1 Introduction
The large pore BEA zeolite is already used, or could be used, in various acid catalyzed reactions of refining (isobutane alkylation with n-butene [1], FCC as an additive to FAU zeolites [2]), of petrochemicals (aromatic alkylation such as cumene synthesis [3,4]), and of fine chemicals (Meerwein Pondorff Verley reduction of 4-tert butylcyclohexanone [5], anisole acetylation [6,7]). The great industrial interest of this zeolite can be related to the generally small crystallite size [8] and to its particular pore system and acidity.
BEA zeolite exhibits a three dimensional channel system with 12-membered ring apertures of 0.56 × 0.56 nm and 0.66 × 0.67 nm. Its framework structure is complex. Indeed, BEA zeolite is an intergrowth hybrid of two distinct structures named polymorphs A and B [9]. Unsatisfied linkages are present in the region connecting the two polymorphs inducing a stacking disorder with a high concentration of internal defects.
The location, nature and role of the active sites involved in catalytic reactions are still matter of debate. HBEA zeolite has at least three different types of acid sites. Brønsted sites usually attributed to the bridging hydroxyls and two types of Lewis sites assigned to extraframework aluminum species or to aluminum in defect positions. As demonstrated by spectroscopic studies (IR and NMR), the distribution of these sites and their properties are strongly dependent on the composition and structure of the as-synthesized zeolite as well as on the nature and severity of the post-synthesis treatment.
Many reports have discussed the transformation of tetrahedrally coordinated aluminum atoms of HBEA zeolite into octahedrally coordinated ones, which are framework Lewis sites. This was demonstrated through simple calcination of the ammonium form zeolite [10,11], water adsorption [12], and steaming [13,14]. The reverse transformation can occur in the presence of ammonia, methanol or pyridine [12], or during ionic exchange [10].
The infrared studies also evidence two main contributions in the hydroxyl stretching range of zeolites. The most intense band around 3740 cm–1 results from silanol groups whereas the band at 3610 cm–1 is usually assigned to bridging hydroxyl groups Si–OH–Al [15]. The other bands correspond to aluminic hydroxylated species with a Lewis acid character [16,17]. The IR signals around 3660–3680 cm–1 are usually assigned to aluminic extraframework species or partially hydrolyzed aluminic species, bound to the framework via one or two oxygen bonds [11,13,15]. The exact chemical nature of these sites is still a matter of debate. The HBEA zeolite IR spectrum presents an additional band in the hydroxyl region named by Kiricsi et al. [13] ‘Very High Frequency’ band since it is centered around 3780 cm–1. This band has only been observed in modified alumina [18], dealuminated ZSM5 [19,20], and HBEA [11,13,15–17,21] zeolites. It is very sensitive to the post-synthesis treatment [16,17,19]. Different assignments have been proposed for this band. In modified alumina, it is ascribed to OH groups coordinatively bonded with a single tetrahedral or octahedral aluminum cation [18]. Lago et al. [20] assigned it to partially hydrolyzed framework aluminum. According to Loeffler et al. [19], such a high frequency hydroxyl stretching vibration would correspond to isolated AlOOH species. Trombetta et al. [21] suggested that this band results from extraframework small alumina clusters. Kiricsi et al. [13] assigned this band to hydroxyl groups connected to aluminum, which is leaving the framework (transient state species). Vimont et al. [22] assigned it to tricoordinated aluminum atoms partly linked to the framework and showed that it is related to a framework vibration. The bands around 3660, 3680 and 3780 cm–1 exhibit a very strong Lewis acidity since they are able to retain pyridine at very high temperatures [16,17]. These bands are very sensitive to the post-synthesis treatment [17]. The band at 3680 cm–1 is exclusively observed for samples dealuminated by HCl or ammonium hexafluorosilicate. Moreover, pyridine adsorption studies followed by FTIR spectroscopy have shown the existence of two Lewis acid sites with different strength [17]. The weakest Lewis acid site, which was observed for samples treated by HCl or ammonium hexafluorosilicate, would be associated to extraframework Al (EFAL) species very close to protonic sites. This association of Lewis and Brønsted sites would be responsible for the aluminum ion formation which is observed at high pyridine temperature desorption [17].
Several authors have demonstrated the heterogeneity of the Brønsted site strength [15,16,23]. The presence of very strong Brønsted sites has also been noticed in several works [11,15,16,24]. The strongest Brønsted sites would be located in the well crystallized areas, the weakest in the areas of lower crystallinity [15]. Pazè et al. [23] have identified weak Brønsted sites as bridging hydroxyls perturbed by H-bond interaction with the zeolite framework. These sites give a broad IR band in the 3600–3200 cm–1 range.
The aim of the present study is to get a better understanding of the nature and the acid properties of the aluminic species present in HBEA zeolite. For that, a series of HBEA zeolites samples has been prepared by two dealumination treatments (steaming, acid leaching) of a parent sample (Cal) and the various aluminic species were identified and quantified. The effect of operating conditions (temperature, acid concentration, time of treatment) on dealumination kinetics, and the chemical nature and properties of aluminic species will also be discussed.
2 Experimental
2.1 Dealumination procedures
The parent sample (Cal) results from the calcination under dry air flow up to 550 °C of a NH4-BEA sample supplied by Zeolyst International (CP814E). Chemical and 29Si NMR analysis of the NH4-BEA sample shows total and framework Si/Al ratios of 12.5 and 15.5, respectively.
The steamed samples, St, were prepared by treatment of the sample Cal at 500, 600 or 700 °C under a water–air mixture flow (Pwater = 93.3 kPa) for one, three or 6 h.
The acid treatment, Ac, was carried out under stirring at temperatures of 30 and 100 °C, with a HCl concentration of 1 N and an acid solution to zeolite ratio of 10 cm3 g–1. After treatment, the zeolite samples were recovered by filtration, washed with a large amount of warm deionized water (70–80 °C) and then oven-dried at 120 °C for 24 h.
The samples will be called St or Ac according to the dealumination treatment followed by the treatment temperature in °C and the treatment time in hours.
2.2 Physicochemical characterization of the samples
The global Si/Al ratio of all the samples was determined from elemental analysis. The pore size distribution (micropores and mesopores) was obtained from nitrogen adsorption at 77 K. The apparent crystallinity was estimated by X-ray diffraction. Infrared spectra were recorded with a Nicolet Magna FTIR 550 spectrometer (resolution 2 cm–1). The position of the structure bands (450–1250 cm–1) and especially that of the asymmetric stretch vibration (νTOT) at 1080–1200 cm–1 was determined using KBr wafer containing 0.5 wt.% of the zeolite sample. For each samples, ten measurements have been done for different positions of the wafer. The concentration of Brønsted and Lewis sites were calculated from the integrated area, for a temperature of pyridine desorption of 150 °C, of the PyH+ and PyL bands at 1545 and 1450 cm–1, respectively. The experimental methods were described in detail in a previous papers [17,25].
