 1 summarizes the main characteristics of samples used for catalytic tests. The samples belonging to the K series of montmorillonite-type acid clays (K5, K10, K20, K30), possess an increasing silica-to-alumina ratio, and a corresponding decreasing amount of iron oxide, of alkali/alkaline earth metal oxides, as a consequence of the dealumination (acid treatment) procedure. The latter also causes the increase in surface area; the pore distribution for K5 and K30 is illustrated in Fig. 1 . It is shown that the dealumination treatment modifies the pore size distribution; the mesopores distribution in the K30 sample is shifted towards the macropore region, while in the K5 sample the majority of pores falls in the 20–60-Å pore radius range. Sample FULCAT 22B possesses a composition that is intermediate between that of K5 and of K10 samples.
Main characteristics of commercial samples used for catalytic tests
Sample | SiO2 (wt %) | Al2O3 (wt %) | SiO2/Al2O3(wt ratio) | Fe2O3 (wt %) | CaO + MgO + K2O + Na2O, (wt %) | Loss on ignition (wt %) | Moisture content (wt %) | Surface area, (m2 g–1) |
FULCAT 22B | 61.2 | 13.9 | 4.40 | 5.9 | 5.4 | 7.0 | 6.0 | 180 |
K5 | 65.0 | 19.0 | 3.42 | 4.8 | 4.4 | 6.5 | 200 | |
K10 | 73.0 | 14.0 | 5.21 | 2.7 | 3.8 | 6.0 | 240 | |
K20 | 75.0 | 12.5 | 6.00 | 2.4 | 3.3 | 6.3 | 240 | |
K30 | 80.0 | 10.0 | 8.00 | 1.8 | 2.0 | 6.0 | 330 |
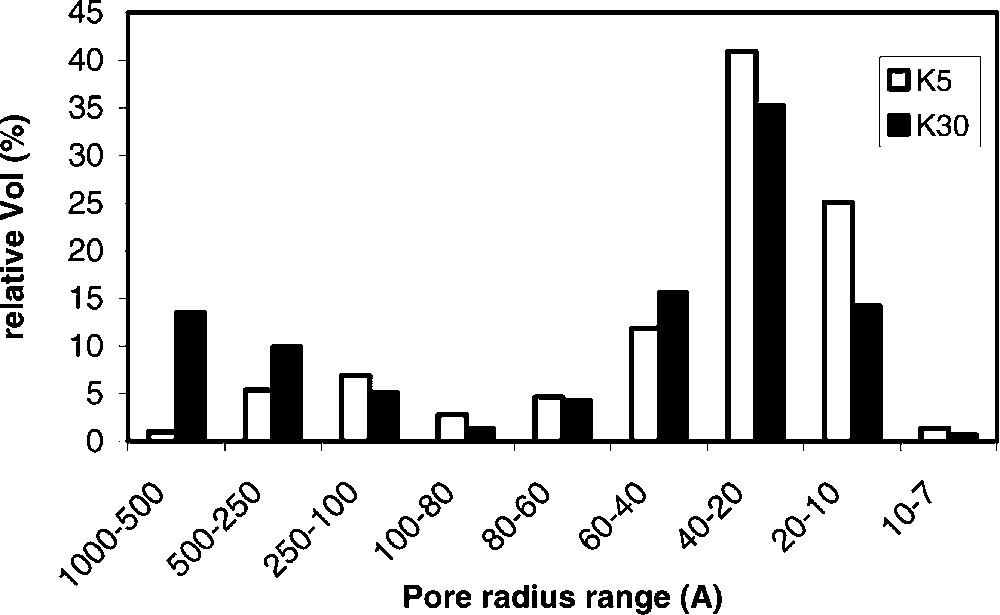
Relative distribution of pore size in K5 and K30 samples.
The XRD patterns of samples belonging to the K series are shown in Fig. 2 . Patterns are those of silico-aluminates (e.g., montmorillonite IPDF 02-0037, silica IPDF 33-1161, and tosudite IPDF 22-0956); additional reflections are observed in K5, attributable to Ca/Mg/Fe/Si mixed oxide (IPDF 13-0421), and to kaolinite Al2Si2O5(OH)4 (IPDF 06-0221). These reflections disappear in dealuminated samples.
