1 Introduction
Rhenium octahedral cluster chemistry is based on the well-established Re6L14 unit, built from a rhenium octahedron, characterized by twelve metal–metal bonds [1,2]. This metal cluster core is enclosed in a cube of ‘inner’ ligands Li capping each triangular face of the Re6 octahedron, and six ‘apical’ ligands located on terminal positions through the apices of the metal octahedron. The resulting and well-defined Re6L14 units are similar to the molybdenum and tungsten ones. In all these compounds, the transition element is in the d4 state, meaning that it is in the oxidation number + II for Mo and W and + III for Re. It follows that these chemistries are parallel, but that the rhenium compounds are, as a general rule, obtained with more charged anionic ligands: as an illustration, no pure halide is known for rhenium, in contrast to molybdenum and tungsten, while a number of rhenium octahedral clusters have been reported in chalcogenide and chalcohalide chemistries.
The synthesis of the octahedral clusters clearly belongs to the field of solid state chemistry as the synthesis of such compounds requires high temperatures in reducing conditions. In contrast, when the cluster compounds are formed, due to the solubility in polar solvents of many of these very stable entities, they can be used as precursors for the synthesis via solution chemistry route and for structural characterization of new classes of molecular compounds.
First, it is necessary to determine in detail the pertinent phase diagrams. As an illustration, the Cs–Re–S–Br system has been selected, on the basis of the numerous phases potentially present and its specific suitability for accurate structural determinations.
In a second step, the cluster-based anionic units, which behave like building-blocks, can be functionalized, via a solution chemistry route, by organic ligands or/and assembled with a variety of inorganic, organic or metal–organic counter-cations, exhibiting different geometries. A wide variety of original phases have then been obtained and structurally characterized. In many examples they obey to stacking rules, which correspond to homothetic expansion of standard structure-types.
An early survey of rhenium clusters with various nuclearities can be found in Ref. [1], and a very exhaustive and comprehensive review on the chemistry of hexanuclear rhenium chalcohalide clusters has been published recently by P. Batail et al. [2]. In the present account, we will remind the rules governing the formation via solid state reactions of various cluster-units and their interactions, highlighting the most recent results, and then we will give some illustrations of their use as building blocks for coordination chemistry.
2 Solid-state chemistry
2.1 General synthesis
High-temperature syntheses are usually carried out in sealed silica tubes, from powdered elements (Re, S, Se) and sometimes, metal halides. Halogen is also introduced for instance as ReCl5 or liquid bromine, the latter being introduced under argon and frozen before sealing the mixture in a silica tube. Stoichiometric amounts are used, with the exception of bromine, for which an excess of 10–20% is generally used. Reaction temperatures are typically in the 700–950 °C range for a reaction time of 24–48 h; very long times (up to 10–15 days) are sometime used in order to increase the size of crystals.