3 Results and discussion
3.1 Effect of dealumination treatments on the zeolite composition
It is well known that both framework Al (FAL) atoms and EFAL species can be eliminated through acid leaching with consequently a decrease in the global Si/Al ratio whereas steaming causes essentially FAL extraction with deposits of the extracted species on the zeolite, hence without change in the global Si/Al ratio. Therefore, with both methods, it is essential to characterize the framework composition (degree of framework dealumination) as well as to estimate the amount of EFAL atoms. 29Si MAS NMR is often used to determine xAl, i.e. the atom fraction of Al in tetrahedral sites
Fig. 1 shows the effect of acid leaching on the frequency of this band and on the percentage of dealumination determined from elemental analysis data. During the first 10 min of acid treatment at 30 °C, there is a significant dealumination of the zeolite (53.5%) with practically no change in the frequency of the structure band. It can, therefore, be concluded that most of the Al atoms extracted from the zeolite were in extraframework positions. Afterwards the zeolite dealumination is much slower: 150–200 times slower than initially, and there is an increase in the frequency, which indicates a framework dealumination.
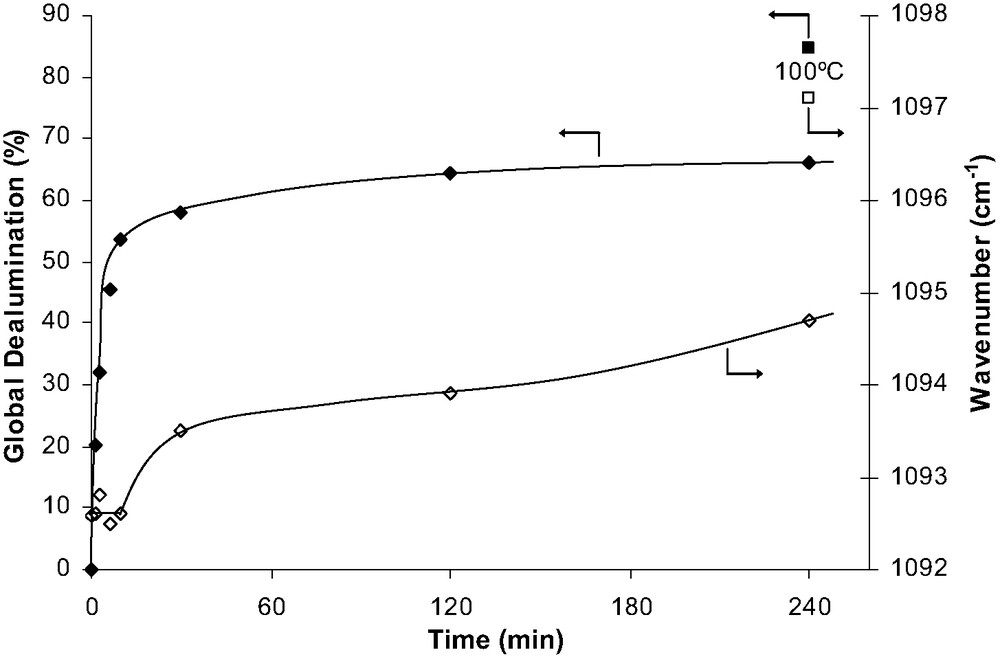
Percentage of global dealumination (■) and wavenumber of the asymmetric stretch vibration νa(TOT) (□) vs. treatment time for acid leached samples.
Steaming at 500 °C causes an increase in the structure band frequency, the effect of time being very close to the one observed with acid leaching at 30 °C. As could be expected, more severe conditions of steaming and acid leaching cause a more significant increase in the frequency hence in the framework dealumination.
In Fig. 2, the frequency of the structure band of acid leached samples was plotted as a function of x′Al, the total Al atom fraction in the zeolite
- • For x′Al between 0.074 (Cal sample) and 0.036, i.e. for short treatment times (t), the frequency remains constant indicating that there is no framework dealumination hence only a selective extraction of EFAL species.
- • For x′Al < 0.036, i.e. for t > 10 min, a linear relation can be obtained between the frequency and x′Al values, which suggests that there is essentially framework dealumination and that most likely xAl is practically equal to x′Al. In agreement with this proposal, the equation of the straight line is similar to the one obtained by Coutanceau et al. [26] between the frequency value and the atom fraction of Al in tetrahedral site (xAl).
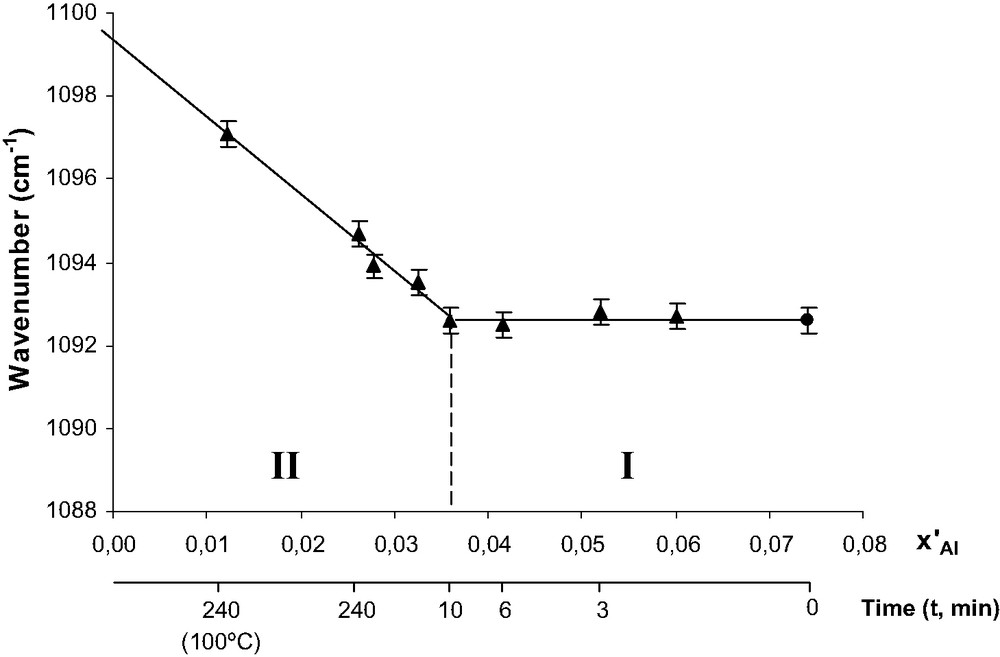
Frequency of the most intense TOT band vs. x′Al the atom fraction of Al in the HBEA samples. The duration of the acid treatment (t, min) is also indicated in abscissa. Reproduced by permission of the Royal Society of Chemistry [25].
