1 Introduction
Ordered mesoporous silicas are interesting hosts for organic chromophores, as the optical systems so obtained fall between microporous hosts (zeolites), that can align small molecules, and patterned micron-scale photonic band-gap materials [1–3]. In particular, the dye Disperse-Red-1 (2-{[4-(4-nitrophenylazo)-phenyl]-ethyl-amino}-ethanol), hereafter referred to as DR1) is a push–pull chromophore which, together with its derivatives, has long been investigated as optical nonlinear dopant [4] in polymers [4–7], sol-gel silica matrices [8–11] and organic–inorganic hybrid materials [10–13]. Inclusion in HY zeolite has also been obtained via an in situ (ship-in-the-bottle) procedure [14].
Covalent anchoring of chromophores to mesoporous silica surface is generally carried out through post-synthesis treatments, implying: (i) removal of the surfactant, usually by calcination of the mesophase; (ii) modification of the surface, e.g. by silanation with 3-aminopropyltriethoxysilane; (iii) grafting onto the functional group of the organic molecule [15–18].
A different possibility is the covalent bonding of dye molecules to the silica surface by co-condensation [19], which only requires the functionalization of the dye with a suitable group (e.g., a triethoxysilane arm). This procedure has been first reported by Lebeau et al. [20,21]. Ganschow et al. [22] then reported the anchoring of an azo dye and rhodamine dye to MCM-41 internal surface. In this latter case, co-condensation was microwave-assisted to reduce the reaction time and avoid dye degradation during the hydrothermal synthesis. However, this procedure is not entirely safe, as it generates relatively high temperatures, albeit for a short time.
Object of the present paper is the covalent bonding of DR1 to the inner surface of mesostructured silica via a room temperature one-step co-condensation route. To this purpose a suitable DR1 derivative (named DR1UPTEOS) [8], depicted in Scheme 1, has been used. Neutral amine templated mesoporous silica (HMS) were chosen as supports, because the template used in the synthesis (as first reported by Tanev and Pinnavaia [23]) are long-chain amines, readily removable by solvent extraction in mild conditions. Besides being carried out at relatively low temperatures, neutral extraction of amines avoids protonation of the dye molecules, which may have impact on the optical properties of the chromophore: indeed, in contrast, quaternary ammonium ions templated systems such as MCM-41 require HCl treatment.

Besides the sheer synthetic target, other features are of interest. On the one hand, to check whether quite bulky molecules, like DR1UPTEOS, affect the assembling of amine-silica mesophases, and therefore, their structural periodic properties. On the other hand, the dye molecule may act as a probe of the environment, yielding information about its location in the mesostructure, as observed by Ganschow et al. [22]. Knowledge of these features may be important when considering dye-modified mesostructured silicas as alternatives to sol-gel glasses for applications in optics.
2 Experimental section
DR1 has been prepared following the classical literature procedure, modified introducing the triethoxy silane functionality according to Ref. [8], so obtaining the species DR1UPTEOS. Two samples of DR1-modified HMS silica have been prepared, differing for dye content.
For the synthesis, TEOS (9.145 g, Aldrich) and DR1UPTEOS (either 0.014 or 0.050 g) were added simultaneously to a stirred mixture of n-dodecylamine (2.33 g, Aldrich), absolute ethanol (11.85 g, Labochem) and distilled water (35 g, Carlo Erba).
Molar ratios of the synthesis solution were accordingly:
1 TEOS:(2.2 × 10–3–6.2 × 10–4) DR1: 0.013 n-dodecylammine: 0.25 EtOH: 1.94 H2O |
Surfactant-containing materials will be denoted hereafter as A-0 and B-0, respectively.
The amine was removed by refluxing the solid (ca. 500 mg) in boiling solvent (150 ml) for 6 h. The extraction was repeated two times. Two different solvents have been used, absolute ethanol and acetone: the solvent used is indicated hereafter by a second letter A or E, so that, e.g. B-E means the sample with higher dye content extracted with ethanol. In all cases, after extraction the solvent was only pale colored, indicating that no significant dye leaching had occurred. Instead the powders were strongly colored, and showed different hues.
