1 Introduction
The coordination chemistry of Pb(II) has long been of interest for many reasons, one of the most obvious being its association with understanding of the toxicity of lead compounds [1–3], though there are certainly more positive features [4,5]. A fundamental aspect that has attracted considerable attention is whether or not the coordination geometry about Pb(II) reflects repulsive effects due to a ‘stereochemically active’ lone pair [6], usually considered to be the 6s2 electrons of the valence shell [7–9]. A problem in identifying the ‘valence shell’ is, of course, a common one with heavy elements, often associated with whether or not relativistic effects may be discerned in the chemistry of the element concerned [10,11]. Experimentally, this is associated with the problem of establishing the primary coordination sphere of the element, structure determinations by X-ray diffraction being the usual means of so doing. The common observation that Pb(II) is found in a very asymmetric environment of a large number (often 10 or more) of possible donor atoms [7,8], however, has often meant that this problem has been by no means easily resolved. In a particular case [12], lead–oxygen separations ranging from 2.38–3.07 Å, for example, have been considered, on the basis of bond-valence calculations, to be indicative of significant bonding interactions and there are numerous examples [5,12–18] of ‘long’ Pb···X contacts that are nonetheless much shorter than the next most distant contacts and that have thus been taken as indicative of weak attractions. Uncertainty in the definition of the primary coordination sphere donor atoms in turn generates uncertainty in the identification of any coordination sphere ‘hole’ that might be taken to signify the presence of a stereochemically active lone pair [7,15]. It is, however, common to observe a marked asymmetry in the environment of Pb(II), the structures of the two polymorphs of PbO, litharge [19] and massicot [20], providing striking examples, such that relatively short contacts lie within one hemisphere and relatively long contacts within the other, and such a ‘hemidirected’ coordination sphere [7] has been taken to be a more subtle indicator of lone-pair activity (as recently discussed in [21]). There are, nonetheless, difficulties arising essentially from the fact that most information is obtained from crystal structure determinations, as in many cases there is evidence that ‘intermolecular’ attractions in the condensed crystalline state, such as π–π stacking of aromatic ligands [15,18], may play some role in distorting the coordination sphere. Intramolecular agostic interactions (involving ligand hydrogen atoms) provide another example of such a factor, which may also be important only in the solid state [14].
Somewhat surprisingly, given the familiarity of chemistry in which Sn(II) exhibits both Lewis-acid and Lewis-base behaviour [22], little attention seems to have been paid to the possibility that the presence of a lone pair on Pb(II) may be ‘masked’ by its involvement in donor bonding. Nonetheless, there is some evidence [23] for the thesis that Pb(II) may indeed be a stronger Lewis base than Sn(II), at least when both are in the environment of oxygen donors. This concerns complexes where phenyl substituents appear close enough to be acting as (hexahapto) acceptor sites, though other cases, such as that of lead crotonate [24] (discussed in [7]), have been shown to involve approaches to Pb of simpler entities that might be considered susceptible to addition of a nucleophile. The present report of the structure of the bis(1,10-phenanthroline) complex of lead(II) nitrate-succinate provides, amongst other things, further food for thought on this issue.
2 Experimental
2.1 Synthesis
Bis(bis(1,10-phenanthroline)lead(II)) succinate-dinitrate, Pb2(phen)4(μ-C4H4O4)(NO3)2, C52H36N10O10Pb2 The initial sample of this material and the one from which the crystal used for the X-ray structure determination was drawn, was the unexpected product of a synthesis designed for other purposes. Once the constitution had been established by the structure solution, a rational synthesis was conducted as follows: a solution of disodium succinate was prepared from a mixture of Na2CO3 (106 mg) and succinic acid (118 mg) in water (5 ml) by heating it on a steam bath until all solid had dissolved and CO2 evolution had ceased. Water was added to bring the volume to 200 ml and the whole solution heated (steam bath) to ~80 °C before mixing in a solution of Pb(NO3)2 (662 mg) in water (50 ml, 80 °C). 1,10-Phenanthroline (720 mg) in methanol (50 ml) was immediately added to the hot, vigorously agitated solution and this final mixture then allowed stand to cool to room temperature. Long, very faintly yellowish needles of the product slowly deposited and were collected after 20 h, washed with water and air-dried. Yield: 1.05 g (76%). Analysis: found (calc.) for C52H36N10O10Pb2, C, 45.6 (45.41); H, 2.8 (2.64); N, 10.2 (10.18)%.
2.2 Crystallography
The crystal structure determination was performed under the supervision of Dr Z. Starikova at the X-ray Structural Centre, General and Technical Chemistry Division, Academy of Science of Russia, INEOS, Vavilov Str., 28, Moscow B-334, 117813, Russia. E-mail: star@xray.ineos.ac.ru. Basic data are given in Table 1. A .cif file has been deposited with the CCDC, deposition number 231871. These data may be obtained free of charge via www.ccdc.cam.ac.uk/conts/retrieving.html or from the Cambridge Crystallographic Data Centre, 12 Union Road, Cambridge CB2 1EZ, UK; Fax. (+44) 1223-336-033 or deposit@ccdc.cam.ac.uk.
Crystal and refinement data for Bis(1,10-phenanthroline)lead(II) nitrate–succinate
Chemical formula | Pb2(phen)4(μ-C4H4O4)(NO3)2 |
C52H36N10O10Pb2 | |
M (g mol–1) | 1375.3 |
Space group | P (No. 2) |
a (Å) | 9.052(2) |
b (Å) | 11.522(2) |
c (Å) | 11.956(2) |
α (°) | 96.43(1) |
β (°) | 109.58(1) |
γ (°) | 100.82(1) |
V (Å3) | 1133.2(3) |
Z | 1 |
Dcalc (g cm–3) | 2.015 |
μ(Mo Kα) (mm–1) | 7.50 |
Crystal size (mm) | 0.36 × 0.22 × 0.15 |
λ (Å) | 0.71073 |
T (K) | 293(2) |
R1 | 0.027 |
wR2 | 0.063 |
3 Results and discussion
The present succinate–nitrate adds to the quite extensive family of ‘mixed-anion’ lead(II) compounds known [15,21], with the presence of nitrate here being formally a consequence of the fact that the succinate acts as a bridge between two lead centres rather than acting as a chelate to one. Interestingly, if a limit of 2.6 Å were to be placed upon Pb(II)-donor atom separations regarded as involving coordinate bonding, only one oxygen atom of a succinate carboxylate group would be considered bound and the nitrate groups considered uncoordinated. Further, only one nitrogen centre of each of two phenanthroline ligands would be bound to each Pb centre and the Pb atoms would thus be 3-coordinate and pyramidal, as in the representation of the centrosymmetric stoichiometric unit shown in Fig. 1. If, however, the bonding limit is extended to 2.75 Å, the succinate carboxylate and both phenanthroline units attached to each Pb all become (asymmetric) chelating entities, leading to Pb atoms that are 6-coordinate in a very strongly ‘hemidirected’ array (Fig. 2a), evidently consistent with the presence of a lone pair in the apparent coordination-sphere vacancy remote from the bound atoms. To find any potential donor centre within this ‘vacancy’, it is necessary to extend the bonding limit to at least 3.30 Å, in which case one nitrate-O would become coordinated, while only at 3.62 Å could a second nitrate-O (of a different anion) be considered to enter the coordination sphere (Fig. 2b). It is possible, nonetheless, to view the Pb atoms of Pb-succinate-Pb units as linked via Pb2O2 rhombs (Fig. 2b), geometrically a feature of many Pb(II) complex structures [15,17,18,23,24], involving these two nitrate-O atoms. Further, at a limit of < 3.9 Å, it is possible to find two phenanthroline-C atoms (and their associated hydrogen atoms) approaching each Pb, thus giving each metal atom a very asymmetric environment (Table 2) of ten possible donor centres or eight donors plus two acceptors, if a formal double bond of phenanthroline could be regarded as undergoing addition of the Pb via its lone pair (Fig. 2c). Lead-aromatic carbon separations similar to the present have been observed in several instances (see [23]) and are similar to those observed for lead-unsaturated-C in lead(II) crotonate [25], for example (Fig. 2d). In the present case, at least, it is possible that these approaches are indicative of agostic Pb···HC interactions, a possibility which is consistent with the fact that no significant distortion of the aromatic ring geometry is apparent. The Pb···H separations (3.27, 3.55 Å) do seem too long [14], however, for any such interaction to be significant.