This clear division of Fig. 2 in two domains, one essentially with EFAL extraction at short leaching times (domain I), the other with essentially FAL extraction for long leaching times (domain II) indicates a very large difference in rate between these two processes (two orders of magnitude). Furthermore, the linear correlation between νa(TOT) and xAl can be used to determine the xAl value corresponding to the samples dealuminated by steaming hence the percentage of framework dealumination of the steamed samples. The values of x′Al, xAl, and of the percentage of dealumination (total and framework) are reported in Table 1 for all the acid treated and steamed samples. As it could be expected, these percentages increase with both steaming time and temperature.
Composition of BEA samples and percentage of total and framework dealumination
Sample | x′Al | xAl | % dealumination total | % dealumination framework |
Cal | 0.074 | 0.037 | – | – |
Ac30(0.025) | 0.060 | 0.037 | 20 | 0.2 |
Ac30(0.05) | 0.052 | 0.035 | 32 | 3.3 |
Ac30(0.1) | 0.042 | 0.037 | 46 | 0.0 |
Ac30(0.17) | 0.036 | 0.036 | 53 | 2.2 |
Ac30(0.5) | 0.032 | 0.032 | 58 | 11 |
Ac30(2) | 0.028 | 0.028 | 64 | 25 |
Ac30(4) | 0.026 | 0.026 | 66 | 29 |
Ac100(4) | 0.012 | 0.012 | 85 | 68 |
St500(1) | 0.074 | 0.031 | – | 16 |
St500(3) | 0.074 | 0.027 | – | 27 |
St500(6) | 0.074 | 0.024 | – | 34 |
St600(3) | 0.074 | 0.019 | – | 47 |
St700(3) | 0.074 | 0.010 | – | 72 |
3.2 Effect of dealumination treatments on the Brønsted and Lewis acidities
The composition of the unit cell formula as well as the concentrations of FAL, EFAL atoms, Brønsted and Lewis sites are reported in Table 2. The effect of acid leaching at 30 and 100 °C is shown in Fig. 3A, in which the percentages of elimination of Brønsted sites are plotted as a function of time. For both temperatures, the elimination of Lewis sites is faster than that of Brønsted sites and for both acid sites the elimination rate decreases with increasing time. Thus, for acid leaching at 30 °C, there is, in the first 3 min, elimination of 60% of the Lewis sites and of less than 10% of the Brønsted sites. This fast initial decrease in Lewis acidity can be related with the fast dissolution of EFAL species. Furthermore, the slower elimination of protonic sites suggests a framework dealumination. The degree of elimination of both Brønsted and Lewis sites increases significantly with temperature. Thus, after 4 h of acid leaching, 85% of the Lewis sites and 40% of the Brønsted sites are eliminated at 30 °C whereas at 100 °C practically all the Lewis sites (98.5%) and 73% of the protonic sites are eliminated.
Composition of the unit cell formula of the acid treated samples, concentration of FAL, EFAL Al atoms, and of Brønsted and Lewis sites (μmol g–1)
Sample | Unit cell composition | FAL | EFAL | Brønsted sites | Lewis sites |
Cal | H2.34Al2.34Si61.66O128.00 (2.59EFAL) | 590 | 650 | 315 | 340 |
Ac30(0.025) | H2.34Al2.34Si61.66O128.00 (1.60EFAL) | 596 | 406 | 290 | 190 |
Ac30(0.05) | H2.27Al2.27Si61.66(OH)0.28O127.72 (1.12EFAL) | 581 | 288 | 285 | 134 |
Ac30(0.1) | H2.37Al2.37Si61.66O128.00 (0.31EFAL) | 614 | 80 | 276 | 130 |
Ac30(0.17) | H2.29Al2.29Si61.66(OH)0.20O127.8 | 596 | 0 | 273 | 119 |
Ac30(0.5) | H2.07Al2.07Si61.66(OH)1.08O126.92 | 539 | 0 | 255 | 103 |
Ac30(2) | H1.76Al1.76Si61.66(OH)2.32O125.68 | 460 | 0 | 207 | 69 |
Ac30(4) | H1.67Al1.67Si61.66(OH)2.68O125.32 | 435 | 0 | 188 | 52 |
Ac100(4) | H0.75Al0.75Si61.66(OH)6.36O121.64 | 198 | 0 | 84 | 8 |
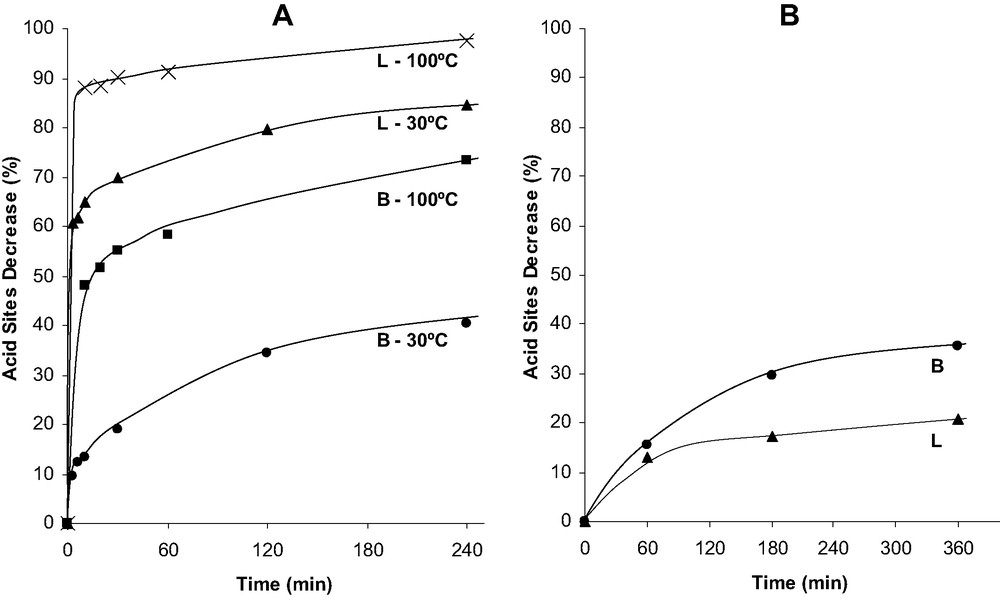
Brønsted (B) and Lewis (L) acid site evolution vs. dealumination time for acid leached samples at 30 and 100 °C (A) and steamed samples at 500 °C (B).