Reference measurements were carried out on three systems: (i) DR1 in a water–ethanol-surfactant solution without any silica (with the same molar ratio of the synthesis solution); (ii) DR1-free material; (iii) DR1-containing HMS obtained by adding the same solution as above to the synthesis mixture before TEOS, so to get a HMS system containing un-anchored DR1 (sample R). With the latter, however, extraction brings about the complete leaching of the dye and yields a colorless powder.
Samples were characterized by XRD (Philips X’pert, CuKα radiation), N2 adsorption (Micromeritics ASAP2000) for surface area and porous size distribution evaluation, and by FTIR spectroscopy (Bruker Equinox 55, equipped with MCT detector). For FTIR measurements powders have been pressed into self-supporting discs and located in a cell allowing outgassing (residual pressure < 10–3 mbar).
Optical properties have been investigated by means of UV–visible spectroscopy (Varian Cary 500) working in diffuse reflectance mode. Powders have been located in quartz cell and subjected to room temperature outgassing before measurement (residual pressure < 10–3 mbar): the same cell allows controlled gas adsorption.
Chemical analysis has been carried out on the extracted B samples. The nitrogen content was found to be about 0.20% (in weight) for both B-A and B-E samples. The value estimated on the basis of the dye content in the synthesis solution is 0.23%. This evidence confirms that no significant loss of dye occurred during the synthesis and extraction of materials.
3 Results
3.1 Structural characterization
Fig. 1a reports the XRD patterns of extracted B samples. The (100) reflection is observed at about 2θ = 2.2°, together with a diffuse scattering at about 2θ = 4.5°. This pattern is typical of these wormhole-like materials [24]. A value of 3.9 nm is calculated for d100 of both samples.
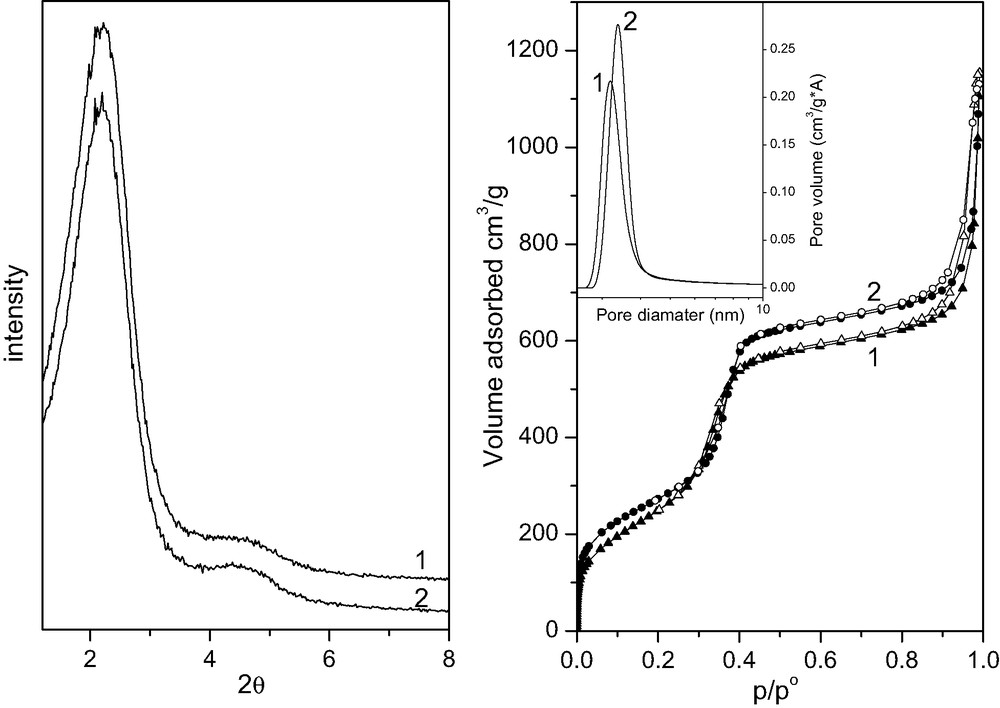
Section a: XRD patterns of B-E (curve 1) and B-A (curve 2). Section b: N2 adsorption (full symbols) and desorption (hollow symbols) branches of B-E (1) and B-A (2). Inset: BdB pore size distributions of B-E (curve 1) and B-A (curve 2).