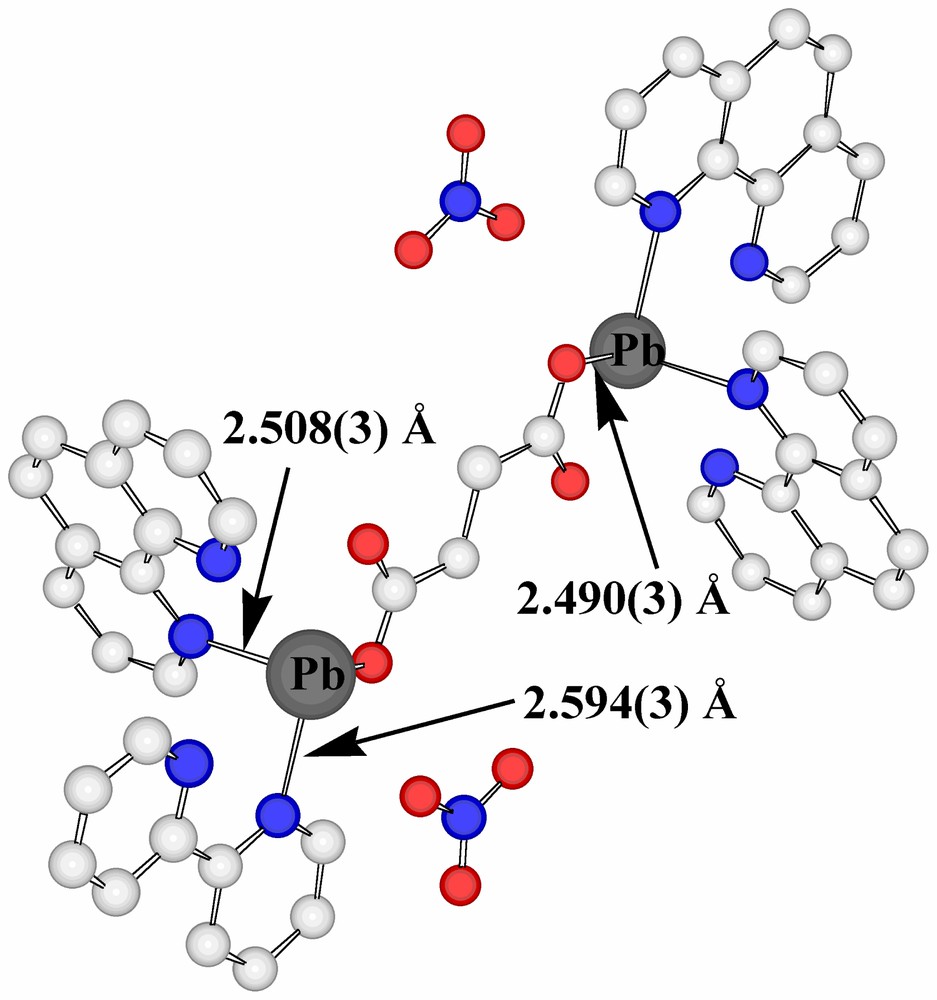
A view of the centrosymmetric stoichiometric unit found within the lattice of Pb2(phen)4(μ-C4H4O4)(NO3)2. Only contacts to Pb of < 2.6 Å are shown as bonds. Here and in the following figures, except where specifically noted, atoms are colour-coded as C = white, N = blue, O = red, Pb = grey.
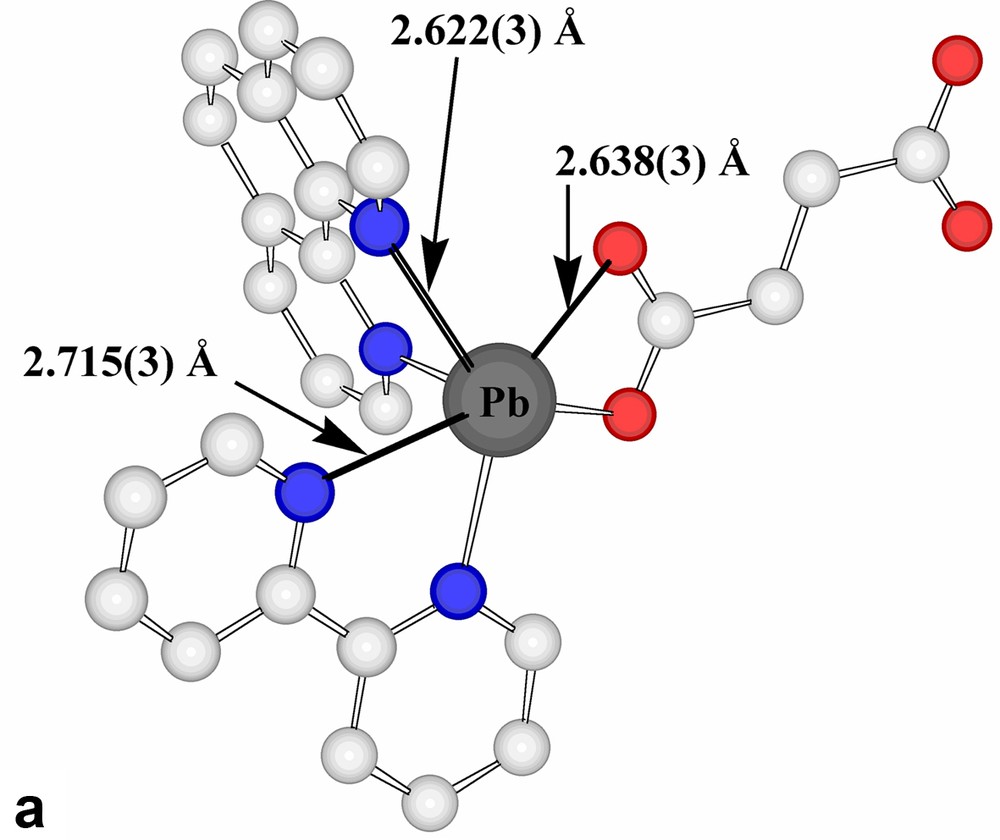
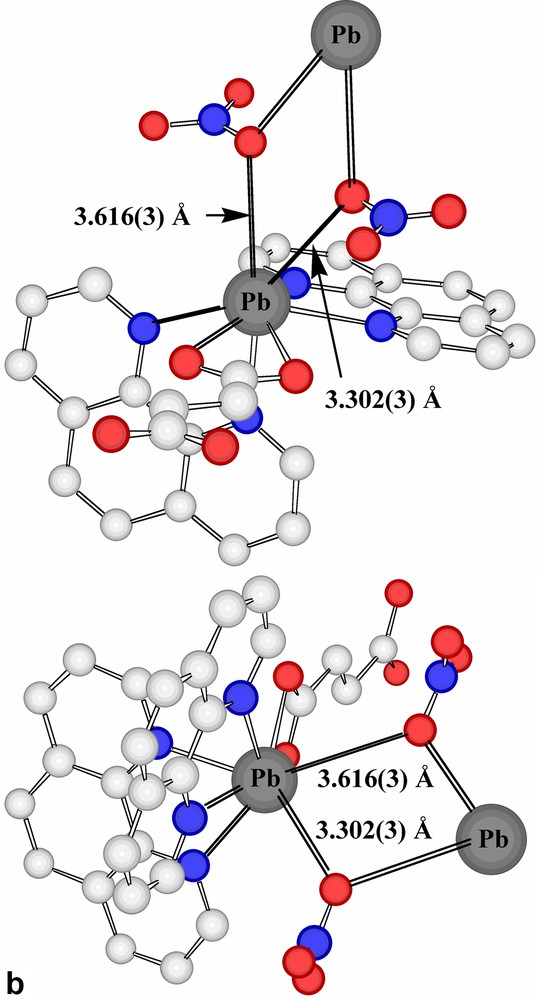
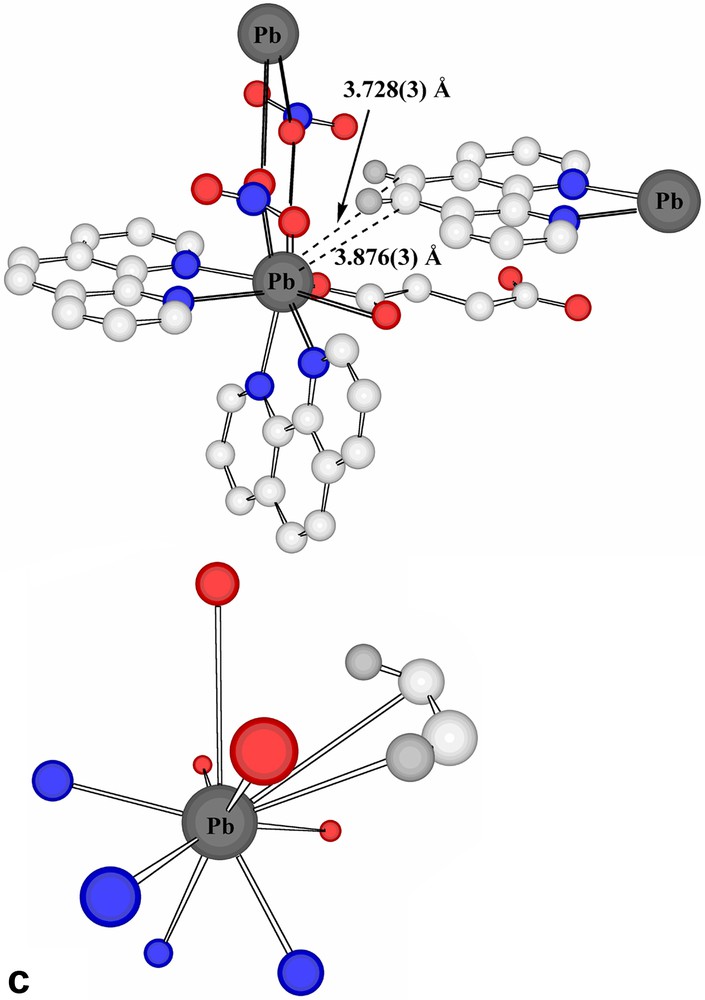
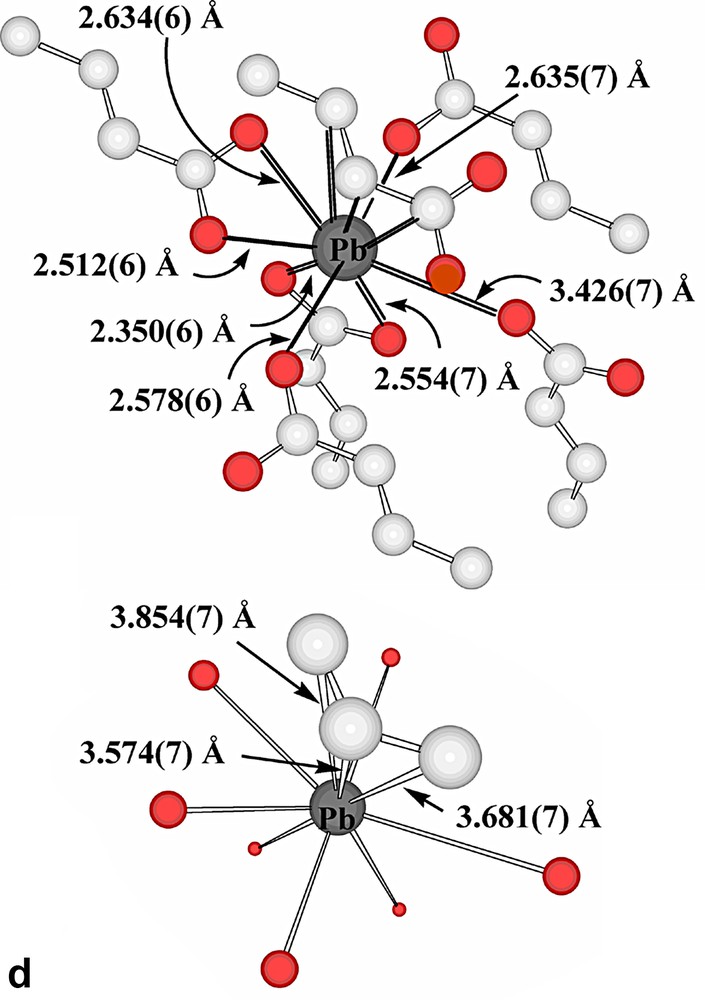
(a) Expansion of the coordination sphere of Pb in Pb2(phen)4(μ-C4H4O4)(NO3)2 to include contacts at < 2.75 Å as bonds (reflected in chelation of the phenanthroline and succinate entities). (b) Two views of the consequences for Pb coordination of expanding the bonding limit to 3.62 Å. The Pb2O2 rhomb formed as a result of bridging by nitrate-O atoms to a Pb of an adjacent succinate-bridged dimer is also shown. (c) The coordination environment resulting for Pb in Pb2(phen)4(μ-C4H4O4)(NO3)2 when the bonding limit is extended to 3.9 Å. The lower figure shows simply Pb and the contact atoms. (H atoms shown light grey.) (d) The coordination environment of Pb in lead crotonate [24] when the criterion of a bonding contact is relaxed to a separation of < 3.9 Å. For clarity, Pb···C(crotonate) separations are shown on the simplified representation.
Geometry of the Pb(II)C2N4O4 coordination sphere, regarded as comprised of five chelating units as in Fig. 2c
Chelate Unit | Pb···Da (Å) | D–Pb–D (˚) |
Phenanthroline Ab | 2.594(3), 2.715(3) | 62.2(1) |
Phenanthroline Bb | 2.508(3), 2.622(3) | 64.5(1) |
Succinate carboxylate | 2.490(3), 2.638(3) | 50.7(1) |
Pb(ONO2)2 | 3.302(3), 3.613(3) | 60.4(1) |
C2 unit of phenanthroline A′ | 3.726(3), 3.876(3) | 73.6(1) |
a D = donor atom (C, N or O).
b See text for identification of the inequivalent phenanthroline ligands.