Steaming causes a more limited decrease in both Lewis and Brønsted acid site concentrations (Fig. 3B). Whereas the decrease in Brønsted acidity can be related to framework dealumination, the one in Lewis acidity indicates that the creation of new Lewis EFAL species is slower than the condensation of EFAL species and the elimination of defect sites.
Fig. 4 shows the change in the concentration of Brønsted and Lewis acid sites as a function of x′Al. For the Brønsted acidity of acid leached samples, the figure can be divided into the two domains previously defined in Fig. 2. For domain I (0.036 < x′Al < 0.074), which corresponds to the extraction of EFAL species, only a small decrease in the Brønsted acidity can be observed; for domain II, the decrease in protonic site concentration is more significant and moreover proportional to x′Al. Fig. 5A shows that for all the dealuminated samples (with and without EFAL species), the concentration of Brønsted acid sites is proportional to the framework aluminum content. However, the slope of the straight line is much lower (∼0.50) than the expected value of 1. This lower value cannot be related to a partial blockage of the access of pyridine molecules to the protonic sites or to the neutralization of these sites by cationic EFAL species. Moreover, it cannot be due to a too weak acidity of part of the bridging OH groups, at the desorption temperature (150 °C) all these OH groups interact with pyridine molecules.
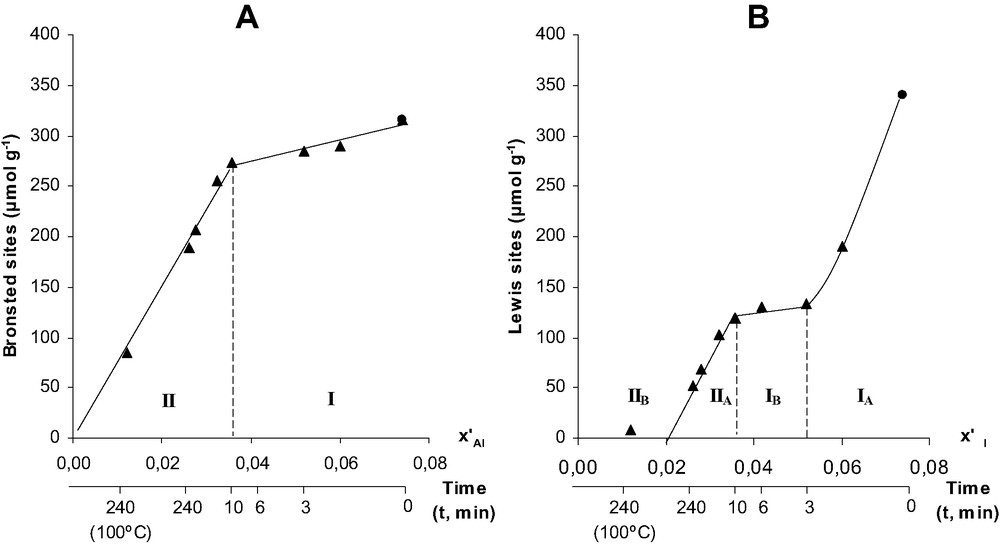
Concentration of Brønsted (A) and Lewis (B) acid sites vs. x′Al the atom fraction of Al in the HBEA sample treated by HCl. The duration of the acid treatment (min) is also indicated in abscissa. Reproduced by permission of the Royal Society of Chemistry [25].
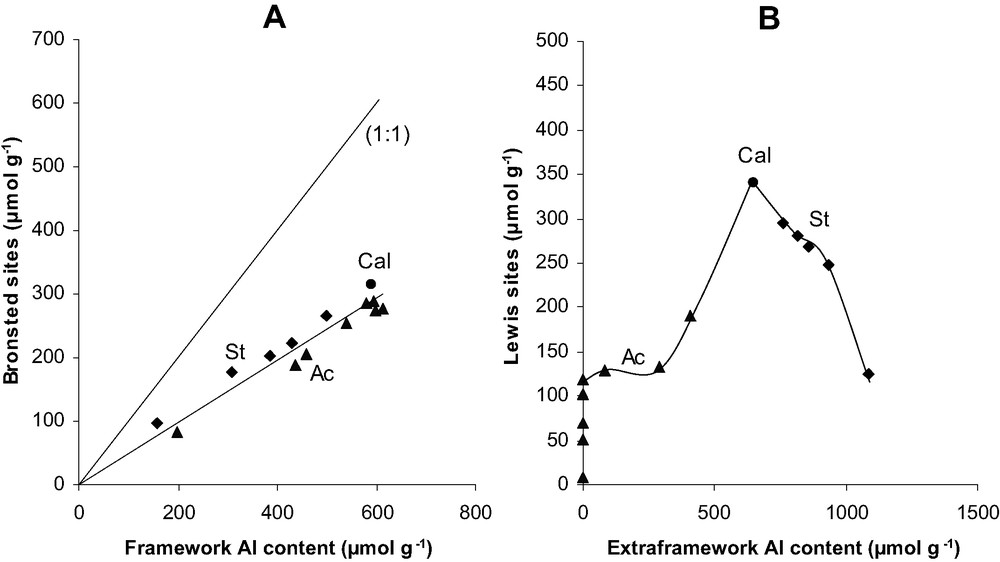
Evolution of the Brønsted site concentration vs. framework aluminum content (A) and Lewis site concentration vs. extraframework aluminum content (B) for acid leached and steamed samples.