Similar XRD patterns, not reported, were obtained for samples A, R and for the dye-free HMS. Whereas for sample A basically the same d100 is observed, slightly different values are obtained for sample R and for the DR1-free HMS (4.1 and 3.5 nm, respectively).
Fig. 1b reports the N2 adsorption isotherm related to samples B-E and B-A. A typical type IV isotherm is observed, with pore filling occurring in the range 0.3–0.41 p/p0, and inflection point of the desorption isotherm at about 0.33 and 0.36 p/p0 for B-E and B-A, respectively.
At pressure above 0.9 p/p0 a hysteresis loop is observed, due to condensation in textural porosity. The same feature has been reported by Pinnavaia and coworkers for samples prepared similarly [24,25].
The surface areas calculated by the Brunauer–Emmet–Teller (BET) method for the portion of isotherm between 0.05 and 0.2 p/p0 are 933 and 1000 m2 g–1, for B-E and B-A, respectively: corresponding CBET values are about 40 and 70. The pore size distributions in terms of the Barret–Joyner–Halenda (BJH) [26] model are centered at 2.6 and 2.8 nm, respectively, while Broekhoff and de Boer [27] and Horvath and Kawazoe [28] models yield to same values of 3.2 and 3.4 nm for B-E and B-A (inset to Fig. 1b). We favor these latter, as the BJH values are probably underestimated [29–32].
3.2 FTIR characterization
Fig. 2 shows the IR spectra of B-E and B-A samples outgassed at room temperature (curves 1 and 2, respectively). Bands marked by an asterisk at 1603, 1588, 1518 and 1345 cm–1 are due to DR1, i.e. to C=C stretching ring modes [33], and to NO2 symmetric stretching mode [34], respectively.
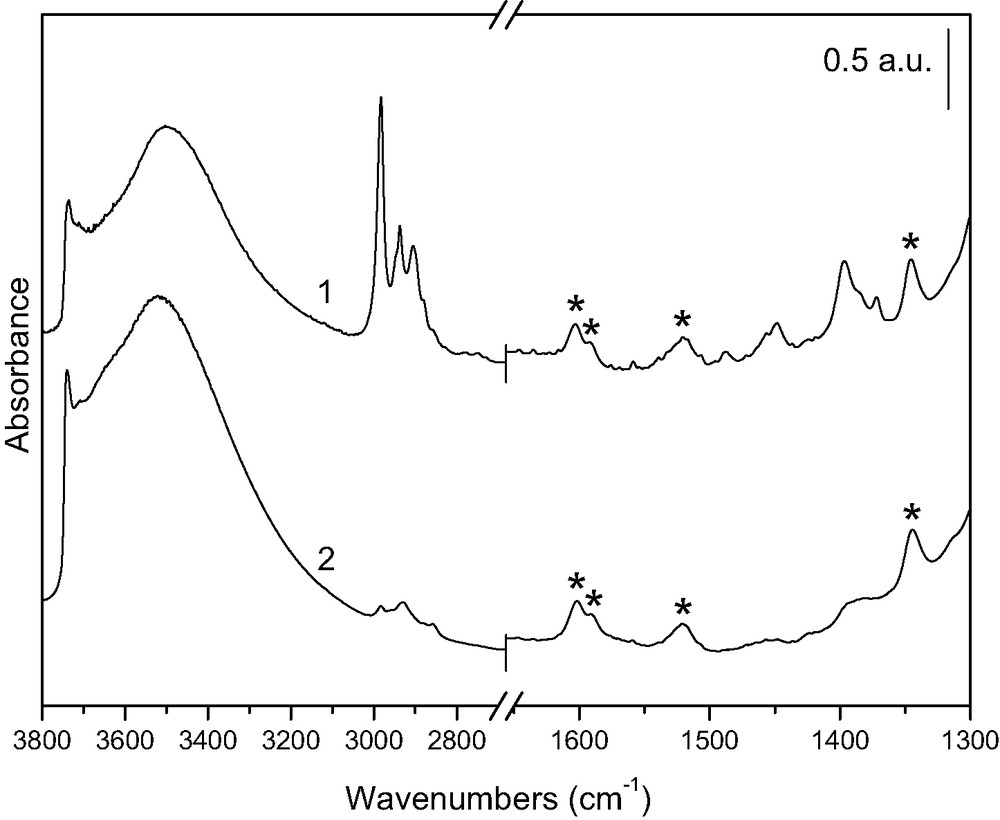
FTIR spectra of B-E (curve 1) and B-A (curve 2) outgassed at room temperature. Asterisks indicate chromophore modes.