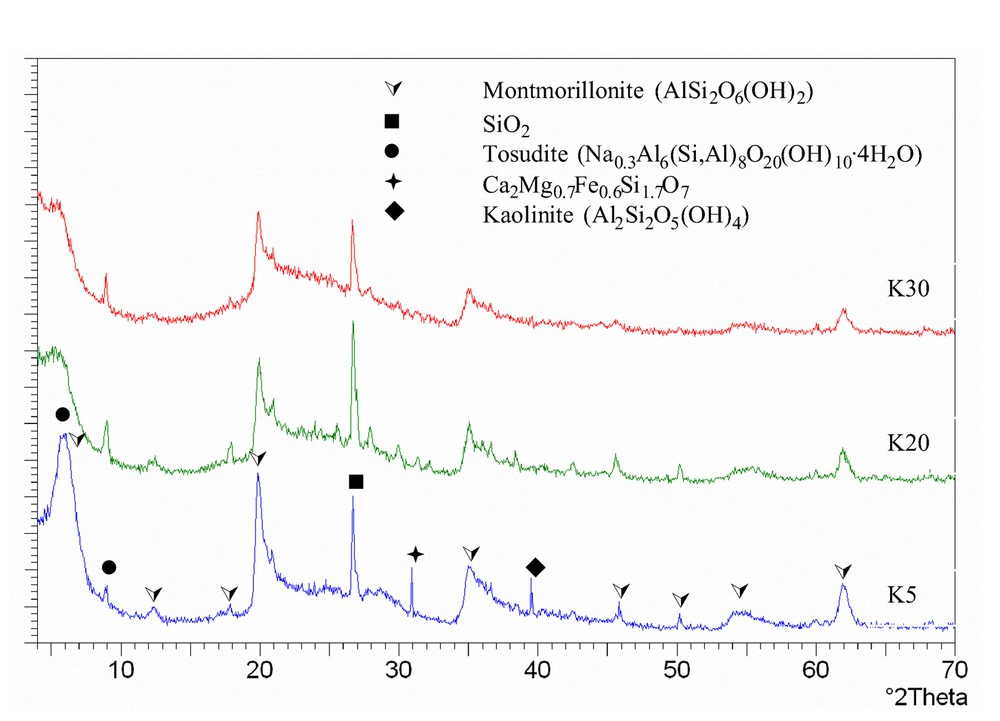
X-ray diffraction patterns of K samples.
The acidity of samples K5 and K30 was checked by means of pyridine adsorption. Fig. 3 plots the FT–IR spectra of K5 and K30 samples, after evacuation and adsorption of pyridine at room temperature, followed by heating at 200 and at 400 °C. It is shown that, after evacuation at 200 °C, sample K5 possesses a higher number of Brønsted sites than sample K30, as inferred from the higher intensity of the IR band positioned at 1545 cm–1. After evacuation at 400 °C, the residual amount of Brønsted sites is very low, while Lewis sites are still present, as inferred from the band falling at 1455 cm–1. The intensity of the latter band is comparable in the two samples. Flessner et al. [22] found that the overall number of surface acid sites (Brønsted + Lewis), as determined by ammonia adsorption, decreases on increasing the Si/Al ratio. The ratio between the number of surface silanols at the external layer, and of Al2OH groups in the interlayer region also was found to follow the Si/Al ratio. Spectroscopic measurements evidenced that the highest number of Brønsted acid sites was in samples having the lowest alumina content (K30); this led to the conclusion that medium-weak Brønsted sites are associated to external silanols in the tetrahedral clay layer. The acid strength of these sites was not much affected by the Si/Al ratio. Lewis acid sites, relatively strong, were instead associated to low-coordination Al3+ cations [22]. The authors proposed that the progressive dealumination of the K samples, from the K5 to the K30 sample, degraded the octahedral clay layers, with a reduction of the interlayer charge and thus making internal protonic acid sites, associated to silanols, available for interaction with organic molecules. Disagreement with data reported by Flessner et al. [22] concerns the number of Brønsted sites, which in our case was found to be higher in the sample having the higher Al content (K5). This difference may be due to the fact that the basic probe used in the present work is smaller (pyridine against pivaronitrile); this made it possible better access of the probe into the intralayer spacing of the clays, in which part of hydration water remained entrapped even after thermal treatment and evacuation at high temperature. Water may be responsible for the generation of Brønsted sites.