The change in the Lewis acidity with x′Al is more complex (Fig. 4B). Domain Ia (0.052 < x′Al < 0.074) corresponds to the first 3 min of acid leaching. A significant decrease in the Lewis site concentrations is observed (340–134 μmol g–1). This decrease can be related to a fast extraction of EFAL species. However, the decrease in EFAL atom concentration is equal to 362 μmol g–1, i.e. only 1.7 times higher. This means that essentially monomeric or dimeric EFAL species are eliminated at very short times. In domain Ib (0.036 < x′Al < 0.052), the remaining EFAL species (288 μmol g–1) are extracted. This extraction is 20 times higher than the Lewis acid site elimination. This shows that the extracted EFAL species are mainly polymeric with a very low Lewis acidity per Al atom. For leaching times longer than 10 min (x′Al < 0.036), practically no EFAL species are present in the zeolite samples. Therefore, the residual Lewis acidity can be related to structure defects only. In domain IIa (0.02 < x′Al < 0.036), the decrease in Lewis acidity is practically proportional to x′Al (Fig. 4B) as it was the case for the Brønsted acidity (Fig. 4A). This decrease corresponds to an elimination of 278 μmol g–1 of Al, of 120 μmol g–1 of Lewis (Table 2) and of 130 μmol g–1 of Brønsted sites (Fig. 4A), i.e. the elimination of one Al atom has as a consequence the elimination of roughly one acidic site, Lewis or Brønsted. This observation could explain the low value of the ratio between the concentrations of Brønsted sites and of extracted FAL atoms: part of the extracted FAL atoms are not Brønsted but Lewis acidic sites corresponding to framework defects probably tricoordinated Al atoms. The residual Lewis acidity becomes close to zero for x′Al (or xAl) values of 0.02 (estimated by extrapolation of the curve in Fig. 4B).
Steaming does not cause an increase but a decrease in the Lewis acidic site concentration (Fig. 5B). This decrease is most likely related to an increase in the degree of polymerization of the EFAL species under the severe steaming conditions hence to a decrease of the Lewis acidity per Al atom.
3.3 Distribution and rate of extraction of the various aluminic species of the Cal sample
The distribution of the various aluminic species in the Cal sample, i.e. FAL species of bridging OH groups, mono + dimeric and polymeric EFAL species and framework defects can be estimated from the total and framework composition and the Lewis acid site concentration of the acid treated samples and from the observations made in Section 3.2. The Cal sample contains 590 μmol g–1 of FAL constituted partly by defects (tricoordinated FAL atoms): 120 μmol g–1, partly by the Al atoms of the bridging OH groups: 470 μmol g–1. This later concentration is 1.5 times greater than the concentration of protonic sites: 315 μmol g–1. This difference, which is too large to result from imprecision in the determination of the extinction coefficient is also difficult to explain by a partial neutralization of the protonic sites by cationic EFAL species or by a too weak acidity of these sites. In addition, the Cal sample contains 650 μmol g–1 of EFAL atoms, 55% of them being mono or dimeric (360 μmol g–1), 45% polymeric (290 μmol g–1).
The dissolution rates of these various Al species were estimated from the slopes of the tangent at time zero to the curves in Figs. 1 and 3. Mono or dimeric EFAL species are the most rapidly eliminated (8 mmol h–1 g–1); polymeric EFAL species are at least four times more slowly extracted. The dissolution rate of FAL species (bridging hydroxyl groups and framework defects) is much slower (initial rate: 0.1 mmol h–1 g–1) and decreases with time. Moreover, there is apparently no large difference in the elimination rate of these two types of FAL species. Thus, the concentration of defects passed from 119 to 69 (–50) μmol g–1 from 10 to 120 min when the one of the protonic sites (hence bridging hydroxyl groups) passes from 273 to 207 (–66) μmol g–1 (Table 2).
3.4 Effect of dealumination treatments on the hydroxyl bands
The IR spectra of the parent sample (Cal) and of some steamed and acid leached samples are presented in Fig. 6. Five OH massifs appear in the Cal spectrum. The hydroxyl band assignment is reported on Table 3.
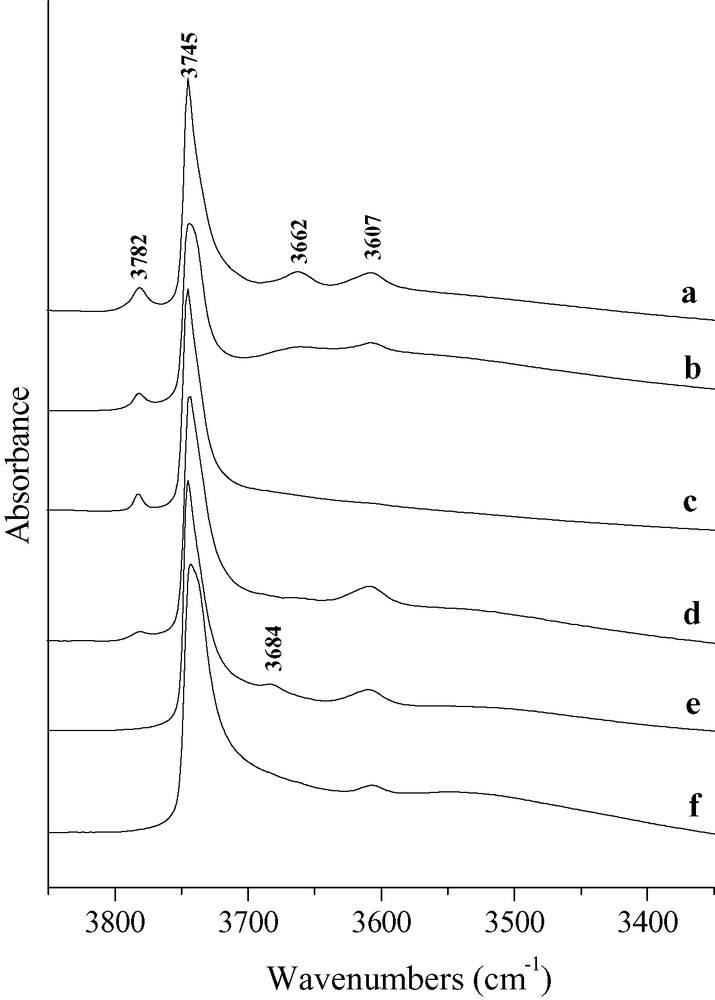
Infrared spectra of the hydroxyl region of activated samples Cal (a), St500(3) (b), St700(3) (c), Ac30(0.5) (d), Ac30(2) (e), Ac100(4) (f).
