1 Introduction
Polyoxometallates (POM) are oxygen-bridged metal clusters well-known for their exquisite redox chemistry. As early as 1916 Ridle reported on the reversible photochemical action of polyoxometalates [1]. Since then, many studies disclosured that these compounds exhibit a fascinating redox chemistry in a way that: a) they can be reduced, keeping their structure intact, via a plethora of ways, i.e. photochemically [2–6], radiolytically [7], electrolytically [8] or by a variety of chemical reducing reagents [9] and b) they can reversibly attribute their excess electrons to a variety of inorganic and organic molecules [10]. The best-known, typical categories and structures of POM are pictured in Fig. 1.
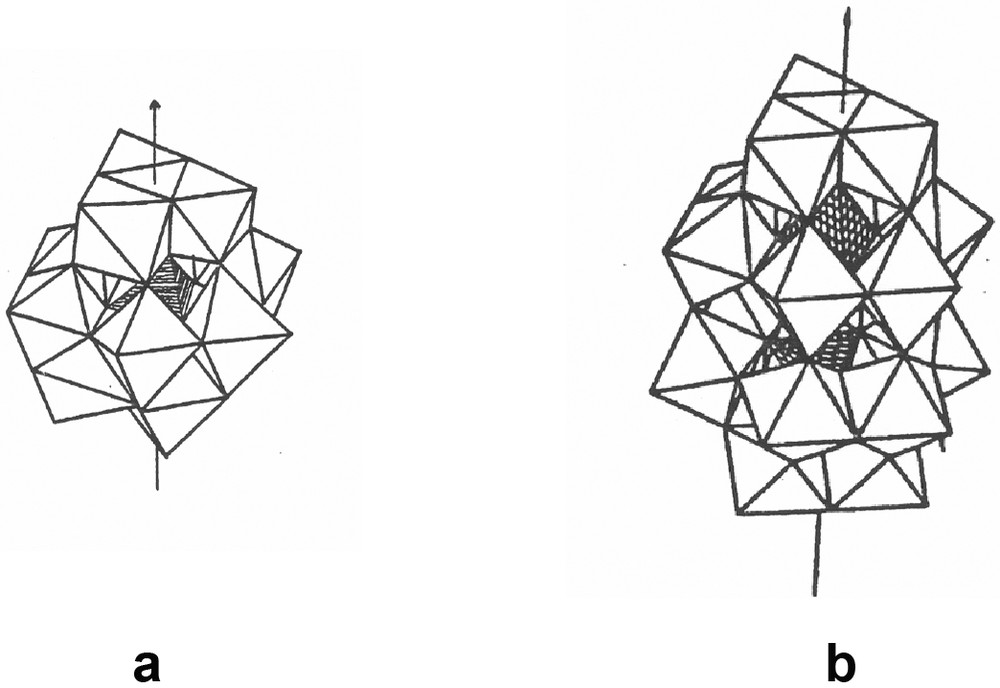
Characteristic structures of POM. (a) XW12O40n–, Keggin structure. They are composed of MO6 octahedra sharing corners and edges. The heteroatom X = P, Si or H2 is within the central (shaded) tetrahedron XO4. (b) P2Mo18O626–, Wells–Dawson structure, comes from the molybdate Keggin ion by removing three MoO6 octahedra and joining the two 9-molybdo half units.
On the other hand, metal ions are also excellent electron reservoirs as they exhibit variable oxidation states and the possibility of multiple reductions. However, despite the fascinating redox chemistry of both polyoxometalate ions and metal ions (for the corresponding reduction potentials see Fig. 2), no review concerning electron transfer processes between these two species has been presented. Since, besides our recent work, very little and early work has delved into this topic, we summarize and comment our latest results on redox interactions between POM and metal ions, focusing on the photocatalytic reduction of metal ions in the presence of polyoxometalates and present the perspectives of these studies in recovery of metals or synthesis of colloidal metal nanoparticles.