Under these conditions, numerous original compounds have been reported. As an example, a quaternary diagram, relative to the Cs–Re–S–Br system, is displayed on Fig. 1, using a pseudo-ternary representation, where ternary compounds lie onto the axes, and quaternary ones inside the triangle.
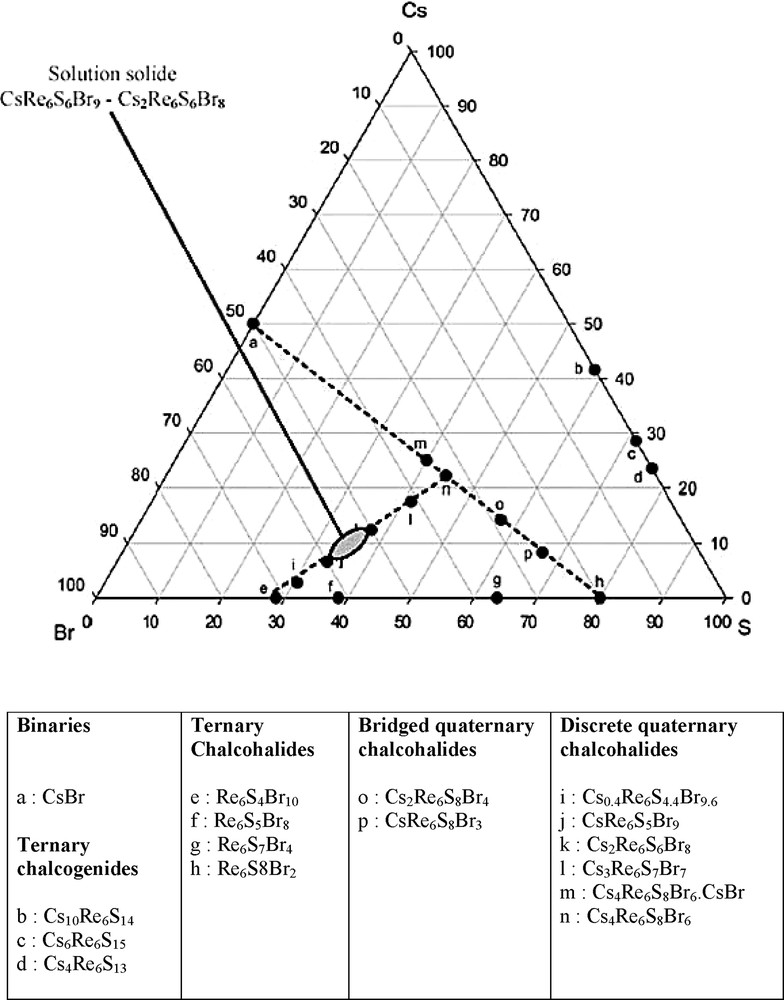
Representation of the quaternary diagram relative to the Cs–Re–S–Br system. Here is used a pseudo-ternary representation, where ternary compounds lie onto the axes, and quaternary ones inside the triangle.
2.2 Predictable approach of expected phases
Expected phases can be predicted using together the following rules:
- • rhenium is in the oxidation state + III;
- • the elementary building block is the unit Re6L14 or more precisely Re6Li8La6, where Li can be a mixture of halogen and chalcogen, very often with a statistical disorder, but with an overall composition such as (XxY1–x)i8 where x is an integer, while La is preferentially a halogen ligand. It is to be noted that, due to the often-encountered statistical distribution on inner ligands, it is advisable to choose systems where halogen and chalcogen ligands have very different diffusion factors in order to be able to accurately derive the stoichiometry from structural determination;
- • the cluster units can be either isolated or interconnected via two types of bridges, following a nomenclature early established by H. Schäfer [3]: i) simple inter-cluster bridges La–a where the bridging atom is in apical position for the two adjacent clusters (Fig. 2a) and is systematically a halogen (with the unique exception of the ternary chalcogenides described below, where there is obviously no choice) ; ii) double inter-cluster bridges La–i, meaning that the bridging atom is in apical position for a cluster and in inner position for the adjacent one (Fig. 2b) ; indeed, each bridging atom will be counted for one in the environment of the cluster (in order to maintain the Re6Li8La6 unit formula), and counted for 1/2 for establishing the chemical formula of the compound; a third bridging type, Li–i, has been reported in the crystallochemistry of molybdenum clusters, but do not seems to form in the case of rhenium;
- • the VEC (Valence Electron Count), defined as the number of electrons available to form metal–metal bonds, is fixed to 24, implying twelve order-one bonds; the VEC is easily calculated, taking into account seven valence electrons for each rhenium atom in the d4 state, then deducing the number of electrons transferred to the ligands, in an ionic model, and finally adding the electrons transferred from the eventual additional element to get a counter-cation; this ‘magic number’, corresponding to fulfilling the metal–metal bonding states of the Re6L14 molecular orbital diagram, is respected in most cases, although a VEC of 23 has been reported in limited examples, in which the cluster has been oxidized [2,4];
- • if an anionic charge is carried by the unit, it has then of course to be balanced by counter-cations.