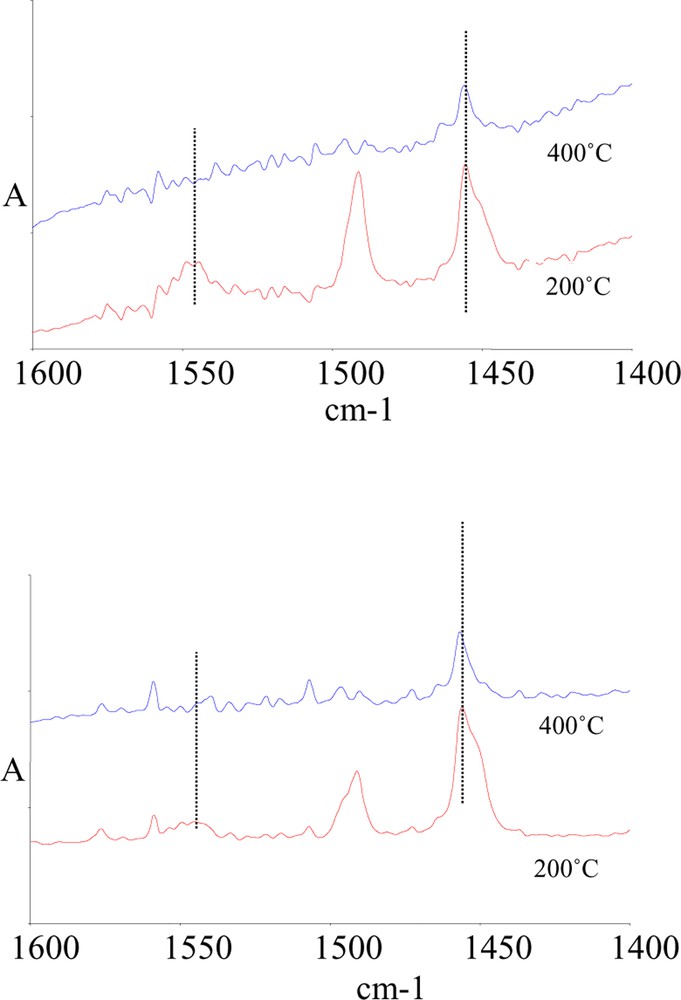
IR spectra of sample K5 (top) and of K30 (bottom) after adsorption of pyridine at room temperature, and evacuation at 200 and at 400 °C.
3.2 The reactivity in resorcinol acylation: the reaction scheme
Tests were made by changing the reaction time, with the K5 and K30 samples; results are summarized in Fig. 4 , which reports the selectivity to the products, resorcinol monobenzoate (RMB) and 2,4-dihydroxybenzophenone (2,4-DHB), as functions of resorcinol conversion; the latter was varied by varying the reaction time and/or the amount of catalyst loaded. Under our conditions, no other by-products were found. It is shown that with both catalysts the only primary product was RMB, the selectivity of which was total for low resorcinol conversion. On increasing the conversion, the selectivity to RMB decreased, with a corresponding increase in the selectivity to 2,4-DHB. Therefore, the latter was exclusively formed by consecutive reaction upon RMB, via Fries rearrangement. This suggests that (i) RMB is not directly transformed, once formed, to 2,4-DHB inside catalyst porosity, since otherwise this would correspond to a direct contribution in the formation of this compound (which instead was not observed), and (ii) RMB first diffuses out of the catalyst pores, and successively is activated and transformed to 2,4-DHB. One possible explanation is that the high hydrophilic features of the clay surface, especially in samples having higher alumina content, makes the pores filled with more polar substances, such as benzoic acid and resorcinol, while less polar compounds rapidly diffuse out of the clay porosity and undergo consecutive transformation at the active sites located at the external particle surface.