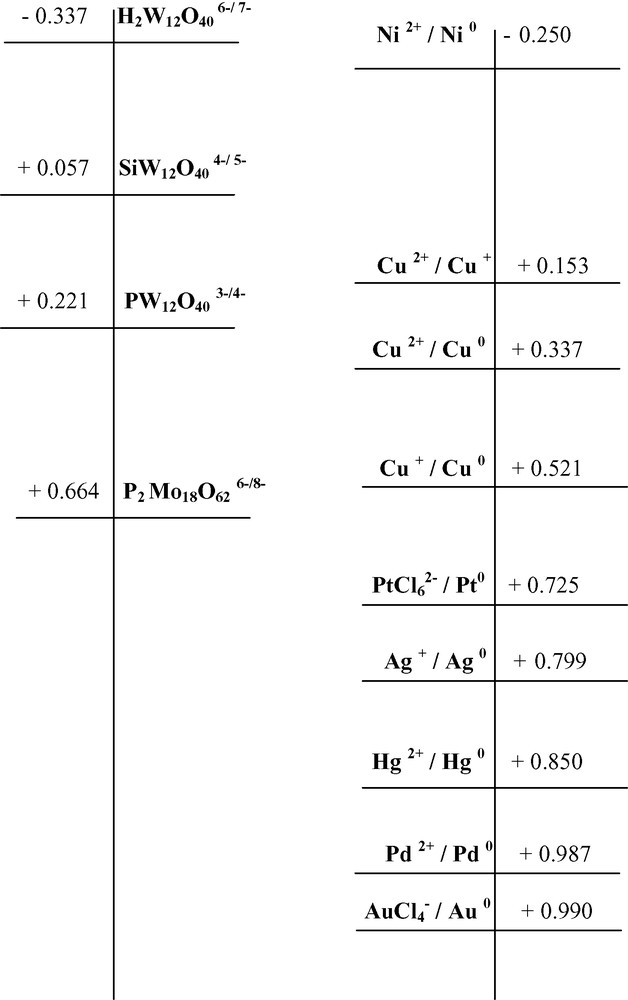
Redox potentials of polyoxometalate anions and metal ions (volts vs. NHE).
1.1 Photocatalytic reduction of metals by POM
As early as 1956, Chalkley had the inspiration to use photochemically produced, one-electron-reduced Keggin tungstophosphate, PW12O404–, to reduce and precipitate silver ions to metallic silver [11]:
(1) |
This process, firstly used in titration of the reduced POM in order to evaluate the excess negative form of the reduced species, was finally advanced to a patented photography process. As a resonance to Chalkley's pioneering work we set out recently to shed light on the interaction of several POM (SiW12O404–, P2Mo18O626– and PW12O403–) with various metal ions like Ag+, Pd2+, Au3+, Cu2+, Ni2+ or Hg2+ in the presence of UV-near Vis light (λ > 320 nm). Our results on this topic suggest that, by and large, reduction of metal ions to their zero state form takes place in the presence of an organic substrate (S), i.e. alcohols or various organic pollutants, as electron donor and POM as photocatalyst in a net photocatalytic process, which is depicted in reaction
(2) |
We have shown that, depending on the experimental conditions, either precipitation–recovery of metals takes place, an issue of high environmental and economic benefits, or, low-ionic-strength solutions, synthesis of colloidal metal nanoparticles is achieved, a topic of great concern for materials science. We summarize herein the various parameters that influence this photocatalytic cycle.
2 Recovery of metals
Recovery of metals is a topic of great concern from environmental and economic aspect. Since many metals are either toxic or valuable, the development of methods that aim to recovery of metals could contribute to the solution of environmental problems and saving of finite resources as well.