Hydroxyl band assignment for the sample Cal
IR hydroxyl band frequencies (cm–1) | Assignment | References |
3746–3744 | Amorphous silica or external surface silica at extraframework silica alumina debris | [15] |
3738–3730 | Internal silanols and silanols | [15] |
3607 | Bridging hydroxyl groups | |
3662 | AlOH groups, EFAL species | [15,19,21] |
3782 | Tricoordinated Al atoms linked to the framework by two O atoms | [22] |
3700–3300 | Hydrogen bonded OH groups | [10,19,24,27–29] |
3.4.1 Silanol bands
Acid leaching causes an increase in the band area of the silanol massif, which is roughly proportional to x′Al: this area passes from 7.6 to 11.9 cm–1 from the Cal sample to the Ac100(4) sample. Furthermore, the silanol massif becomes more asymmetric to lower wavenumber with dealumination, which indicates an upper contribution of the internal silanol component. Moreover, the large band between 3600 and 3300 cm–1 slightly increases with the severity of the acid treatment (Fig. 6), which can be assigned to the formation of silanol nests by acid dealumination of the framework:
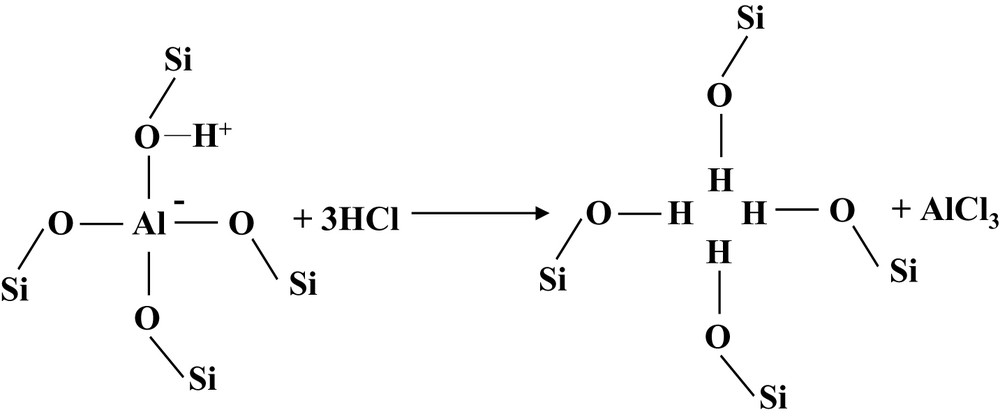
Steaming causes a small positive effect on the intensity of the silanol massif centered at 3745 cm–1 and on the wideness of the band. Moreover it causes a decrease in the intensity of the large band at 3600–3300 cm–1 (Fig. 6).
Fig. 7 shows on the example of the Cal sample that only a minor part of the silanol massif is affected by pyridine adsorption. The apparent acidity of the silanols absorbing at 3747 cm–1, which was already observed for HY zeolites [28] was explained by an indirect perturbation due to pyridine coordinated to a neighboring Lewis acid site. However, no internal silanols of HY zeolite interact with pyridine at 150 °C as it is the case with HBEA (band at 3737 cm–1).
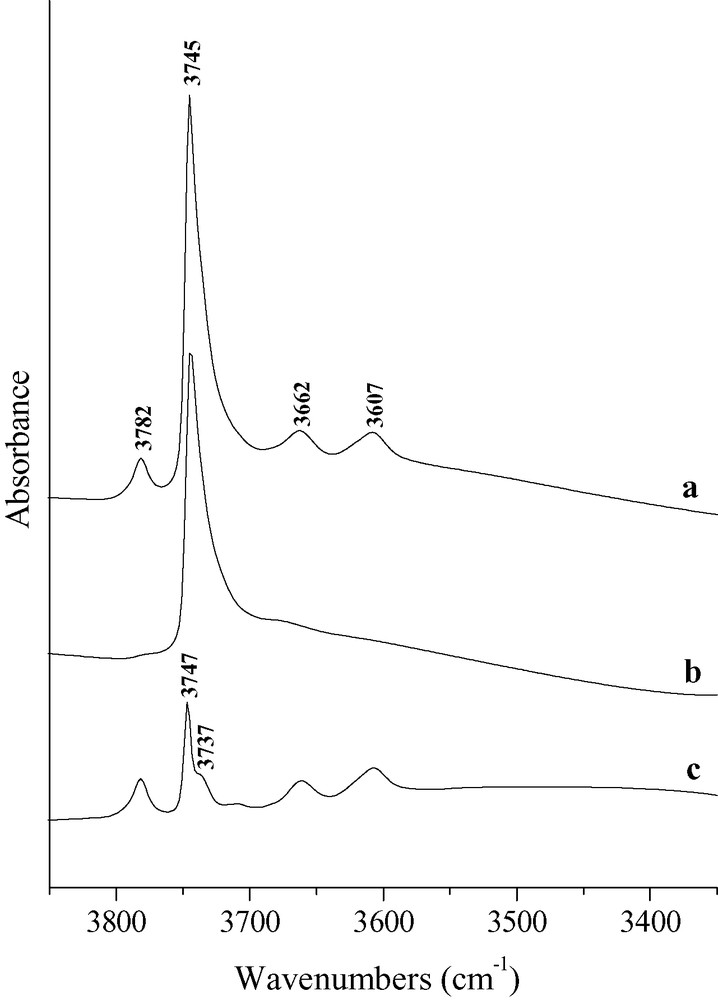
Infrared spectra of sample Cal after activation (a) and after pyridine adsorption and desorption at 150 °C (b) and the difference spectrum c = a – b.
3.4.2 Other hydroxylated species
Acid leaching at 30 °C causes a significant decrease in the intensity of the bands at 3782 and 3660 cm–1 (Fig. 6). Furthermore, there is at short time (3 min) a slight increase in the intensity of the band at 3607 cm–1 corresponding to bridging OH groups followed by a decrease at long time. Acid leaching causes also the appearance of an additional band at 3680 cm–1. This band was previously ascribed either to small neutral or cationic EFAL clusters [11] or to framework SiOH groups at defect sites [30,31]. As the band disappears after pyridine adsorption at 150 °C, the first proposal seems most likely. The effect of steaming is different: slight decrease with the severity of steaming in the intensity of the band at 3782 cm–1, significant decrease in those of the bands at 3660 and 3607 cm–1, and no appearance of the band at 3680 cm–1. The decrease in the intensity of the bands at 3607 and 3660 cm–1 can be related to framework dealumination and EFAL species oligomerization respectively. Furthermore, the low sensitivity to steaming of the band at 3782 cm–1 indicates that both the bonding of the corresponding partially coordinated Al atoms to the framework and the OH bond are very resistant. This observation is in contradiction with the usual description of the tricoordinated Al species as a transient state between framework aluminum atoms and extraframework species [13].
The intensity of the OH bands at 3782, 3660, 3607 and 3680 cm–1 were plotted in Fig. 8 as a function of x′Al for acid leached samples. It should be noted that all the bands disappear completely after pyridine adsorption–desorption at 150 °C, hence should correspond to acidic Brønsted OH groups or hydroxylated Lewis species. The intensity of the 3782 cm–1 band decreases very strongly at short leaching times: after three and 30 min, it is 1.7 and 3.8 times lower than on the Cal sample. This significant initial decrease, simultaneous to the elimination of monomeric and dimeric EFAL species suggests that most of the species responsible for this band could be in extraframework position. This proposal is in accordance with Trombetta et al. [21] who have assigned this band to small extraframework clusters of alumina. It could also explain the decrease in the intensity of this band with the severity of steaming hence with the degree of polymerization of EFAL species. Moreover, this band does not appear in the Ac30(2) sample, which however, contains non EFAL Lewis sites ascribed above to FAL defects.