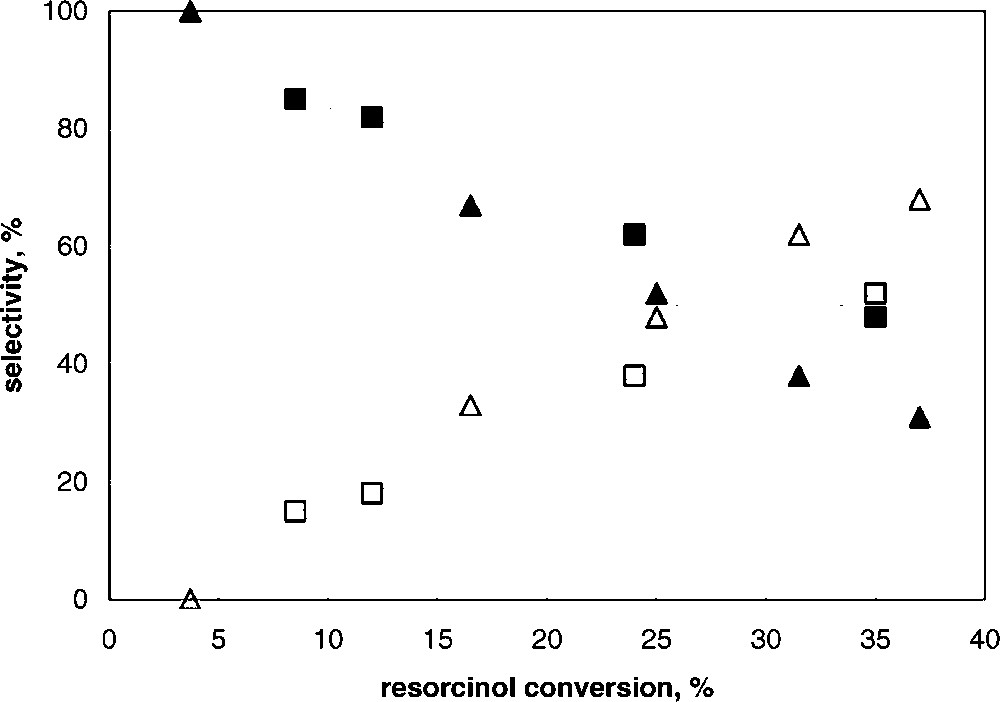
Selectivity to RMB (full symbols) and to 2,4-DHB (open symbols) as functions of resorcinol conversion for K5 (▴) and K30 (■) catalysts.
Tests shown in Fig. 4 were made so as to obtain data far from the possible equilibrium; further data were obtained with the FULCAT 22B sample at 180 °C, reaction time 2 h, and by addition of 50% wt. of mesitylene as the reaction solvent. This also makes possible the continuous removal of the water formed by the O-acylation through the azeotrope mesitylene/water. Results are compared in Table 2 with those obtained (i) by carrying out the reaction in the absence of solvent, (ii) by reacting RMB alone (thus starting the reaction in the absence of resorcinol and benzoic acid), (iii) by reacting together resorcinol, benzoic acid and RMB, (iv) by reacting RMB with an equimolar amount of water. Even for reactions made starting from RMB, data have been expressed with reference to resorcinol (thus, under the hypothesis of loading an equimolar amount of resorcinol and benzoic acid), and considering RMB and 2,4-DHB as the reaction products. This makes possible the comparison of data obtained when starting from RMB with those obtained when starting from resorcinol + benzoic acid. It is shown that the distribution obtained was the same when starting from the different reacting mixture. This suggests that the reaction between resorcinol and benzoic acid to yield RMB is fully reversible, and that the hydrolysis of RMB to yield resorcinol and benzoic acid also occurs in the absence of added water. This is an indication of the role played by water retained in clays.