It has recently been presented that photocatalysis with POM could contribute to recovery of metals. In that respect, illumination of an aqueous solution of metal ions in the presence of POM and organic substrate leads to the oxidative degradation of the organic and the simultaneous reduction–precipitation of metals in elemental state [12]. In particular, the mechanistic scenario comprises the photochemical oxidation of the organic by excited POM and the concomitant formation of reduced POM
(3) |
In turn, reduced POM are reoxidized by metal ions, without the need of light, via
(4) |
This process has been successful in the removal-recovery of a great variety of metal ions including either toxic metallic compounds (chromium and mercury [13]) or valuable metals such as copper [14], silver [15], gold, palladium [16] and platinum. It is effective even in traces of metal ions (ppm to ppb) that are completely removed from the treated solution (final concentration of ppb range), exhibiting quantum yield of ca. 5–10%. What is more, compared to the widely reported TiO2 photocatalyst, which is suspended in the aqueous solution, the homogeneous POM-based process exhibits some further advantages: (a) the final metal product is obtained in pure form and (b) no poisoning of the catalyst is marked, keeping the process catalytic throughout photolysis.
Fig. 3 illustrates the photo-degradation of 2,4-dichlorophenol (dcp) and the simultaneous quantitative recovery of silver (> 99.9%) in the presence of H3PW12O40 photocatalyst [12].
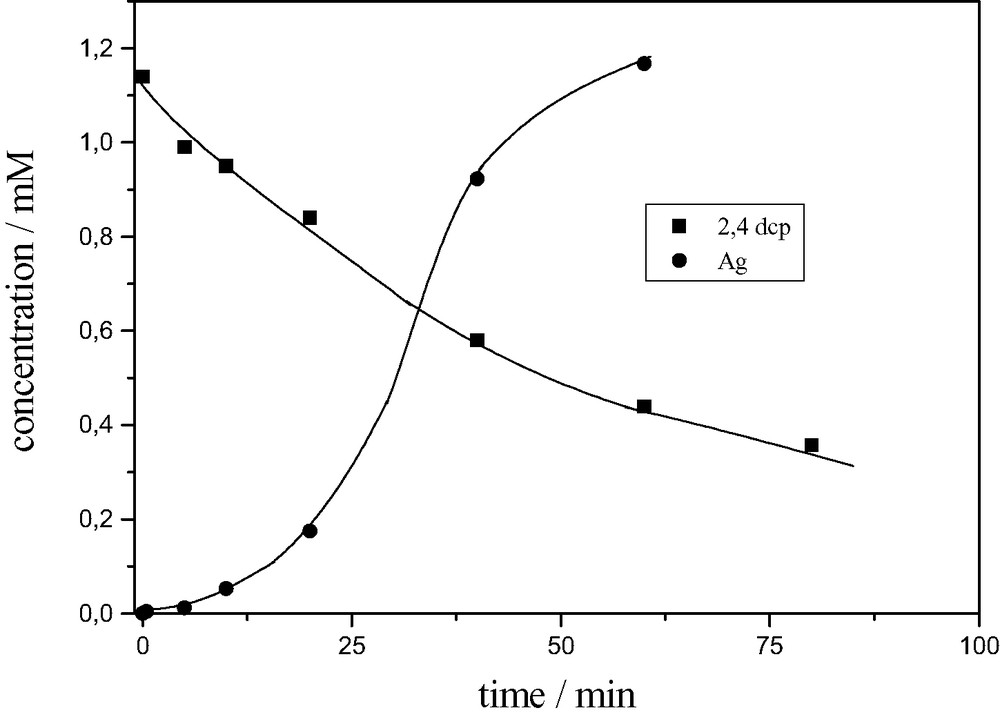
Degradation of 2,4-dcp and simultaneous recovery of silver upon photolysis of a deaerated solution 2,4 dcp (10–3 M), H3PW12O40 (7 × 10–4 M), Ag+ (1.2 × 10–3 M). pH 1, 4 ml, T = 20 °C, λ > 345 nm.
