1 Introduction
Second-order nonlinear optical (NLO) polymeric materials have long been recognized as potential candidates for electro-optic applications [1]. The interest in these materials derives from their better processability and larger NLO responses than inorganic materials such as lithium niobate crystals (LiNbO3), currently used in electro-optical modulators.
In order to exhibit second-order optical effects, these materials must have π electrons, which delocalize in response to an applied electric field, resulting in spatial asymmetry. At the molecular level, the second-order NLO properties (molecular hyperpolarizability (β)) arise from non centrosymmetric π-conjugated charge transfer molecules containing both electron-acceptor and electron-donor groups connected by an electron-transmitting bridge (push–pull structure, characterized by a large dipole moment, μ) [2]. The bulk second-order NLO response originates typically from noncentrosymmentric alignment of NLO chromophores in poled polymers [2].
Quantum mechanical analysis based on a simple two-level model [3] and the bond order alternation (BOA) principle [4] have provided useful structure/property relationships for the design of chromophores with ever improving β values. It has been shown (Chart. 1) that very large nonlinearities can be achieved by combining heterocyclic conjugating units such as thiophene with tricyano-derivatized furan electron-acceptor [5], for example FTC chromophore I [6], or by employing extended polyene π-bridged systems with strong multicyano-containing heterocyclic electron-acceptor like CLD chromophore II [7].
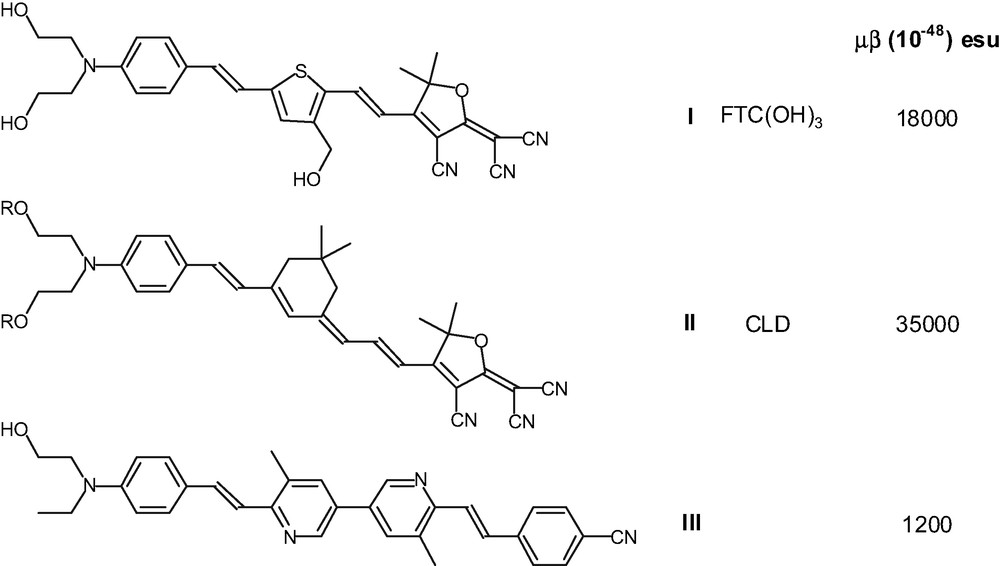
Structure and properties of some π-conjugated chromophores.
Compared to organic nonlinear optics, the field of organometallic nonlinear optics is relatively less explored, even if organometallics are potentially interesting candidates for NLO purposes [8]. Indeed, they show very strong absorption bands [metal-to-ligand charge transfer (MLCT) and ligand-to-metal charge transfer (LMCT)] in the UV–Vis region, which are related to high transition dipole moments and low transition energies. Among the metallocene systems that have been examined in detail from both experimental and theoritical perspectives, ferrocene-containing compounds have emerged [9]. Although the electron-donor ability of this group is moderate, comparable to p-methoxyphenyl group [9], it offers the possibility of the NLO properties redox switching as well as an easy introduction of chirality.