In the spectrum of B-E, bands are observed at 1395 and 1448–1455 cm–1, together with intense peaks at 2983, 2937 and 2905 cm–1. These are due to CH3 and CH2 modes of ethoxy groups, formed by reaction of the surface with hot ethanol during the extraction [35], according to the equation:
Et–OH + SiOH → Et–O–Si + H2O. | (1) |
The weak bands in the same regions of curve 2 are not to be ascribed to ethoxy groups, but more probably due to traces of organic contamination. The dye molecule in principle has an N=N stretching mode: this is, however, intrinsically weak and at the most contributes to the weak band at 1388 cm–1 [33,34]. Similarly, the contribution from the CH2 and CH3 groups of the dye molecule is reckoned to be negligible on the basis of the spectrum in solution (not reported).
Both the narrow peak at 3736 cm–1, due to quasi-isolated silanols, and the broad band centered around 3500 cm–1, due to H-bonded silanols, are less intense in curve 1, in agreement with the fact that ethoxylation of the silica surface consumes silanols, according to reaction (1). No bands due to surfactant molecules are observed in the FTIR spectra, suggesting that extraction has been effective in both cases.
3.3 UV–visible characterization
In all cases, the change in dye content from A-type samples to B-type samples only brought about an increase in intensity of the UV–visible absorption. This suggests the absence of dye aggregation [8,11], in agreement with the low concentration of the dye in the system. As a consequence only data concerning B-type samples are illustrated.
Fig. 3 compares the UV–visible spectra of B-0 (curve 1), B-A and B-E (curves 2 and 3, respectively). Corresponding values of λmax are collected in Table 1. Spectra in Fig. 3 refer to samples outgassed at room temperature: absorption maxima in the presence of air are somewhat displaced, as absorption is sensitive to humidity (Table 1). A single nearly symmetric band between 400 and 600 nm is observed, due to the π–π* charge transfer electronic transition typical of the push–pull chromophore.