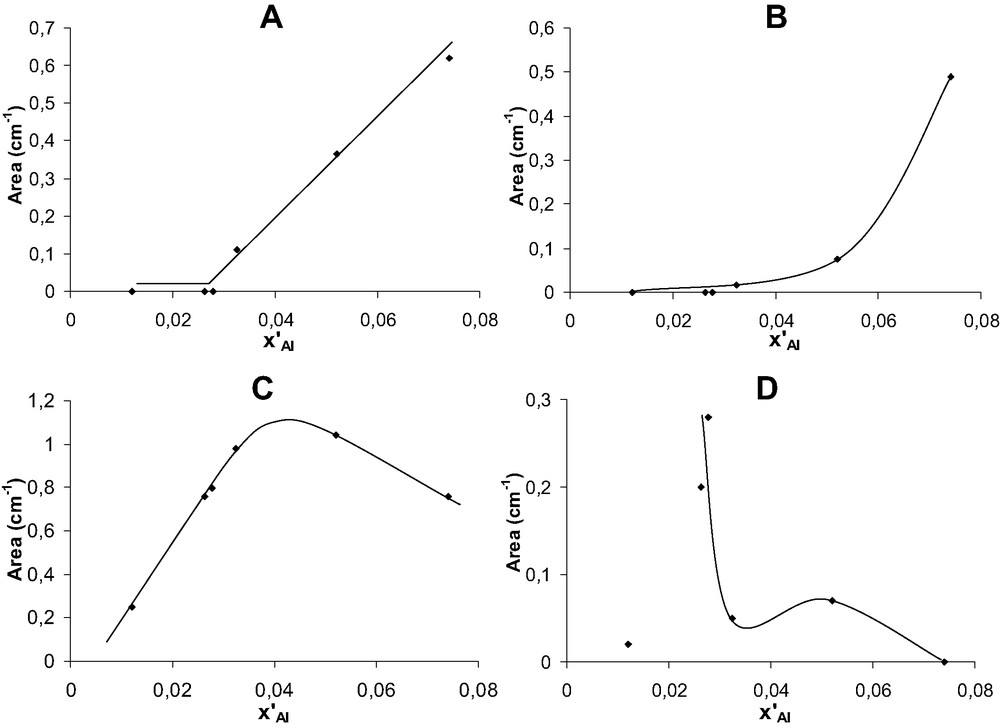
Area of the OH bands at 3782 (A), 3660 (B), 3607 (C) and 3680 cm–1 (D) vs. x′Al for acid leached samples.
The initial decrease in the 3660 cm–1 band is still more pronounced: its intensity is 3.5 times lower after 3 min leaching hence similar to the decrease in the amount of the monomeric or dimeric EFAL species. Afterwards, the decrease in the band is much slower (Fig. 8B), hence can be related to the elimination of polymeric EFAL species. The attribution of this band to hydroxylated EFAL species is in agreement with the literature (Table 3).
The intensity of the band at 3680 cm–1 not observed on the Cal and steamed samples increases with leaching time (Fig. 8D). This increase seems to be related to the disappearance of framework defect Lewis sites: indeed it is particularly pronounced for x′Al passing from 0.032 to 0.026 (domain IIa in Fig. 4B).
The intensity of the bridging hydroxyl band at 3607 cm–1 of acid leached samples passes through a maximum for an x′Al value between 0.04 and 0.05 (Fig. 8c), i.e. the elimination of EFAL species especially the monomeric ones, which occurs at short leaching times seems to be accompanied by the ‘creation’ of bridging OH groups. This could be explained by the presence of EFAL species in cationic positions, their exchange by the protons of HCl liberating the corresponding OH groups. However, it should be reminded that no protonic sites able to retain pyridine adsorbed at 150 °C appear at short leaching times (Fig. 4A). For x′Al ≤ 0.032 (leaching times ≥ 0.5 h), i.e. when only FAL atoms are eliminated from the zeolite, the intensity of the 3607 cm–1 band is proportional to x′Al (or xAl), as it was the case for the protonic acid sites concentration (Fig. 8c). Furthermore steaming causes a significant decrease in the intensity of the 3607 cm–1 band, which can be related at least for a part to the framework dealumination.
The intensity of the bridging OH band of zeolites should be proportional to xAl, the Al atom fraction in tetrahedral sites. Fig. 9 shows that whereas it is roughly the case for the acid leached samples, values of the 3607 cm–1 band intensity lower than expected are found for the calcined and steamed samples. The difference can be very significant. Thus for the St700(3) sample, the band intensity (I) is only 15% of the one expected from the linear correlation between I and xAl. This difference between observed and expected intensities is much too large to result from imprecision in the determination of xAl. It is most likely related to the presence of large amounts of defects and of monomeric EFAL species (being Lewis sites and hydroxylated species), which would perturb the neighboring of bridging hydroxyls groups.
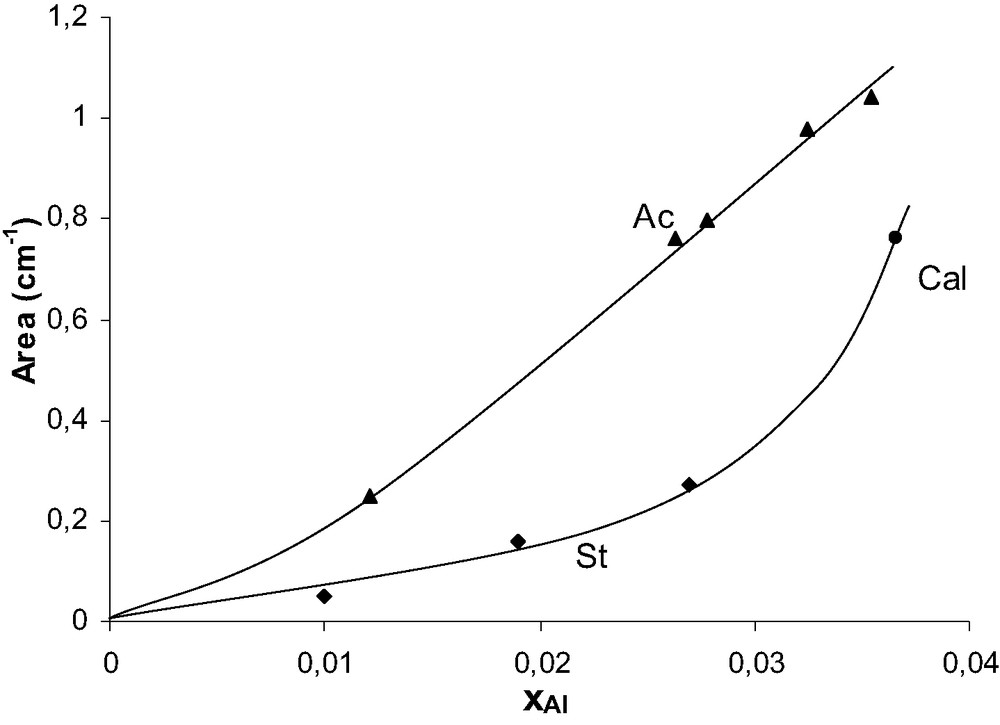
Area of the bridging OH band (at 3607 cm–1) vs. xAl for acid leached and steamed samples.