Many of the conventional methods implemented to recover metals are inhibited by the presence of organic compounds and a pretreatment step to remove or destroy organics is generally required. The POM-based method not only does not suffer from this problem, but also the presence of organic compounds promotes recovery of metals and vide infra. More specifically, an increase in the concentration of the organic accelerates the rate of metal reduction, since more reduced POM are produced via reaction (3), favoring reaction (4) [14,15,17]1. Moreover, using metal ions that are more efficient for reaction (4), according to the order Ag+ > Cu2+ > Ni2+ = no metal = 0, the degradation of organic is accelerated, since more amount of oxidized POM is available to participate in reaction (3) [12].
What is more, one does not have to search for a sacrificial electron donor, a rather expensive organic compound, but hazardous organic compounds, which have to be disposed of, can serve this function. In this way, purification of aquatic systems from both organic and inorganic pollutants can take place at ambient temperature in a simple one-pot process.
2.1 Redox control
2.1.1 Metal effect
As is the case in many reductive processes, the rate of metal ions reduction by POM follows thermodynamics, that is metals with more positive values of reduction potential are reduced and recovered in a higher rate. In the presence of a certain POM, i.e. the 1-equivalent reduced species SiW12O405– or PW12O404–, the reduction of easily reduced metals, such as Pd2+, Ag+, AuCl4–, Hg2+ or PtCl62– (Fig. 2) is favored. On the contrary Ni2+ ions, which are characterized by more negative reduction potential (Fig. 2), cannot abstract electrons from any of the studied reduced POM. A similar trend is noticed when using another POM, the two-equivalent reduced P2Mo18O628–. Its reoxidation rate by metal ions is higher in the case of Pd2+, which exhibits more positive redox potential, than Ag+.
In the case of Cu2+ ions, which exhibit intermediate values for reduction potentials [E0 (Cu2+/Cu0) = 0.337 V, E0 (Cu2+/Cu+) = 0.153 V, E0 (Cu+/Cu0) = 0.521 V vs. NHE], the extent of copper reduction depends on kinetic parameters, that is the competition of the further reduction of the obtained Cu+ to Cu0 with its reoxidation to Cu2+. Note that copper and mercury as well, do not precipitate immediately upon irradiation with UV-near Vis light of a (propan-2-ol/SiW12O404–/Mn+) solution. An induction period is observed in the start of metal deposition, attributed to the formation of the stable Cu+ or Hg+ intermediates. On the contrary, silver, which is directly reduced to Ag0, or palladium, which precipitates through the unstable Pd+ state, precipitate promptly to the elemental state.
Reduced POM are also named and serve as ‘soluble cathodes’. By careful choice of the redox potentials of POM (Fig. 2), selective reduction–precipitation of metal ions can be obtained. Fig. 4 depicts the selective precipitation of palladium from a mixture that also contains copper and nickel ions at 10-fold higher concentrations, using the POM SiW12O404– as photocatalyst.

Selective recovery of palladium from a mixture of Pd2+, Cu2+, Ni2+. Photolysis of a deaerated solution of SiW12O404– (7 × 10–4 M), propan-2-ol (10–3 M), Pd2+ (10−4 M), Cu2+ (10−3 M), Ni2+ (10–3 M). pH 3, NaClO4 0.1 M, λ > 320 nm, T 18 °C. (Reprinted from Ref. [16]).
2.1.2 POM effect
POM can serve as excellent probes for redox reactions as their reduction–reoxidation does not involve break or formation of chemical bonds and pure electron transfer takes place. Since the redox properties of POM can also be widely and precisely varied, depending on the nature of polyanions, manipulation of the efficiency of the process can be achieved by selecting POM of appropriate redox potentials.
POM catalysts that are more efficient in photo-oxidative degradation of organic compounds are also more effective in reductive recovery of palladium (Fig. 5). The rate of photo-reaction (3) follows the order PW12O403– > SiW12O404– > H2W12O406–, in consistence with the order of increasing value for the 1-electron reduction potential of POM. In this order, the reduced POM are produced in greater amounts and, thus are more efficient in reducing metal ions.