Extending considerations beyond the immediate environment of a given lead atom, a view of the lattice of Pb2(phen)4(μ-C4H4O4)(NO3)2 down c (Fig. 3a) shows the Pb atoms to lie in columns, with the minimum separation between atoms in separate columns, 5.768 Å being that of those pairs bridged by nitrate anions. Succinate entities, in which each O4C4 unit is close to planar, provide longer links between Pb atoms 9.574 Å apart (Fig. 3b), and lie in planes only slightly inclined to the c axis and close to parallel to the planes of the nitrate anions. One set (A) of phenanthroline ligands (Fig. 3c (i–ii)) also lies in planes nearly parallel with those of the succinates, in such a way that pairs of phenanthroline ligands are separated by single succinate entities. Since the two phenanthroline ligands on each metal have different orientations, it is unsurprising, given the importance of π–π stacking and other interaromatic attractions apparent in the solid-state structures of numerous complexes of azaaromatic ligands [26,27], to find that the lattice contains two arrays of parallel phenanthroline units. The second set (B) of phenanthroline ligands lies in planes nearly orthogonal to those of the first, though in this case forming an essentially infinite ‘slipped’ stack [27] of aromatic entities (Fig. 3d (i–ii)).
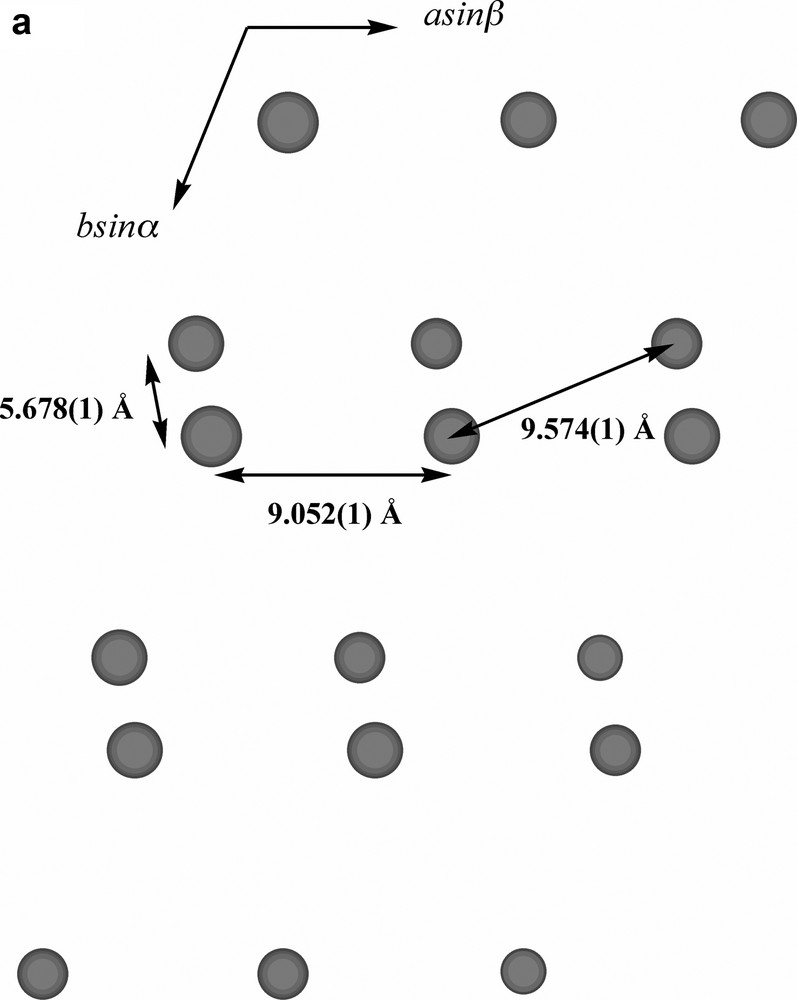
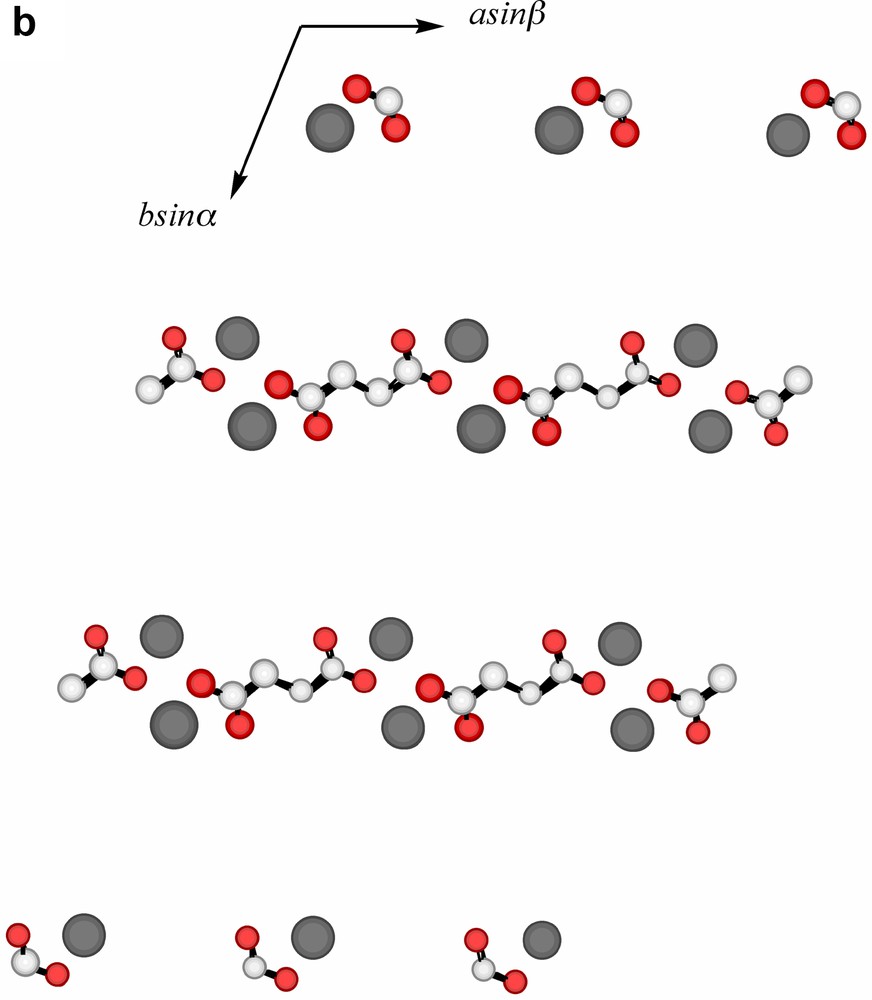
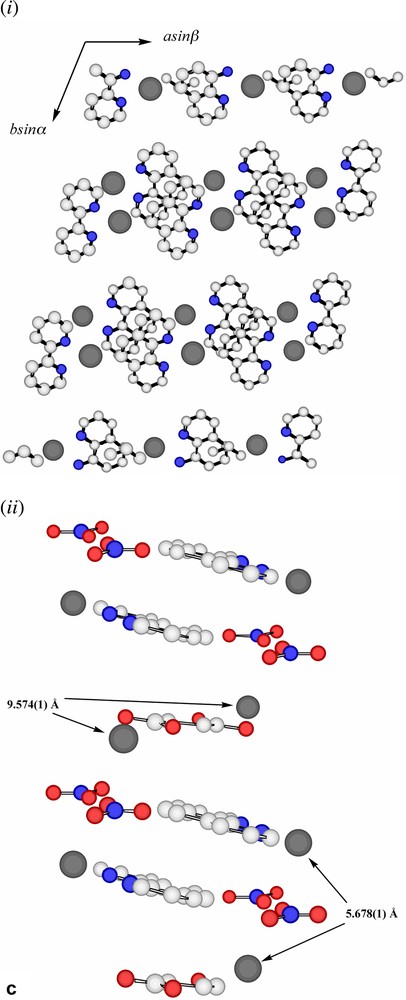
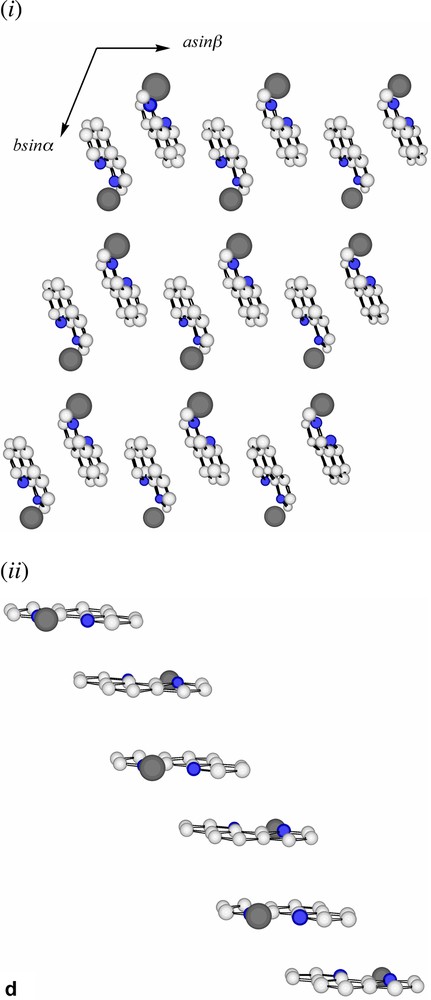
(a) A view, down c, of the array of Pb atoms in the lattice of Pb2(phen)4(μ-C4H4O4)(NO3)2. Each visible atom is in fact the head of a column, the shortest distances between atoms in the different columns being indicated. The separation of 5.678(1) Å is that between Pb atoms bridged by nitrate (Fig. 2b) and that of 9.574(1) Å that of the Pb atoms linked by succinate. (b) A similar view to that in (a), with succinate entities added. Bonds to Pb are not shown. (c) (i) A lattice view as in (a) showing Pb atoms and phenanthroline units of type A (see text). These phenanthroline units form columns in which the succinate units are interposed (see (ii)). (ii) A view, near orthogonal to that in (i), showing how the nitrate, succinate and phenanthroline-A entities lie in near parallel planes and how pairs of phenanthroline-A ligands are separated by single succinate units. (d) (i) A lattice view as in (a) showing Pb atoms and phenanthroline units of type B (see text). The alternating orientations of the phenanthroline units in the (horizontal) stacks are apparent. (ii) An approximately orthogonal view to that of (i) showing the ‘slipped’ form of the stacking.