Results obtained starting from different reaction mixtures. Catalyst FULCAT 22B; reaction temperature: 180 °C. Solvent: mesitylene, 50 wt % of the reaction mixture. Reaction time: 2 h
Reaction mixture (mol) | R conv. (%) | Sel. RMB (%) | Sel. 2,4-DHB (%) |
R (0.1) + BA (0.1), no solvent | 61.3 | 26.0 | 74.0 |
R (0.1) + BA (0.1) | 80.6 | 31.9 | 68.1 |
R (0.06) + BA (0.06) + RMB (0.04) | 77.9 | 30.9 | 69.1 |
*RMB (0.1) | 80.9 | 31.2 | 68.8 |
*RMB (0.1) + W (0.1) | 81 | 35.6 | 64.4 |
* Final concentrations of components (R, RMB, DHB) have been in all cases referred to resorcinol (R), i.e., considering the latter as the starting reactant, and RMB/DHB as the products. In order to do this, the initial concentrations of R and BA have been assumed to be equal to that of RMB effectively loaded in the reactor.
Our data agree with those reported by Hoefnagel and van Bekkum [5], who studied the reaction between resorcinol and benzoic acid catalysed by ion-exchange resins and zeolites. The authors proposed that the main primary reaction that occurs is the esterification to yield the (RMB); evidences for direct ring-acylation were not obtained, but a contribution of this reaction was not excluded. Besides RMB and 2,4-DHB, by-products found were resorcinol dibenzoate (RDB), and 3,6-dioxy-9-phenyl-xanthydrol, a coloured compound obtained by reaction between two moles of resorcinol with one mole of benzoic acid; these compounds were not found in our tests with the K series of catalysts, likely because of the lower conversions achieved. Evidence for the formation of low amounts of these by-products was instead obtained with the FULCAT catalyst, which reached higher resorcinol conversion due to the higher reaction temperature employed (Table 2).
The removal of water from the bulk liquid by azeotropic distillation makes it possible to considerably increase the conversion, which reaches 80.6% against 61.3% in the absence of the solvent, since the esterification reaction is favoured.
Scheme 1 summarizes the main findings.
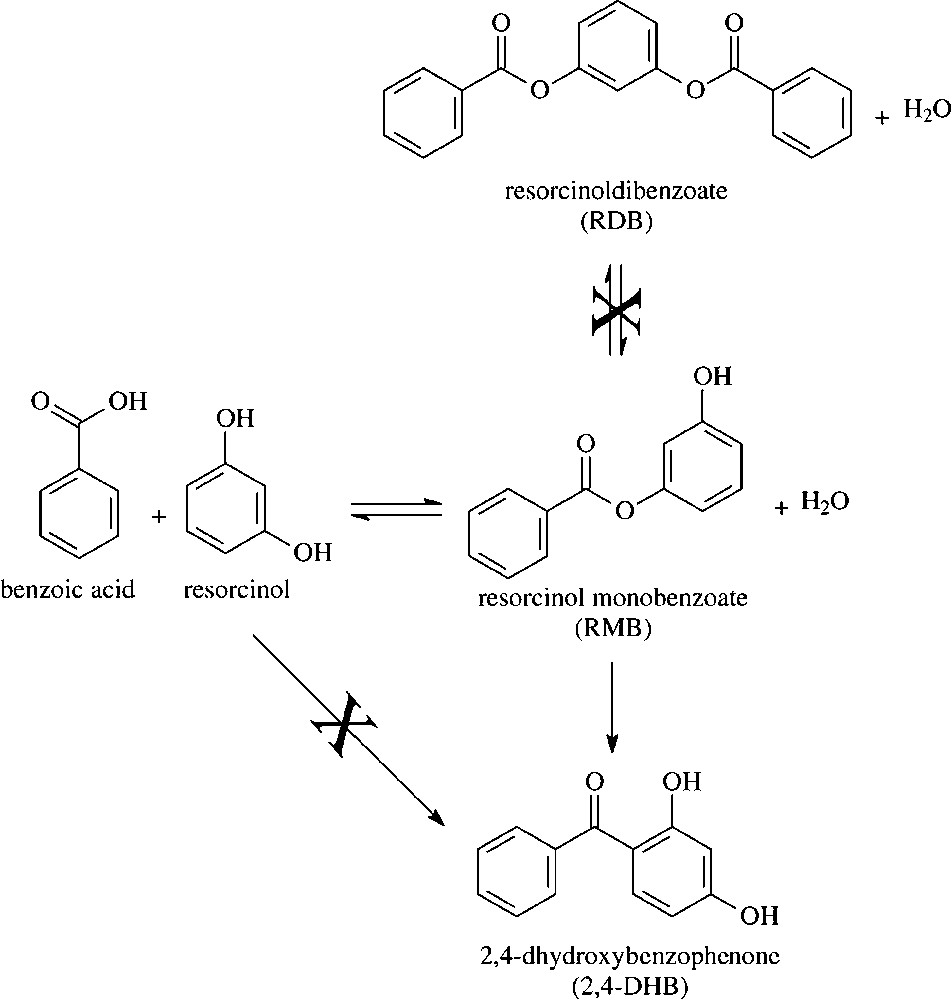
Summarizes the main findings.