In previous papers, we described a series of organometallic push–pull chromophores [10] based on organometallic donors such as ferrocene [10a,e], thiophene conjugated spacers and either organic acceptors, such as benzonitrile [10b,d], or organometallic acceptors. Their syntheses relied on palladium-catalyzed cross-coupling reactions and conventional Wittig or Wittig–Horner reactions. We also described a novel class of NLO 6,6′-(disubstituted)-3,3′-bipyridine-based chromophores (such as chromophore III) [11]. The new large-scale and versatile strategy to access to these compounds is based on (i) the synthesis of a library of conjugated 6-substituted-3-bromopyridine building blocks, (ii) their transformation to 6-substituted-3-pyridylboronic ester building blocks, and (iii) the cross-coupling by the metal-catalyzed Suzuki reaction [12]. The main advantage of this synthetic pathway is the flexibility to combine ‘tailor-made’ building blocks, depending on the expected optical properties of the chromophore.
The present contribution reports on the synthesis of two new ferrocene-based chromophores IV and V, containing the same spacer and acceptor groups as chromophores I and III.
2 Results and discussion
The structures of the new π-conjugated organometallic chromophores (IV–V) are shown in Chart. 2.
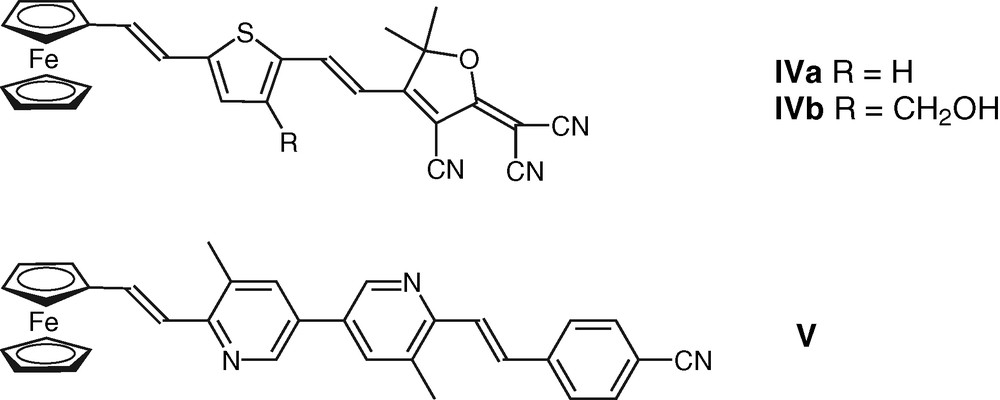
Structure of the newly-designed π-conjugated organometallic chromophores IV and V.
The synthesis of the thiophene-based chromophores IVa and IVb is based on (i) a stereoselective access to the alkenes (E)-4 and (E)-7 as described in Scheme 1, (ii) a Knoevenagel condensation with the tricyanofuran acceptor 10 (Scheme 2).

Preparation of thiophene-based building blocks 4 and 7.
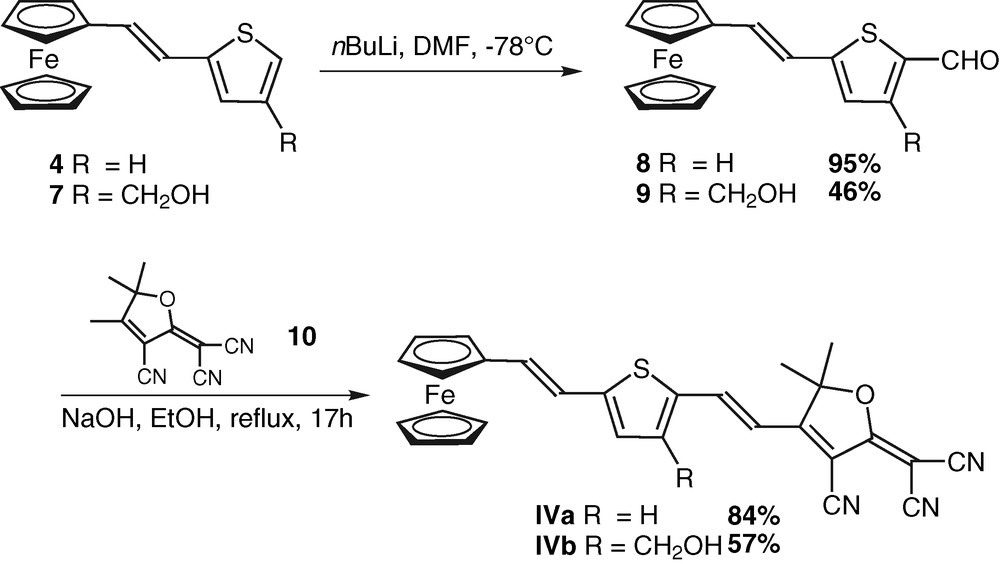
Obtention of chromophores IVa and IVb.