Interestingly, the projection of adjacent phenanthroline rings upon one another is significantly different in the two stacks (Fig. 4a), though both show close atom approaches ~3.47 Å, typical of systems conventionally described as being involved in π–π stacking [26,27], though the actual nature of the projection shows a further variation on those known for coordinated phenanthroline in other Pb(II) complexes [15]. Presumably, such differences in projection are the consequences of other interactions involving the ligands (a parallel to the behaviour of aromatic ligands such as picrate [28] and various others [29]), the most obvious of these being N-and C-coordination to Pb, though there are several close contacts of other forms apparent, including ‘edge-to-face’ approaches associated with partial interlocking of the two phenanthroline arrays (Fig. 4b). Thus, each succinate unit is ‘sandwiched’ between two phenanthroline units in such a way that there are aromatic-C-carboxylate-O approaches of 3.49 Å and methylene-C-aromatic-C approaches (perhaps indicative of CH2-π interactions [30] in that the methylene-C is almost equidistant from two aromatic-C) of 3.71 Å (Fig. 5a). As well, the nitrate anions are involved in a complicated array of contacts with both phenanthroline A and B arrays (Fig. 5b and c). The shorter of these contacts (3.37–3.42 Å) are perhaps best taken as indicative of CH···O interactions, though there are only slightly longer contacts (~3.54 Å) suggestive of a form of stacking of nitrate-O on an aromatic ring.
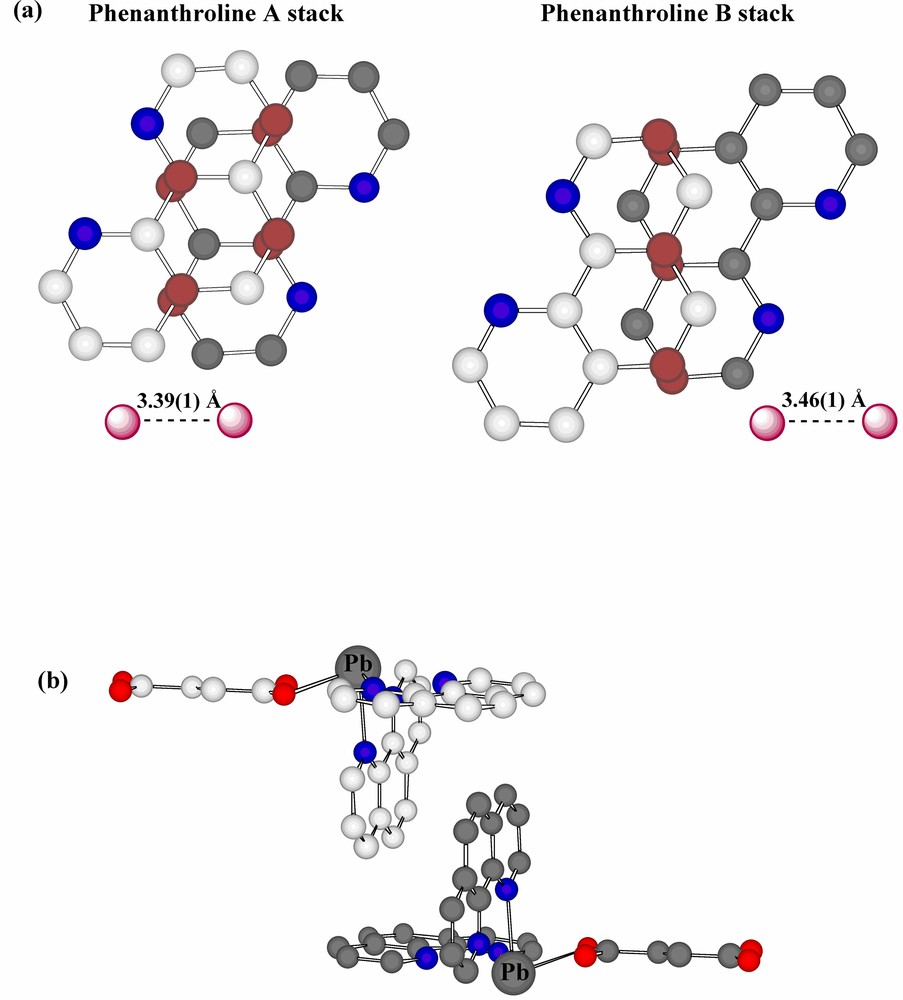
(a) Views, perpendicular to the ring planes, showing the projections of adjacent rings onto one another in the two phenanthroline stacking arrays. For contrast, carbon atoms of one ring are shown in grey and the atoms in closest contact are shown in dull purple. (b) A partial view showing interlocking of the complex units which results in ‘edge-to-face’ contacts of phenanthroline A and B units with atom separations ~3.8 Å.