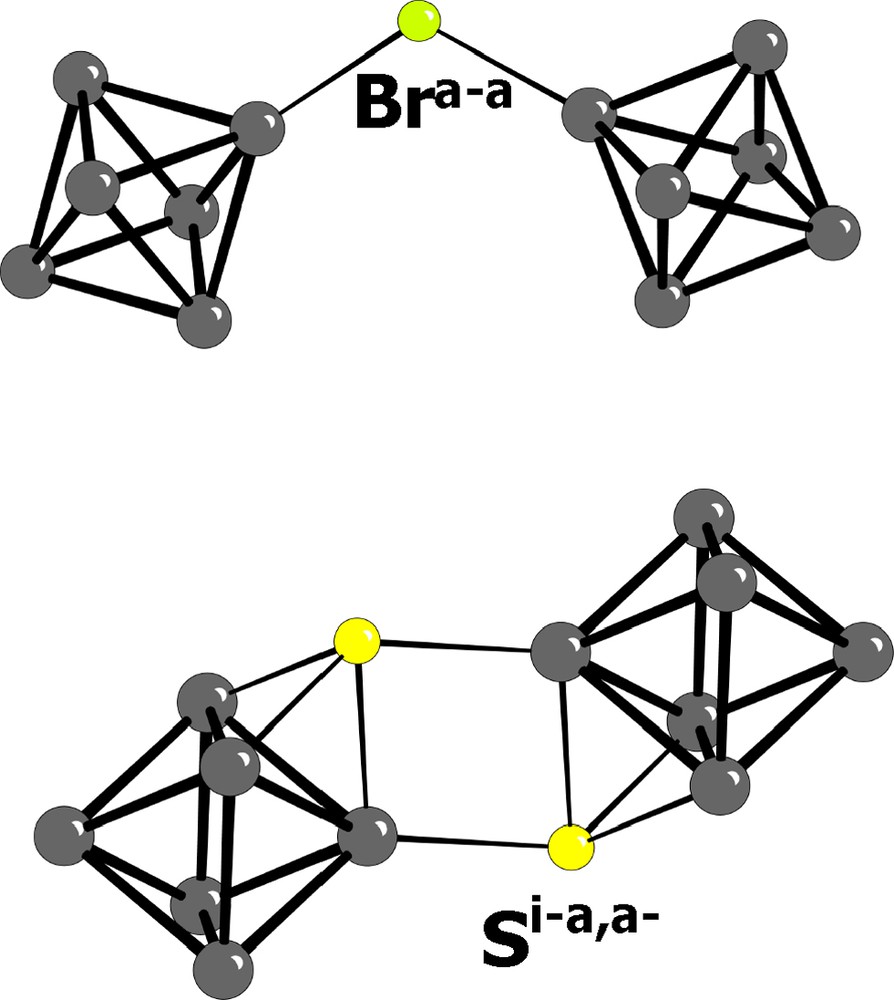
Top: simple inter-clusters bridge La–a. The bridging atom is in apical position for each of the two adjacent clusters. Bottom: double bridges La–i. The bridging atom is in apical position for one cluster and in inner position for the adjacent one.
The results are illustrated in Table 1, in the example of rhenium thio-bromides. Going from the left to the right along a row corresponds to replacing two bromines by one sulfur ligand, without charge change, but implies a decrease by unity of the total number of available ligands, and then the formation of an inter-clusters bridge. In contrast, from the top to bottom is the successive addition of one cation (univalent in this example), and then of one bromine, implying the breakage of an inter-cluster bridge.
Formulation of the Re6 cluster-based predictable phases in the M–Re–S–Br systems (chemical formula and structural formula(e), just below)
Re6S4Br10 Re6[S4Br4]Br6 | Re6S5Br8 Re6[S5Br3]Br4Bra–a2/2 | Re6S6Br6 Re6[S6Br2]Br2Bra–a4/2 Re6[S4Si–a2/2Br2]Sa–i2/2Br4 | Re6S7Br4 Re6[S7Br]Bra–a6/2 | Re6S8Br2 Re6[S6Si–a2/2]Bra–a4/2Sa–i2/2 |
MRe6S5Br9 {Re6[S5Br3]Br6}– | MRe6S6Br7 {Re6[S6Br2]Br4Bra–a2/2}– | MRe6S7Br5 {Re6[S7Br]Br2Bra–a4/2}– {Re6[S5Si–a2/2Br]Sa–i2/2Br4}– | MRe6S8Br3 {Re6[S8]Bra–a6/2}– | |
M2Re6S6Br8 {Re6[S6Br2]Br6}2– | M2Re6S7Br6 {Re6[S7Br]Br4Bra–a2/2}2– | M2Re6S8Br4 {Re6[S8]Br2Bra–a4/2}2– {Re6[S6Si–a2/2]Br4Sa–i2/2}2– | ||
M3Re6S7Br7 {Re6[S7Br]Br6}3– | M3Re6S8Br5 {Re6[S8]Br4Bra–a2/2}3– | |||
M4Re6S8Br6 {Re6[S8]Br6}4– |
Note that, for a given stoichiometry, it is possible within the above assumptions, to derive several developed formulas: as it can be seen in Table 1, it depends on the distribution of the ligands (inner, apical and a–a or i–a bridging). Some factors such as steric hindrance or preferential inner or apical site occupancy could favor a given architecture.