Photocatalytic reduction of palladium in the presence of various POM, upon photolysis (λ > 320 nm) of deaerated solutions containing propan-2-ol 1 mM, POM 0.7 mM, Pd2+ 0.1 mM, NaClO4 0.1 M (T 18.3 °C). (a) PW12O403– at pH 1, (b) SiW12O404– at pH 1, (c) H2W12O406– at pH 3 (Reprinted from Ref. [16]).
2.2 Operational parameters
2.2.1 Concentration
Increase of the concentration of either POM or organic or metal ions leads to an increase of the rate of metal recovery, however up to a saturation value, Fig. 6.
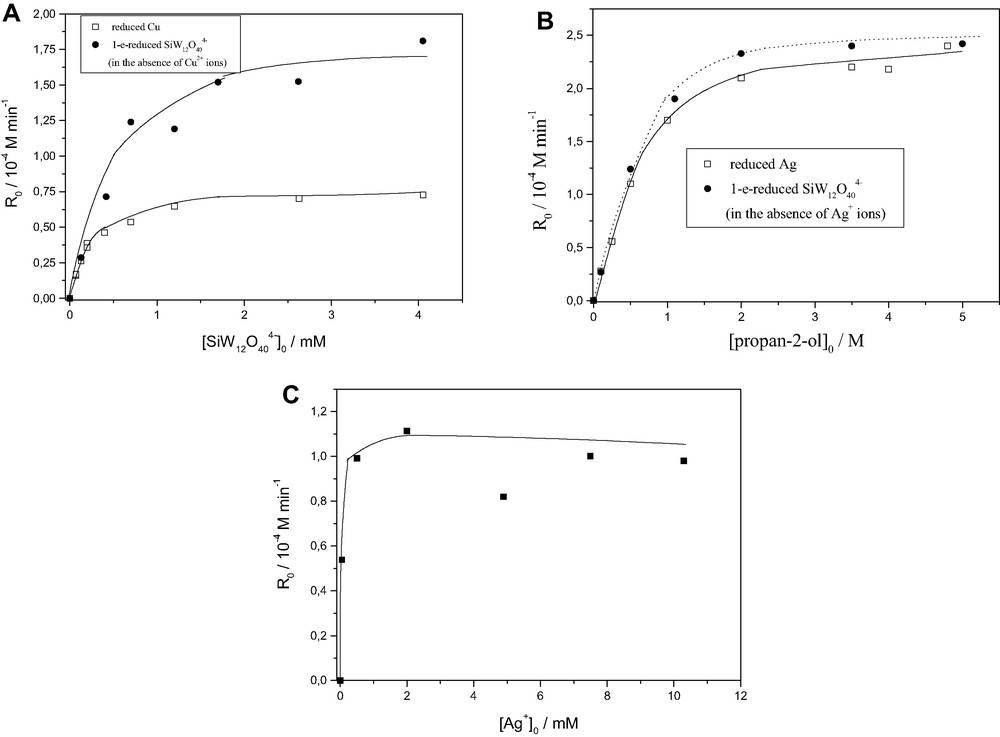
(A). Influence of SiW12O404– concentration on the initial rates, R0, of (□) copper recovery or (•) formation of one-equivalent-reduced tungstosilicate (SiW12O405–) in the absence of Cu2+. Deaerated solutions propan-2-ol 0.5 M, Cu2+ 2 mM.
(B). Influence of propan-2-ol concentration on the initial rate, R0, of (□) silver recovery or (•) formation of one-equivalent reduced tungstosilicate (SiW12O405–) in the absence of Ag+. Deaerated solutions of K4SiW12O40 0.7 mM, AgNO3 2 mM.
(C). Effect of silver ions concentration on the initial rate of silver recovery. K4SiW12O40 0.7 mM, propan-2-ol 0.5 M.
(In all cases NaClO4 0.1 M, pH 5, λ > 320 nm, T 18 °C).