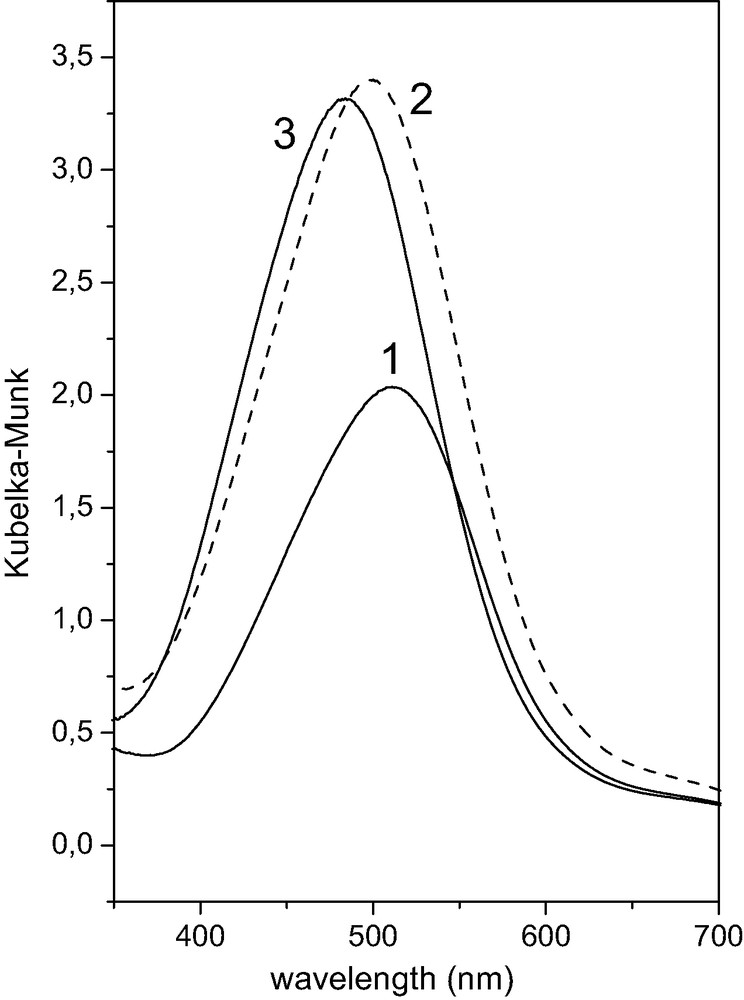
Visible spectra of B-0 (curve 1), B-A (curve 2) and B-E (curve 3) outgassed at room temperature.
λmax values for different DR1-containg HMS samples
Matrix | B-E | B-A | B-0 |
λmax(nm) in air | 480 | 499 | 513 |
λmax (nm) in vacuum (P < 10–3 mbar) | 475 | 492 | 511 |
Removal of the surfactant causes an intensification and a blue shift of the band, such latter effect being more marked for sample B-E (Δλ = 38 nm) than for B-A (Δλ = 26 nm).
Fig. 4 illustrates the behavior of sample B-E when exposed to HCl gas. Red-shift and narrowing of the π–π* band are due to the protonation of the azo group [36–39]. The band becomes also structured, probably because of a modification in the structure of vibrational sub-bands [38].

Visible spectra of B-E before (curve 1) and after (curve 2) exposing to gaseous HCl.
When the sample is exposed to ammonia or trimethylamine after exposure to HCl, the original spectrum is obtained, because the base strips back the proton from the azo group. This may be repeated several times, suggesting that dye molecules are readily accessible to HCl, NH3 and trimethylamine molecule in gas phase.
4 Discussion
Extraction in both ethanol and acetone does not cause sizeable leaching of the dye, so suggesting that DR1 is covalently attached to silica through the triethoxysilane functionality.
Ordered mesoporous silica is obtained, with pore size in the range expected for the synthesis procedure [21,25,26]. CBET values are lower than that observed for calcined and rehydroxylated silica surface and close to the average value expected for a silica surface partially functionalized by organic groups [40,41], i.e. with a lower polarizing effect: this suggest that the dye is actually lining the inner surface. The lower value obtained for B-E with respect to B-A is due to the further increase in the organic nature of the surface, to the ethoxilation occurring during the extraction. Also the lower values obtained for surface area and pore size with sample B-E are probably due to the presence of ethoxy group, in agreement with reports on organically modified mesoporous silicas [41,42].
The accessibility of dye molecules to HCl, NH3 and trimethylamine suggests that these are all available at the surface and not embedded into the walls of the silica matrix.
The intensification and the blue-shift of the absorption maximum in the visible region upon surfactant removal are consequence of a modification of the environment of the dye as also observed by Ganschow et al. [22] in the case of other dyes anchored to the inner surface of MCM-41. In particular the blue shift is due to a change in the polarity. This evidence confirms that dye molecules are mainly located inside the pore system.