Finally, the bottom-left part of the Table is empty because it would correspond to cationic units. A few examples of the latter have been reported in the case of Nb6 and Ta6 clusters [5] but are, up to now, unknown in the case of rhenium, with simple ligands: only the dications [Re6Te8(TeBr2)6]Br2 [6] and its iodine homologue [7] have been reported; they are obtained under medium temperature conditions (400–450 °C), starting from a precursor containing already the trinuclear or hexanuclear rhenium cluster (Re3Br9 and Re6Te15, respectively).
2.3 Ternary compounds
A number of alkaline or alkaline-earth sulfides or selenides of rhenium, based on the Re6Y14 unit have been obtained in the W. Bronger group. Depending on the stoichiometry and synthesis conditions, most of the reported compounds present apical–apical bridges of sulfide Sa–a or disulfide (S–S)a–a type extending along one to three directions [1,8,9]. An exception is the Cs10Re6S14 compound, based on isolated cluster anion, with a very important negative charge [10].
Ternary rhenium chalcohalides based on octahedral Re6 clusters have been reported and are described in detail elsewhere [1]. They correspond to the general formula Re6X4+xY10–2x (integer 0 ≤ x ≤ 4). Then the first term, for instance Re6S4Br10 corresponds to isolated units, while the three following ones illustrate a progressive unit condensation along one, two and three directions of the space. The final step is illustrated for instance by Re6S8Br2, that is built from one pair of sulfide inner-apical bridges and two apical bromine bridges leading to the 3-D Re6[Si6 Si–a2/2] Sa–i2/2Bra–a4/2, while the chloro-seleno homologue Re6Se8Cl2 exhibits a strongly 2-D structure with two selenide inner-apical double bridges and terminal apical chlorines, and is written as Re6[Sei4 Sei–a4/2] Sea–i4/2Cla2.
2.4 Quaternary compounds
They obey of course to the same rules as the ternary chalcohalides, but, due to the charges equilibrium, the number of combinations is even larger, leading to a very rich crystallochemistry.
2.4.1 Discrete units
They lie on the diagonal of Table 1, which corresponds to the richest possible alkaline formulations. Of course, all of them exhibit six apical bromine ligands.
All relevant examples of compounds are known, although there are some unexpected results:
- • a non integer compound, Cs0.4Re6S4.4Br9.6, is isostructural [11] with the monoclinic potassium thiochloride KRe6S5Cl9, while CsRe6S5Br9 presents, in contrast, a trigonal structure extending up to Cs2Re6S6Br8 along a solid solution;
- • a second non-integer phase, Cs2.5Re6S6.5Br7.5, has been detected [11] but not fully characterized;
- • the compound at the right bottom end of the Table, corresponding to the highest anionic charge, Cs4Re6S8Br6, was unknown up to very recently. In contrast, Cs5Re6S8Br7 is easily synthesized and could be written as a double salt Cs4Re6S8Br6, CsBr in accordance with the above nomenclature. However, from the structural determination [12] the latter is better described as a salt of a tetravalent complex cation (Cs5Br)4+(Re6S8Br6)4– (see Fig. 3a). The ‘true’ tetra-anionic unit based Cs4Re6S8Br6 has been recently obtained as a secondary phase in the preparation of Cs5Re6S8Br7 and structurally characterized [13]; the arrangement of cesium cations is very different, as they form a very regular cuboctahedron (Fig. 3b) surrounding the tetra-anionic unit.