(Reprinted from Refs. [14,15]).
Note that the quantum efficiency of metal recovery reflects the quantum efficiency of formation of the reduced POM in the absence of metal ions, following the corresponding stoichiometry. For instance, when 1-e–-donor photoreduced POM reacts with a 2-e–-acceptor Cu2+, the stoichiometry observed is 2:1 (Fig. 6A), while in the case of Ag+, a 1-e–-acceptor, the corresponding stoichiometry is 1:1(Fig. 6B).
Moreover, as can be seen in Fig. 6C the process is effective in a wide range of metals concentration, varying from ca. 3 to 1300 ppm. The wide range of metal concentration at which the POM method is applied as well the low final concentration of metal ions left in the solution, can account for the environmental assessment of the process.
2.2.2 Oxygen
The presence of oxygen in the illuminated solutions retards the precipitation of metal ions. However, the process remains quite efficient. Dioxygen is an efficient electron acceptor from POM (e–) [18], but not as effective as metal ions, i.e. Ag+, Cu2+ or Hg2+, under these certain conditions. Therefore the slight detrimental role of dioxygen in the recovery of metals is not attributed to antagonism for reaction (4), but rather to reoxidizing some of the intermediates obtained upon the reduction of metal ions, for instance Cu+, Agcolloidal or Hgcolloidal.
2.2.3 Influence of anions and ionic strength
Anions that complex strongly metal ions may alter drastically their reduction potential and affect to a great extent the efficiency of the process. For instance, the presence of S2O32– in concentration 1 M in a (Ag+/POM/propan-2-ol) solution is enough to halt the otherwise prompt reduction of silver, through the formation of stable Ag(S2O32–)23– complexes [15]. Similarly, the presence of 0.01 M Cl– in a Cu2+/POM/propan-2-ol solution can retard the precipitation of red copper particles, through stabilization of the intermediate cuprous ion as CuCl [14]. On the contrary, the presence of NO3– or ClO4– that do not complex the metal ions leaves the process unaffected.
What is more important, the ionic strength affects not only the rate but also the nature of the metal product obtained. At high ionic strength values (> 3 × 10−2 M) elemental metals precipitate. However, at low values (< 10−2 M) a slower reduction of metal ions takes place, resulting in the formation of colloidal metal particles [19].
From the aspect of metals recovery, an increased ionic strength solution is desirable not only because the process is speeded up but also because, in this way (i) the recovery of metals is quantitative and (ii) the obtained metal product is more stable against corrosion, since the co-formation of the oxidizable metal nanoparticles, which could act as corrosion centers, is avoided.
3 Synthesis of metal nanoparticles
We have recently shown that by keeping the ionic strength low, illumination of a (Substrate/POM/Mn+) solution leads to formation of metal nanoparticles, through a process in which POM serve both as photocatalysts-reducing reagents and stabilizers [19]. According to Eq. (5)
(5) |
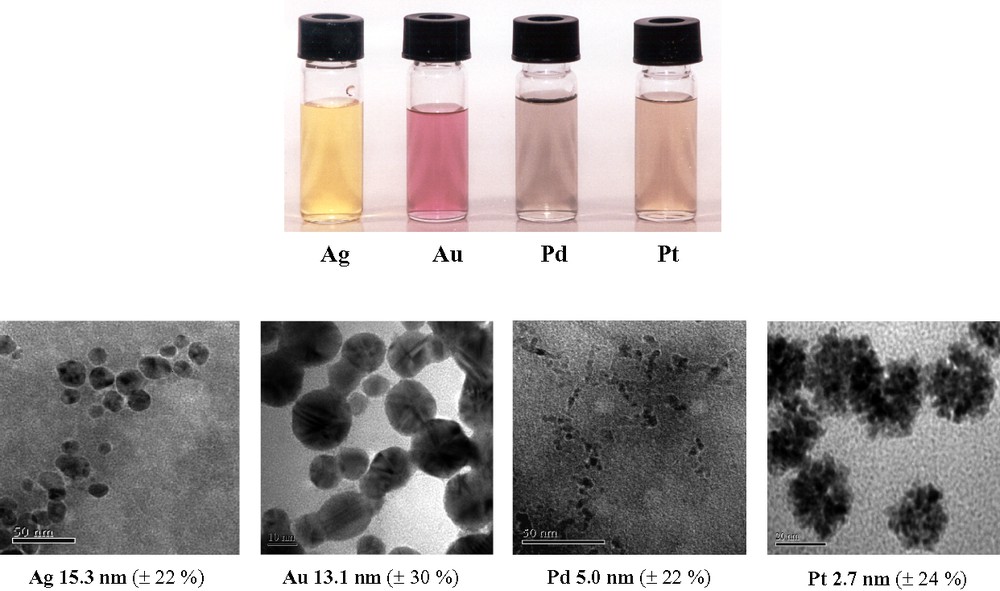