3.3 The reactivity of the Süd-Chemie K series
Table 3 compares the catalytic performance of the K samples; the activity considerably decreased on decreasing the Al content in samples, despite the opposite trend of surface area. This clearly points out that the weak-medium Brønsted sites in samples, associated to silanols on the catalyst surface [22], do not play an important role in the activation of the reactants. On the contrary, the activity trend follows the amount of Al in samples. Therefore, water molecules present in samples and coordinated to Al3+ sites may act as the Brønsted active sites, through protonation of the carboxylic group; in fact, water is present in clays, which are highly hydrophilic materials. Moreover, water is the co-product of the esterification reaction, and therefore is anyway present in the reaction environment.
Catalytic performance of acid-treated montmorillonite clays. T = 145 °C, reaction time: 30 min. No solvent
Sample | R conv. (%) | RMB sel. (%) | 2,4-DHB sel. (%) |
FULCAT 22B | 52.0 | 27.8 | 72.2 |
K5 | 31.5 | 38.4 | 61.6 |
K20 | 22.1 | 62.1 | 37.9 |
K30 | 11.8 | 81.6 | 18.4 |
In order to distinguish between the possible role of Lewis-type and Brønsted-type sites, K5 and K30 samples, i.e., those samples having the highest and the lowest Al content, respectively, have been treated at increasing temperatures, in order to decrease their water content, and have been successively tested as catalysts in the benzoylation of resorcinol. Fig. 5 plots, for the two clays, the loss of weight as a function of the thermal-treatment temperature, the conversion of resorcinol and the selectivity to the products achieved for the corresponding thermally treated samples. It is shown that increasing temperature of thermal treatment led to a progressive loss of weight. Correspondingly, the conversion of resorcinol progressively decreased, and the selectivity to the primary product increased, as a consequence of the decreased level of conversion. Therefore, the data clearly point out that the catalytic activity of these materials is associated to water retained in the solid. The same occurred for FULCAT sample; a pre-treatment at 180 °C led to a decrease in the conversion from 52.0% (for the untreated sample) to 39.0% (for the treated sample).