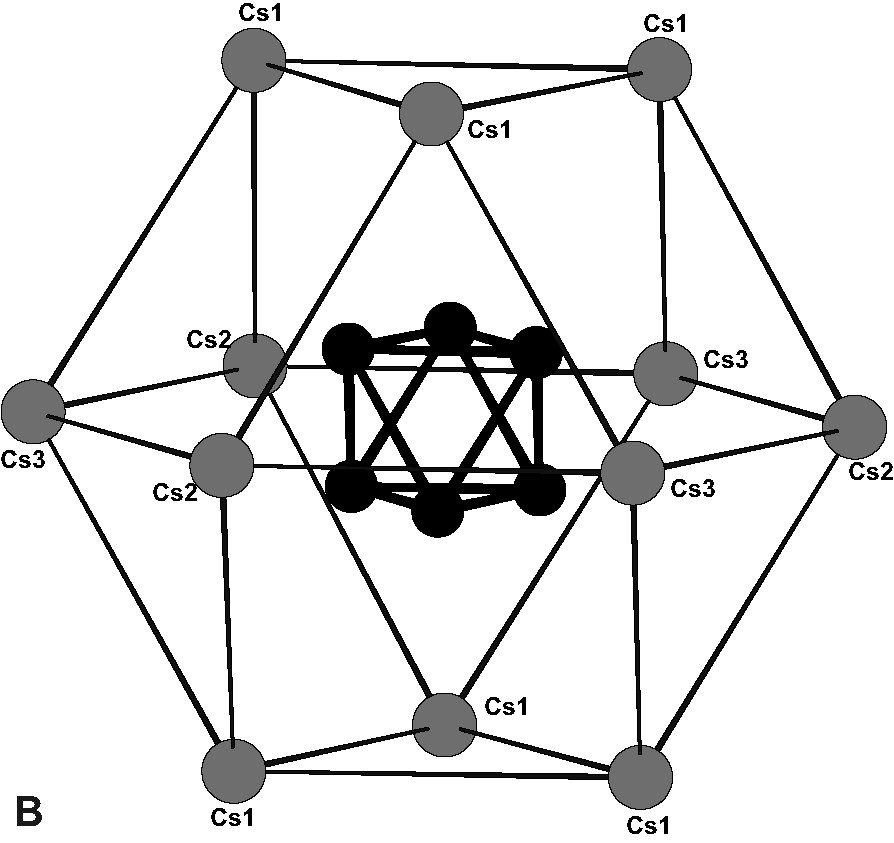
(A) Description of the (Cs5Br)4+ tetravalent complex cation in the (Cs5Br)4+(Re6S8Br6)4– salt (bromine positions half occupied). (B) Arrangement of caesium cations in the Cs4Re6S8Br6 structure as a very regular cuboctahedron surrounding the tetra-anionic unit.
2.4.2 Bridged units
The number and arrangement of ligands shared by adjacent units strongly influences the dimensionality of the structure of the compounds. Indeed, two new low dimensional phases have been recently synthesized and structurally characterized, completing the data of Table 1:
- • CsRe6S8Br3 [14] has for developed formula Cs{[Re6Si6 Si–a2/2]Sa–i2/2Bra–a2/2Bra2}, meaning a polymeric structure where the clusters are linked by both a pair of double sulfur bridges in one direction and a pair of apical bromine bridges in a second one (Fig. 4). This gives a strong 2-D character to this structure, isostructural with the previously reported seleno-iodide CsRe6Se8I3 [15];