Color variation (upper part) and TEM graphs of Ag, Au, Pd and Pt solutions (lower part), obtained upon mixing of photochemically produced, deaerated blue solution of SiW12O405– (3.5 × 10–4 M) with solutions of the corresponding metal ions, AgNO3, PdCl2, H2PtCl6 (final concentration 10–4 M) or HAuCl4 (final concentration 10–3 M), pH 5, T 20 °C (Reprinted from Ref. [20]).
The process exhibits both practical and academic interest. From the practical point of view, it involves an environmental friendly and simple way to synthesize metal nanoparticles since: (a) It takes place at room temperature, rapidly, within a few seconds, utilizing a mild reductant, i.e. SiW12O405– (0.057 V vs. NHE) in catalytic amounts. On the other hand, other reducing methods that proceed at room temperature need extremely strong reductants such as BH4–, hydrogen atoms, solvated electrons or organic radicals that are formed upon radiolysis with γ-rays or sonolysis of aqueous solutions, while other methods that utilize mild reductants request high-stoichiometric amounts and heat in order to complete even after hours or days. (b) The synthesis of metal nanoparticles is achieved in water, a non-toxic solvent. (c) POM, besides reductant, serves also as stabilizer, avoiding the addition of a second, sometimes toxic, reagent that would be needed to arrest the metal particles in nano-dimensions.
This method has paved the way for the synthesis of more complicated nanostructures using POM as photocatalysts. In particular, Sastry in India has used the photocatalytic POM process in order to prepare
- • bimetallic core-shell nanoparticles by synthesizing POM-stabilized gold nanoparticles and then photoreducing the POM stabilizers on the metal surface. This restricts the reduction of the added metal ion (silver) on the surface of the preformed nanometal and core-shell gold-silver particles are obtained [20];
- • silver nanorings, by forming a silver layer on a POM-Ag+ nanostructured salt and then dissolving with base the POM in the interior, leaving the outer ring intact [21];
- • gold nanosheets are formed when photo-reduced POM, immobilized on anthracene that are bound on a liquid-liquid interface, transfer their electrons on gold ions [22].
4 Conclusion
POM act as photocatalysts for the reduction of metal ions by organic electron donors, foreseeing to either i) the removal-recovery of metal precipitates and the concomitant degradation of organic pollutants or ii) the synthesis of metal nanoparticles. They exhibit interesting activity (efficient even for traces of metal ions) and selectivity as well, adjusted by the selection of POM with suitable redox potentials, while operational parameters such as the concentration of POM, organic substrate, metal ions and oxygen affect the photocatalytic cycle.
1 In this concept, one parameter that has to be taken into account when such systems for efficient recovery of metals are established is, beyond the electron donation ability of the organic, the solubility limitations. A representative example is this of atrazine, which has a low solubility in water (30 ppm). No precipitation of copper ions is obtained when atrazine is the sole organic substrate in aqueous solutions, since it produces insufficient concentration of reduced POM to cause precipitation.