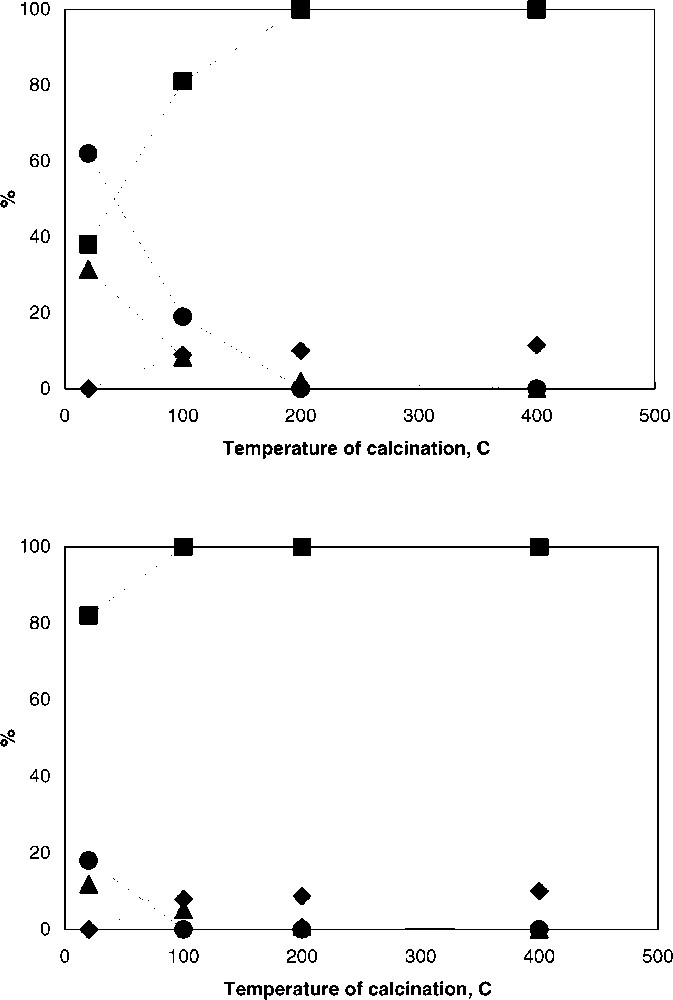
Catalyst weight loss (), resorcinol conversion (▴), selectivity to RMB (■) and to 2,4-DHB (•) as functions of the temperature of thermal treatment for catalysts K5 (top) and K30 (bottom).
Fig. 6 compares the selectivity to the two main products, RMB and 2,4-DHB, at the same level of conversion, i.e., 23–25%, for the whole K series; the silica-to-alumina wt. ratio is taken as the main compositional parameter of samples. The comparison at resorcinol iso-conversion makes possible to search for relationships between the catalyst chemical-physical features and the distribution of products; the latter in fact is affected by the reactants conversion when the reaction scheme consists of consecutive reactions. Moreover, a conversion close to 25% is far from that obtained under equilibrium conditions; therefore, under the conditions employed, the reaction is under kinetic control. It is shown that an increase in the silica-to-alumina ratio leads to an increase of the selectivity to RMB and to a corresponding decrease of selectivity to 2,4-DHB. Since the formation of 2,4-DHB exclusively occurs via consecutive reaction upon RMB, and since the formation of the latter compound is the only primary reaction contributing to resorcinol conversion, the observed effect points out a decrease of the rate of the consecutive intramolecular rearrangement of the ester to the ring-acylated compound occurring in dealuminated samples, with respect to the primary rate of RMB formation.

Selectivity to RMB () and to 2,4-DHB (■) at 23–25% resorcinol conversion as functions of the silica-to-alumina ratio in samples belonging to the K series. T = 145 °C.
Therefore, two different phenomena are evident when the alumina content is progressively decreased in catalysts: (i) a considerable decrease of catalyst activity, and (ii) a decrease of the rate of transformation of RMB into 2,4-DHB as compared to the rate of RMB formation. The effect on selectivity may be associated to the lower clay hydrophilicity and acidity that develops for increasing Si/Al ratios. This decreases the interaction of the catalyst not only with the reactants (thus contributing to the decrease of conversion), but also with RMB, leading to a lower rate of the consecutive transformation of RMB, due to both an accelerated desorption of the product from the active surface, and a slower rate of consecutive re-adsorption of the same compound, preliminary to its intramolecular rearrangement.
4 Conclusions
Acid-treated montmorillonite clays are catalysts active for the acylation of resorcinol with benzoic acid. The activity derives from Brønsted acidity, originated by the interaction of water molecules with Al3+ sites. The removal of water by thermal pre-treatment of clays considerably decreases the acidity, and thus the catalyst activity. The reaction network consists of the reversible primary reaction of resorcinol acylation to yield resorcinol monobenzoate (the contribution of the reverse reaction is low at low resorcinol conversion), followed by the consecutive Fries rearrangement to yield 2,4-dihydroxybenzophenone (scheme 1). The distribution of the products at iso-conversion is also affected by the clay characteristics.