- • Cs2Re6S8Br4 [16], with the structural formula Cs2{[Re6Si6 Si–a2/2]Sa–i2/2Bra4}, forms infinite chains where the clusters are linked by a pair of double sulfur bridges leading to a 1-D framework (Fig. 5). It turns to be isostructural with Cs2Re6Se8Br4 [15] and Rb2Re6S8Br4 [11];
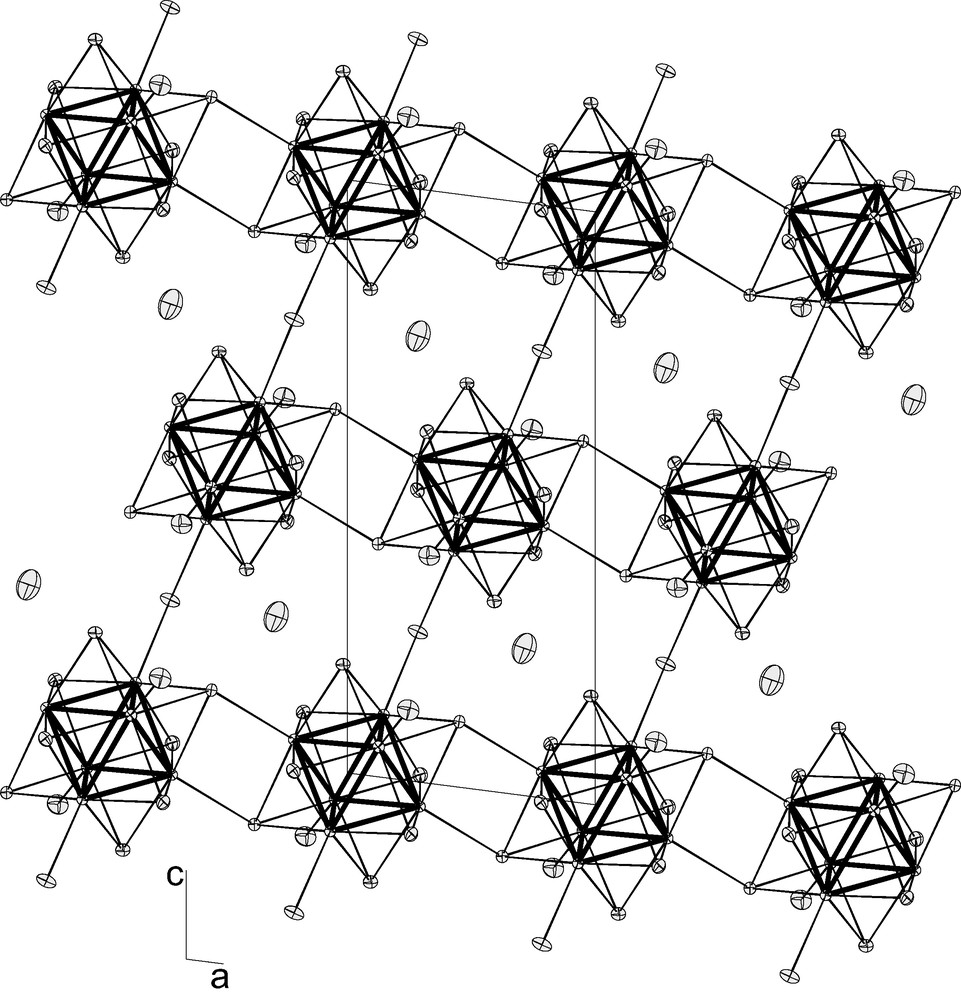
Projection of the structure of CsRe6S8Br3 in the (a,c) plane, illustrating the presence of the {[Re6Si6 Si–a2/2]Sa–i2/2Bra2}– linear chains linked in the second direction by Bra–a bridges.
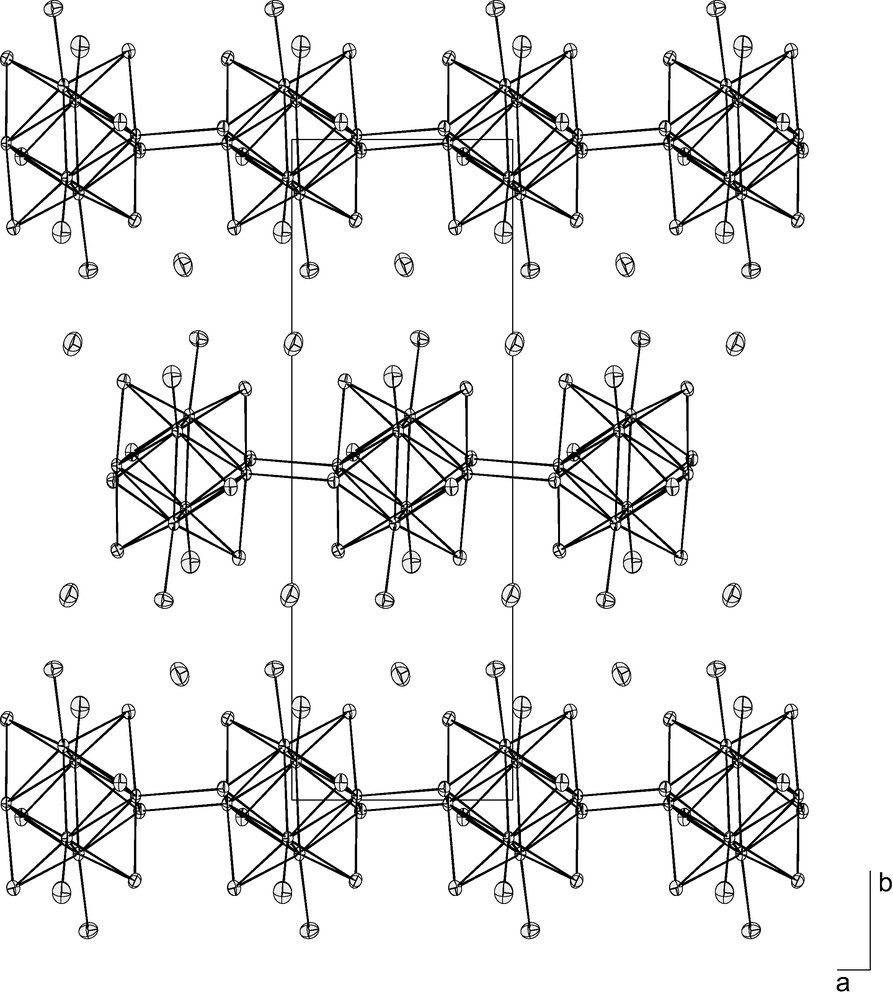
Projection of the structure of Cs2Re6S8Br4 in the (a,b) plane, illustrating the presence of the {[Re6Si6 Si–a2/2]Sa–i2/2Bra4}2– linear chains alternated with Cs+ counter cations.