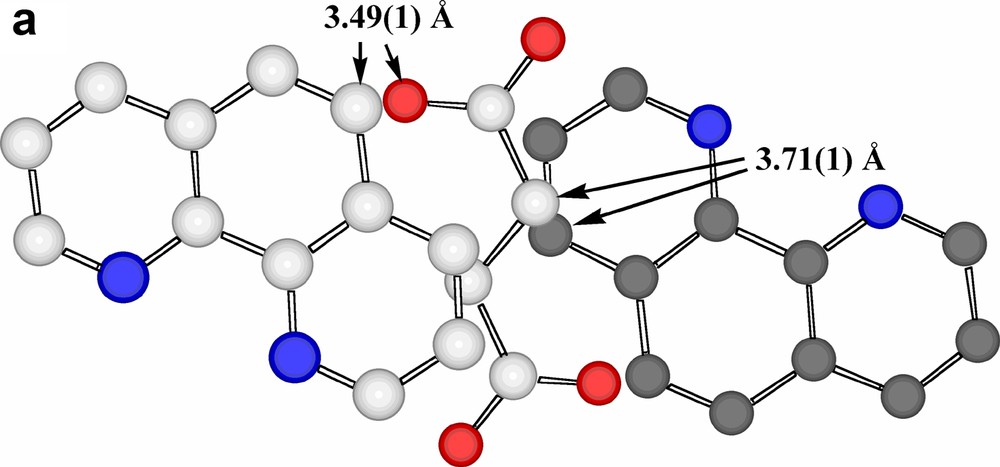
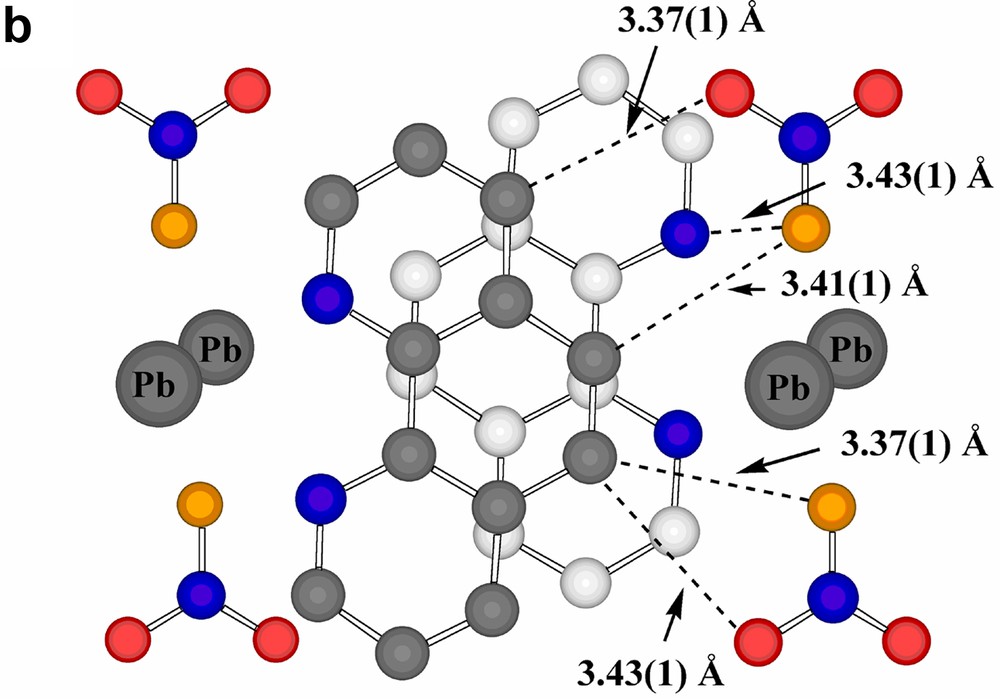
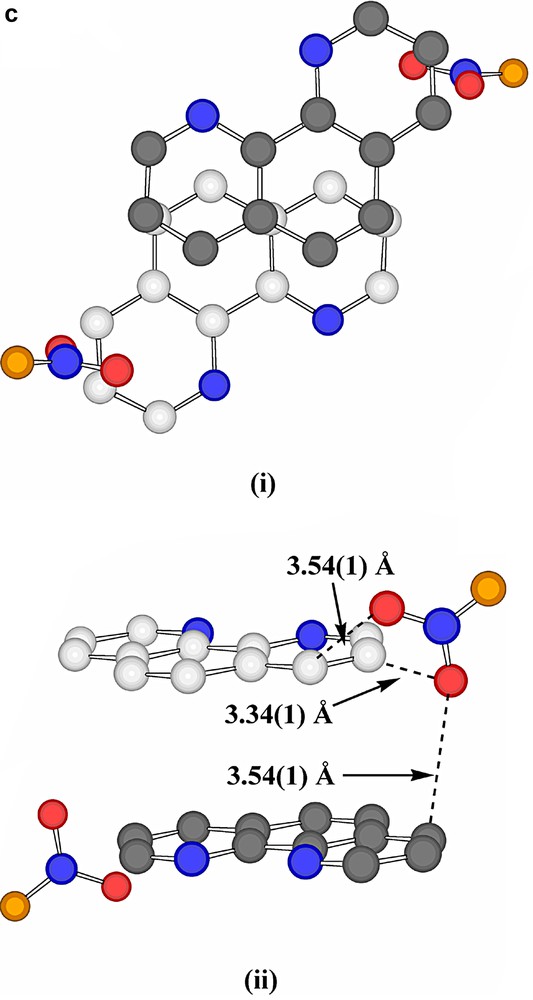
(a) A view, perpendicular to the phenanthroline ring plane, of a phenanthroline-A/succinate/phenanthroline-A sequence within the column running down c. (Again, one ring has carbon atoms shaded grey.) (b) A view, perpendicular to the phenanthroline ring plane, of a pair of adjacent phenanthroline-A ligands and their nearest neighbour nitrate entities. Short contacts, which may be of both CH···O H-bonding and stacking interactions origins, are shown as dotted lines. The nitrate-O atom involved in bonding to Pb (shown) is coloured in orange. (c) Views, (i) perpendicular to the phenanthroline ring plane and (ii) across this plane, of an adjacent pair of phenanthroline-B ligands and their nearest-neighbour nitrate entities. Again, the shorter contacts are shown as dotted lines, the carbons of one ring are shaded grey and the nitrate-O bound to Pb is shown in orange.