In the 1400–1700 cm–1 region, the bands corresponding to pyridinium ions (1637 and 1545 cm–1) and pyridine molecules coordinated to Lewis acid sites (1622 and 1456 cm–1) can be observed with all the samples [17]. A new set of bands at 1603 and 1446 cm–1 was found for acid leached samples. These bands are not visible for the calcined (Cal) and steamed samples. These bands are much more resistant to increased desorption temperature than typical H-bonded or alkaline bonded pyridine, hence should correspond to pyridine coordinated on a second type of Lewis site. The intensity of the band at 1446 cm–1 (called L2) was plotted in Fig. 10 as a function of x′Al. The shape of the curve is quite similar to that of the 3680 cm–1 (Fig. 8d). This band could be ascribed to EFAL clusters resulting from the extraction of Lewis framework Al defects.
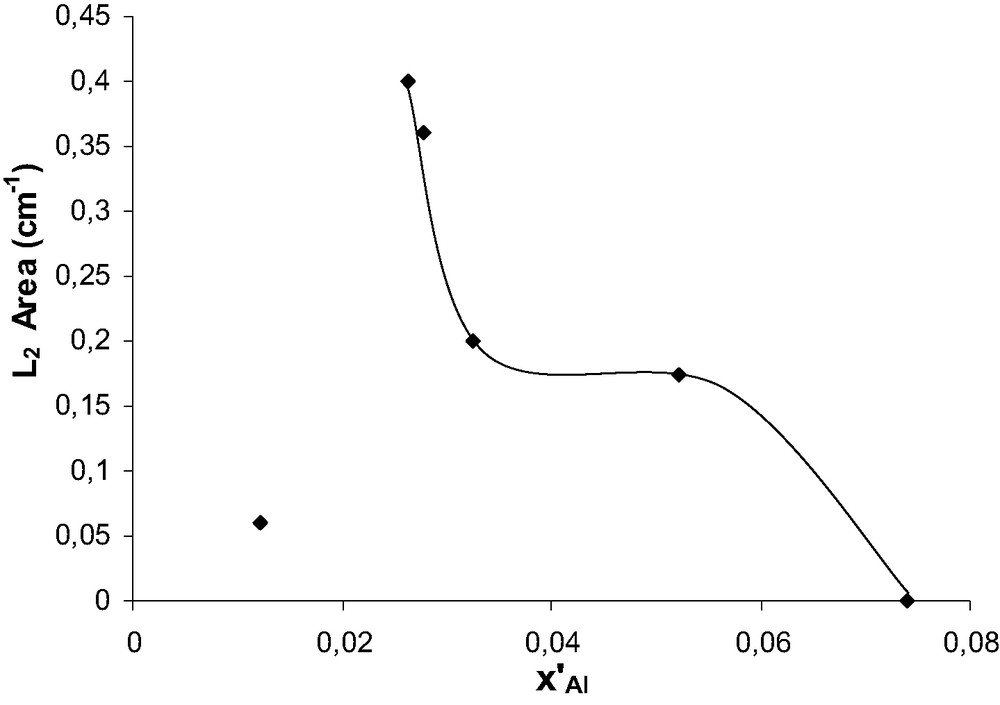
Evolution of L2 band vs. x′Al for acid-leached samples.
4 Conclusions
The following main conclusions can be drawn from this study of dealumination by acid treatment and by steaming of a HBEA zeolite sample with a total Si/Al ratio of 12.5 are:
- • Treatment of HBEA with 1 N HCl solution at 30 °C causes a rapid dissolution of the EFAL species and a slow dissolution of the FAL atoms. The dissolution rate of monomeric EFAL species was found four times higher than of the polymeric species and 80 times higher than that of FAL species.
- • A semi quantitative distribution of the various Al species of the HBEA sample can be established from the total and framework composition of the acid treated samples and from their concentration in Lewis and Brønsted acidic sites [25]. Thus, the parent sample was demonstrated to contain 650 μmol g–1 of EFAL species, 55% of them corresponding to monomeric or dimeric species, 45% to polymeric species, and 590 μmol g–1 of FAL including 120 μmol g–1 of framework defects and 470 μmol g–1 of Al belonging to bridging hydroxyl groups. This original method of characterization of the Al species of BEA zeolites should be particularly useful for specifying the catalytic role of each of these species.
- • The nature of Al species responsible for the various hydroxyl IR bands can be specified from the effect of the acid treatment and steaming on their intensities:
- ○ the band at 3607 cm–1 corresponds to bridging OH groups; however, on the parent and steamed samples, large part of these hydroxyl groups vibrate at lower wavenumber owing to interaction with other hydroxyl groups especially those of defects and monomeric EFAL species;
- ○ the band at 3660 cm–1 is clearly ascribed to monomeric and polymeric hydroxylated EFAL species with a Lewis acidity;
- ○ the band at 3780 cm–1 seems to be related both to monomeric EFAL species and to framework defects with Lewis acidity;
- ○ the band at 3680 cm–1, which appears only on acid treated samples can be ascribed to hydroxylated EFAL clusters resulting from the extraction of Lewis framework defects.
Acknowledgements
The authors wish to thank the financial support from Fundação para a Ciência e Tecnologia (POCTI/EQU/42622/2001) and the fellowship GRICES/ÉGIDE for the support given (mobility resources). J.P. Marques is grateful for the PhD grant from Fundação para a Ciência e a Tecnologia (SFRH/BD/3310/2000).
Vous devez vous connecter pour continuer.
S'authentifier