Building block 4 was obtained via a Wittig condensation [13] between the organometallic phosphonium 2, prepared following a described procedure [14], and commercially available thiophene carboxaldehyde (3). The alkene 4 was isolated as a 80:20 mixture of the two stereoisomers (E) and (Z), respectively. Their separation by chromatography was unsuccessful. However, isomerization of the minor (Z) product could be performed by heating the crude mixture in the presence of a catalytic amount of iodine, giving access to (E)-4 [13] in 67% yield. Concerning the preparation of building block 7, in which the thiophene ring is substituted by a hydroxymethyl side chain, a Wittig–Horner–Emmons condensation was chosen; indeed, this condensation is highly stereoselective. Starting material 4-bromothiophene-2-diethylphosphonate (5) was prepared as described in the literature [6]. Ferrocenecarboxaldehyde (1) was condensed with 5, in the presence of t-BuOK, giving the alkene (E)-6 in 78% yield after purification. No trace of the (Z) isomer was observed. 1H NMR of alcenes 4 and 6 clearly indicate the trans nature of the double-bond, with coupling constants for the ethylene bridge signals of 15.9 and 16.0 Hz respectively. The hydroxymethyl side chain was introduced on 6 via a metal-halogen exchange/formylation/reduction sequence on the fourth position of the thiophene ring, giving compound 7 with a 53% overall yield.
Subsequent formylation of the fifth position of the thiophene ring gave the aldehydes 8 [13] and 9 which were reacted in basic conditions with the tricyanofuran acceptor 10, prepared according to a described procedure [15], to afford the chromophores IVa and IVb in 84% and 57% yields respectively. Their spectroscopic data are consistent with the assigned structures1.
The synthetic approach to prepare chromophore V is based on the Suzuki coupling of a 6-substituted-3-bromopyridine and a 6-substituted-3-pyridylboronic ester building blocks (Scheme 3). To access to these key intermediates, two different routes were used, depending on the electron-donor or the electron-acceptor character of the end-substituent.
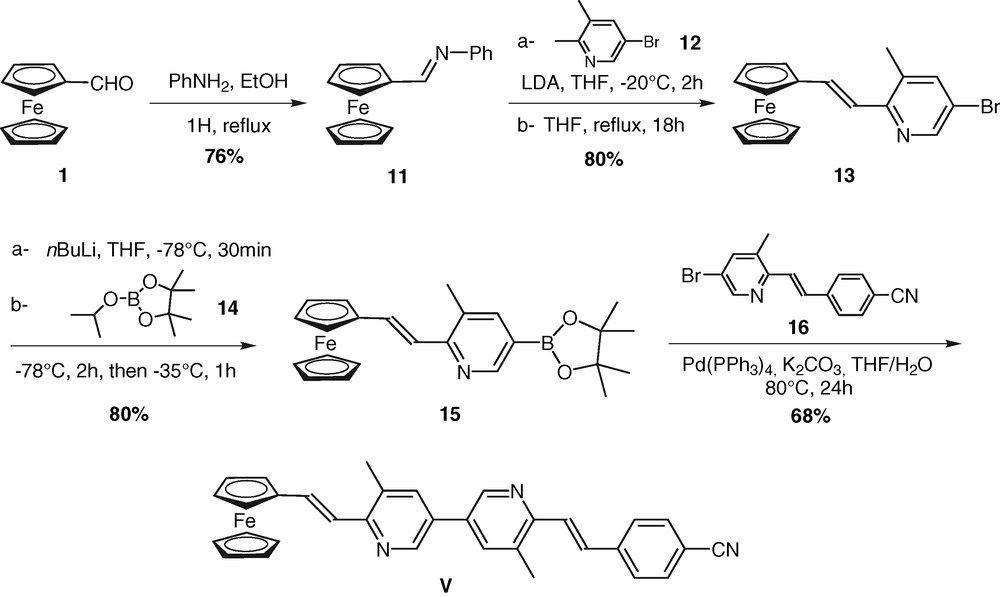
Preparation of chromophore V.