A useful comparison with the present work is provided by a recent structural study of three complexes of Pb(II) with the divergent quadridentate ligand anion tetrakis(imidazolato)borate, [B(Im)4]– [21,31] (Fig. 6a). The nature of the ligand is such that it functions as a bridging ligand and gives in these cases coordination polymers with four imidazole-N donors from four separate anions bound to each lead atom, thus providing an N4 donor-atom array equivalent to that provided by the two phenanthroline ligands in the present case. It is associated with a 1:1 stoichiometry Pb:[B(Im)4]–, so that another anion is required for charge neutrality, leading to the isolation of the new ‘mixed-anion’ species Pb(B(Im)4)(NO3)·n H2O, Pb(B(Im)4)I and Pb(B(Im)4)(C6H5CO2)·0.5 H2O. The lattice array of [B(Im)4]– units is essentially identical in all cases and may in part be maintained by various aromatic interactions (‘multiple imidazolate embraces’?) [26–28,32] involving the imidazolate entities (Fig. 6b), again a parallel with the stacking interactions of the phenanthroline ligands in Pb2(phen)4(μ-C4H4O4)(NO3)2. The Pb···N contacts considered as bonding in these structures range from 2.38 to 2.78 Å (associated with some apparent differences in total coordination numbers), typifying the bond length variability so commonly seen with Pb(II). On this point, it may be noted that, although the nitrate anions in Pb(B(Im)4)(NO3)·n H2O have been described as ‘uncoordinated’ [21], relaxation of the bond length criterion for Pb–O would allow the nitrates here to be considered as chelating-bridging units, creating links between pairs of Pb atoms 4.420 Å apart through formation of Pb2O2 rhombs (Fig. 6c) similar to those identified in other lead-nitrate derivatives [24]. The longer side of this rhomb is of a length (3.319 Å) similar to the separation between Pb and a water-molecule oxygen (3.156 Å), so that a (presumably) weak coordinate bond to water might be added to the lead-atom environment. (This water molecule is also ‘coordinated’ to nitrate through H-bonding.) Thus, although the four imidazolate N-donors may be considered to occupy a hemisphere about Pb, the PbN4O4 entity defined by allowing these extra interactions, though markedly asymmetric, is essentially a ‘holodirected’ [7] array (Fig. 6d), with the only obvious ‘vacancy’ being in the region where bridging to the adjacent Pb may be involved.
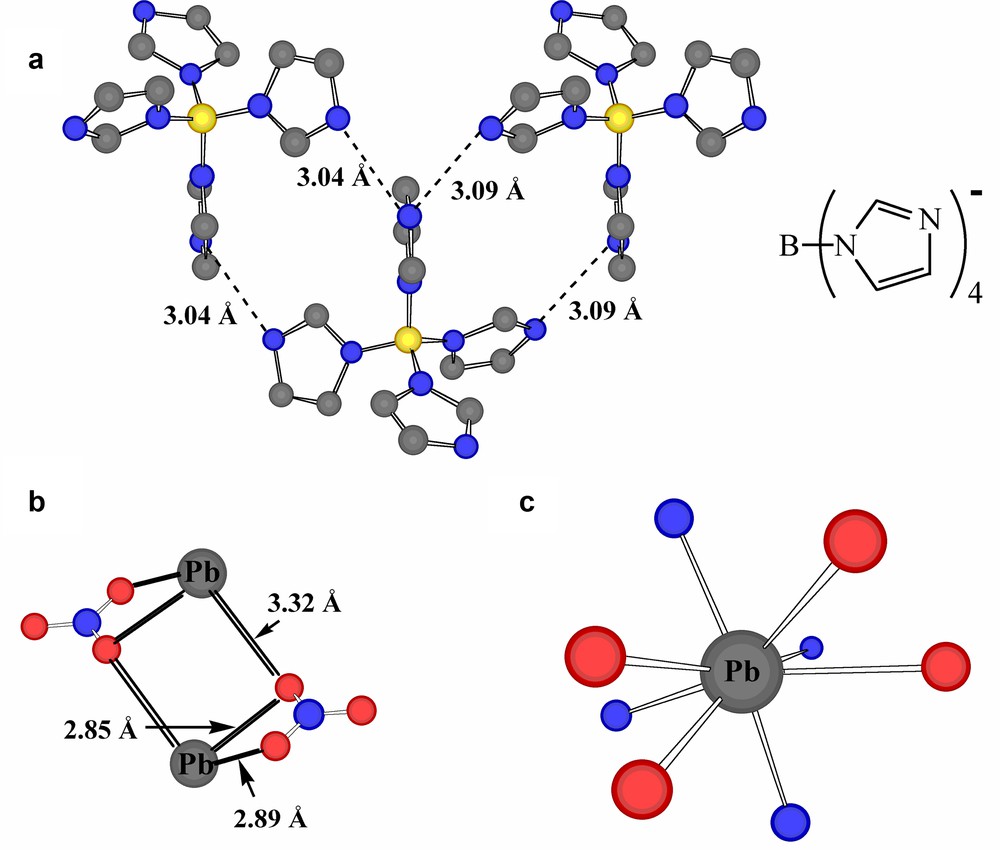
(a) Part of the array of tetrakis(imidazoyl)borate anion, [B(Im)4]– (inset), anions found in the lattice of the coordination polymer Pb(B(Im)4)NO3·n H2O [21], showing N···N approaches. Note that although there is an array of parallel imidazoyl rings, the closest atom···atom contacts there are > 4.5 Å. (b) Nitrate-bridging of Pb atoms within Pb(B(Im)4)NO3·n H2O. (c) A representation of the expanded coordination sphere possible for Pb in Pb(B(Im)4)NO3·n H2O if the criterion of a bonding interaction is extended to a separation of 3.32 Å.
In the case of Pb(B(Im)4)I, although rhombic Pb2I2 units may be discerned in the lattice (and are well-characterised in other Pb compounds [33]) and the Pb···Pb separation is shorter (3.935 Å) than in the Pb2O2 rhombs of the nitrate, one side of the rhomb is 4.556 Å long and seems extreme as an indicator of any significant interaction (Pb···I of 3.1507(7) Å for the other side of this rhomb being described as an example of a strong interaction [21], while the maximum Pb···I observed in other systems is 3.627(2) Å [33c]). Nonetheless, on the ‘open’ side of the seemingly ‘hemidirected’ metal, there are approaches of C and N atoms (3.757 and 3.656 Å, respectively) of one formally ‘uncoordinated’ (to the given Pb) imidazole ring similar to those found in cases of polyhapto interactions of Pb with aromatic rings. These approaches are also similar to those between the iodide units and imidazole ring atoms. Overall, while the Pb environment is clearly very asymmetric, there is again a donor atom sheath that can be said to fully envelop the metal (Fig. 7).
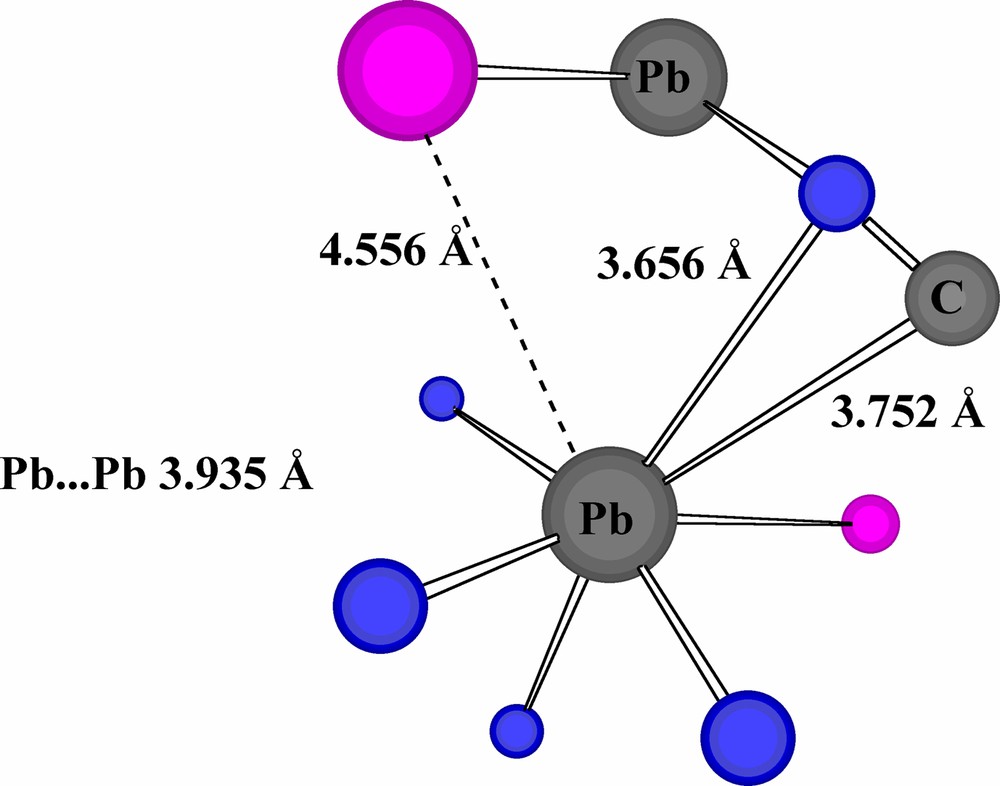
An expanded coordination sphere for Pb in Pb(B(Im)4)I [21] (I = violet).