Indeed, though far less marked than with other solvatochromic compounds [43–45], DR1 does show sensitivity to the polarity of the environment [46] and the π–π* charge-transfer electronic transition shifts towards lower wavelength when the polarity decreases.
For example, outgassing the samples at room temperature, which eliminates physisorbed water so decreasing the surface polarity, causes a blue shift with all B samples (Table 1).
The polarity of DR1 environment in HMS matrix results to decrease along the series: B-0, B-A and B-E. Two facts seem to operate: one is the removal of surfactant, the other the chemical nature of the solvent with which the extraction is carried out.
The former may be accounted for by assuming that the chromophore is affected by the polar heads of surrounding surfactant molecules more than by their lipophilic chains: notice that a certain amount of amine is likely to be protonated in the material [47]. This suggests that the chromophore lies in proximity of the micelles/silica interface, in contrast with its hydrophobic nature. It is worth of note that DR1 is poorly soluble in a ethanol–water solution whereas it is highly soluble in dodecilamine. In fact, if either DR1 or DR1UPTEOS are added to a n-dodecilamine–water–ethanol mixture with the same molar ratios used for the synthesis of sample B-0 and the mixture is first stirred and then maintained in static conditions, the separation of the dye/n-dodecilamine solution from the ethanol/water solution is observed.
The UV–visible spectrum of the reference sample R (not reported) resembles that of sample B-0. This suggests that, also in this case, the dye is located in the mesophase at the polar micelles/silica interface and that the reason of this is not the covalent bonding of the dye to the silica matrix.
Minoofar et al. [48] have proposed that the affinity of the dye (for example, hydrophobicity and hydrophilicity), named “philicity”, is a basic parameter controlling its location within the mesostructure. Our result suggests that the mechanism of this location is probably more complex.
The location of chromophore at the surfactant-silica interface may account for the increase in d100 value (from 3.5 nm in the DR1-free HMS to 3.9 and 4.1 nm in samples B and R, respectively), when considering the dye acting as a sort of “spacer” between the silica surface and the micelle. An analogous effect has been observed when comparing d100 values of MCM-41 silicas prepared in presence of different acids (HCl and HNO3) [49].
Though d100 values of sample B and R are very close, these data suggest that this “spacing” effect is smaller when the dye is covalently anchored to the silica than when it is simply entrapped.
Extraction of B samples leads to the removal of polar heads, thus giving rise to a less polar environment for the chromophore. This effect is more marked with ethanol because of the ethoxilation of the surface, which substitutes polar silanol groups for apolar alkylic groups on the surface. As a broad conclusion, the chromophore seems to be basically affected by the amine polar heads in B-0, by silanols in B-A, and by ethoxy groups in B-E.
From the chemical analysis and the BET surface area, an estimation of the amount of dye molecules per unitary surface area is possible, leading to 1 molecule per 66 nm2 for B-A, i.e. the ethoxy-free surface. Considering cylindrical pores of diameter 3.4 nm, this means that, on average, each molecule is hosted in a portion of pore of about 6.2 nm in length. On the basis of these geometric features and the visible spectra, it seems feasible to describe the dye molecules as separated and non interacting even in the more concentrated sample.
5 Conclusions
DR1 has been covalently anchored to the internal surface of HMS silica by means of a one-step synthesis. Dye molecules are probably induced to sit at the surfactant-silica interface during the mesophase organization and silica polymerization, and their optical properties are affected by the polar nature of this environment. This decreases upon removal of surfactant, because of the elimination of polar amine heads. The surface obtained by extraction with ethanol is less polar than that obtained by extraction in acetone, because of the ethoxilation occurring in the former case.
The dye is accessible to gases from the outer environment, as revealed by the reversible protonation observed upon exposing the material to HCl and ammonia or trimethylamine in gas phase.
Both the sensitivity of the chromophore to the environment polarity and its accessibility may be crucial factors for potential application of DR1-modified mesoporous silica in optics.
Finally, the accessibility of the dye to gases supports the use of the present approach to preparing systems for optical sensing application [1].