Regarding brominated compound 13, bearing an electron-rich aromatic ring, an adaptation of the Siegrist reaction was chosen [16]. The Siegrist method is a condensation between an aryl imine and the methyl group of an aromatic ring, in the presence of a base such as t-BuOK, allowing the stereoselective formation of trans-stilbene derivatives. First, condensation of the ferrocenecarboxaldehyde (1) with aniline in refluxing toluene afforded 11 in 76% yield after recrystallization in diethyl ether. Treatment of complex 11 with 12, prepared as described previously [11a,b], in the presence of LDA, instead of t-BuOK, in THF at –20 °C gave 13 in 80% yield on a multigram scale. As expected, examination of the 1H NMR spectra of the product showed that only the trans-isomer was obtained. Borylation of building block 13 was realized in 80% yield upon reaction with n-BuLi and commercially available 2-isopropoxy-4,4,5,5-tetramethyl-1,3,2-dioxaborolane 14. The brominated building block bearing an electron-withdrawing group 16 was obtained in a reasonable yield (31%) from the one-step Knoevenagel type condensation reaction in acidic medium of p-cyanobenzaldehyde with 12 [12b]. 16 was coupled with the borylated building block 15, under standard catalytic conditions, to give the new push–pull organometallic chromophore V in 68% yield. This compound has spectral data consistent with the assigned structure2.
3 Conclusions
In summary, two new organometallic π-conjugated push–pull chromophores were prepared. Their original design combine an organometallic donor group, the ferrocene, associated with an aromatic spacer, containing thiophene or 3,3′-bipyridine, and a tricyanofuran or a benzonitrile acceptor. This last acceptor group could be used in the future as a ligand to introduce a second organometallic entity, giving access to bimetallic chromophores.
The synthesis of chromophores IV was based on classical organic transformations such as Wittig or Wittig–Horner–Emmons condensations, and Knoevenagel reactions. An alkoxy side chain was introduced (IVb) in order to ultimately incorporate this chromophore covalently in a polymer matrix. The second chromophore, V, was obtained via a convergent Suzuki cross-coupling of a 6-(arylvinylene)-3-bromopyridine derivative and the corresponding pyridinyl boronic ester. These results show that this modular synthetic approach is very attractive in the field of optoelectronics, for example to synthesize other NLO (i) organic chromophores bearing more efficient donor/acceptor pairs, and (ii) organometallic chromophores to extend the series.
The evaluation of the electrochemical, and linear as well as NLO properties of these new families of organometallic chromophores are under investigation.
1 IVb: 1H NMR (200 MHz, (CD3)2CO)) δ (ppm): 8.36 (d, 1H, J = 15.7 Hz); 7.18 (s, 1H); 7.14 (d, 1H, J = 15.9 Hz); 7.01 (d, 1H, J = 15.9 Hz); 6.76 (d, 1H, J = 15.7 Hz); 4.86 (d, 2H, J = 5.4 Hz); 4.65 (m, 2H); 4.45 (m, 2H); 4.19 (s, 5H); 1.87 (s, 6H).
2 1H NMR (200 MHz, (CD3)2CO)) δ (ppm): 8.84 (m, 1H); 8.78 (m, 1H); 7.97–7.90 (m, 5H); 7.83–7.69 (m, 4H); 7.10 (d, 1H, J = 15.4 Hz); 4.67 (m, 2H); 4.38 (m, 2H); 4.17 (s, 5H); 2.60 (s, 3H); 2.48 (s, 3H).