In Pb(B(Im)4)(C6H5CO2)·0.5 H2O, the shortest Pb···Pb separation (6.269 Å) is considerably greater than in either of the other two complexes and no oxyanion bridging is apparent. Two adjacent benzoate units (linked by hydrogen bonding to water molecules) can, however, be regarded as bridging these Pb atoms in that the carboxylate-O atoms chelate one Pb while the ipso-C and its two adjacent C atoms lie at 3.464, 3.577 and 3.592 Å of the second Pb, consistent with trihapto coordination of the aromatic ring. Whether the ring should in fact be regarded as bound in an asymmetric hexahapto fashion to the Pb, given Pb···C separations of 3.806, 3.924 and 3.821 Å for the other ring atoms, is a question which typifies the difficulties involved in assessing the coordination chemistry of Pb(II), as is that as to whether this aromatic ring approach should be considered due to the action of Pb(II) as an acceptor or as a donor [23]. In any case, it is again the case that the metal may be regarded as fully enveloped by a donor-atom array (Fig. 8). Interestingly, the presence of two relatively short Pb···O interactions in this species is associated with significant lengthening of two Pb···N interactions (compared to those in the nitrate and iodide), consistent with the notion that the coordination geometry of Pb(II) may be very readily distorted in response to seemingly quite minor changes in its complete environment. Indeed, the fact that benzoate forms a nearly symmetrical chelate ring while succinate is bound as a markedly asymmetric chelate in Pb2(phen)4(μ-C4H4O4)(NO3)2 may be seen as further evidence for this, as can the variety of Pb···O(carboxylate) distances seen in other carboxylate complexes, e.g. [4,12,34,35,24b].
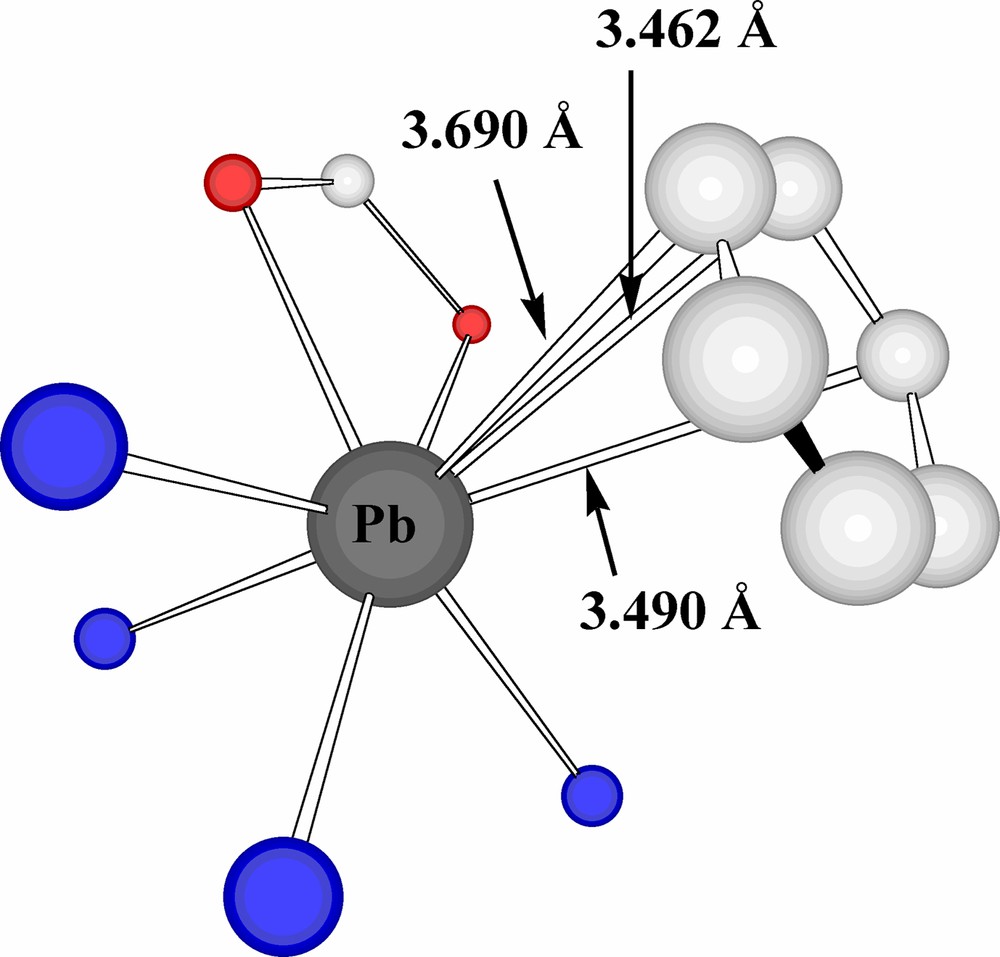
An expanded coordination sphere for Pb in Pb(B(Im)4)(O2CC6H5)·0.5 H2O.
4 Conclusions
The question of whether or not there is evidence for lone-pair activity in Pb(II) complex structures is, in a certain sense, an artificiality, since it is based upon the formal allocation of charge distribution implicit in oxidation-state designation. In most instances, the coordination number of Pb, albeit sometimes difficult to establish, is high enough to require that all valence shell orbitals could be used in the formation of 2c–2e bonds and the question of the exact distribution of electron density over the actual molecular orbitals of the complex can only be resolved through highly sophisticated calculations [7,11]. What is apparent from the extensive structural information now available on Pb(II) compounds is that the coordination environment of the metal is sensitive to a variety of influences that may be considered to originate from interactions well outside the primary coordination sphere. Given the high coordination numbers and the inherently relatively weak bonding interactions of a metal such as Pb [11], this is perhaps unsurprising. A question that remains, nonetheless, is whether it is useful, in a qualitative sense, to attempt to analyse the coordination chemistry of Pb(II) in terms of a central metal accompanied by a lone pair of electrons. Given the ambiguity of most structural evidence for coordination sphere vacancies and the lack of geometric distortions of the ligands in cases where addition of the lone pair might be considered possible, the answer would seem to be that it is not. The extreme of claiming that the possibility of ‘lone-pair’ effects should be ignored because many other factors can be discerned is, all the same, untenable, since the total electron population must have some influence and clearly the possibility of Pb(II) acting as a Lewis-base centre is worthy of consideration. Thus, although it is possible to be misled by the coordination chemistry of lead, the bifunctionality of Pb(II) is a useful reminder of the subtlety of metal–ligand interactions.