3 Solution chemistry
The solubility of some of the above cluster-based compounds (discrete anionic units) in simple polar solvents [17,18] has opened the way to a new approach, of the ‘chimie douce’ type, for rhenium octahedral clusters. Relevant objectives are for instance to access to new materials unattainable by direct synthesis due to the thermal fragility of one partner, to control the self-organization of clusters using the partners as spacers (or vice-versa) and to open the route to new inorganic–organic interface chemistry in order to build functionalized cluster materials. The strategies are to exchange the cationic counterparts, to exchange the ligands of the cluster unit, or both of them simultaneously. Such reactions can be carried out in solution, taking advantage of the solubility of some cluster-unit based compounds.
3.1 Solubility of rhenium cluster compounds
The compounds obtained by solid state chemistry are expected to be usable as precursors for a chemistry carried out in solution. Of course, compounds exhibiting a structure where the units are connected by bridges are not soluble, due to the presence of a rigid framework unless they are submitted to so-called [15,19] ‘excision’ reactions: the latter expression means that inter-cluster bridges are broken by a chemical reaction, for instance with alkaline halide, and then the pending ligand sites are filled by the imported halogen ion. In fact, it is usually more efficient to synthesize directly the resulting compound by solid state reaction.
In contrast, ionic compounds based on isolated units present in most cases significant solubility in common polar solvents. Examples are given in Table 2, which shows the lack, up to now, of any systematic. A significant example is illustrated by the comparison of the behavior of Cs5Re6S8Br7 (Cs4Re6S8Br6, CsBr or more likely (Cs5Br)4+(Re6S8Br6)4–) and Cs4Re6S8Br6: in fact (Cs5Br)4+(Re6S8Br6)4– is readily soluble in water, while the latter is not (this difference allowed to isolate it from the reaction mixture), although both compounds are based on the same tetravalent anionic unit (Re6S8Br6)4–. Even more unexpected is the result of the recrystallization of water solutions of Cs5Re6S8Br7, that gives again the starting compound. In that case, it could have been expected that the complex cation (Cs5Br)4+ would have been broken in solution, and then that Cs4Re6S8Br6 would have precipitated, the additional CsBr remaining in the solution.
Solubility of some Re6 cluster anionic units
Compound | H2O | CH3CN | EtOH | CH2Cl2 |
Cs4Re6S8Br6.CsBr | Yes | No | Low | No |
Cs4Re6S8Br6 | No | ? | ? | ? |
Cs3Re6S7Br7 | Yes | Low | Yes | No |
Cs2Re6S6Br8 | No | Yes | No | Yes |
CsRe6S5Br | Low | No | Yes | No |
Early experience has shown that substituting the inorganic cation by a tetra-alkylammonium salt, using a simple cation metathesis reaction, increases to a large extent the solubility of the cluster materials in common organic solvents [18].
3.2 Exchange of cationic partner
This route is illustrated by many examples, involving either solvated or complexed inorganic cations, or the use of organic or organometallic partners. Due to the large size of the latter in comparison to the starting inorganic cation, very different stackings are evidenced for the final hybrid compounds.
3.2.1 Solvated counter-cation
An example is the structure of Ca(DMSO)6Re6S6Br8 [20] obtained in MeCN from the cesium or, more efficiently, the tetrabutylammonium salt of the dianion [Re6S6Br8]2–, in the presence of CaBr2 which was solubilized by the addition of DMSO complexant. The structure is built from rows of [Re6S6Br8]2– units and [Ca(DMSO)6]2+ cations where Ca is octahedrally coordinated by the oxygen atoms of the solvate (Fig. 6). Due to the 1:1 stoichiometry and the analogous overall size of the two partners, the stacking is a giant homothetic analogue of the CsCl type-structure.
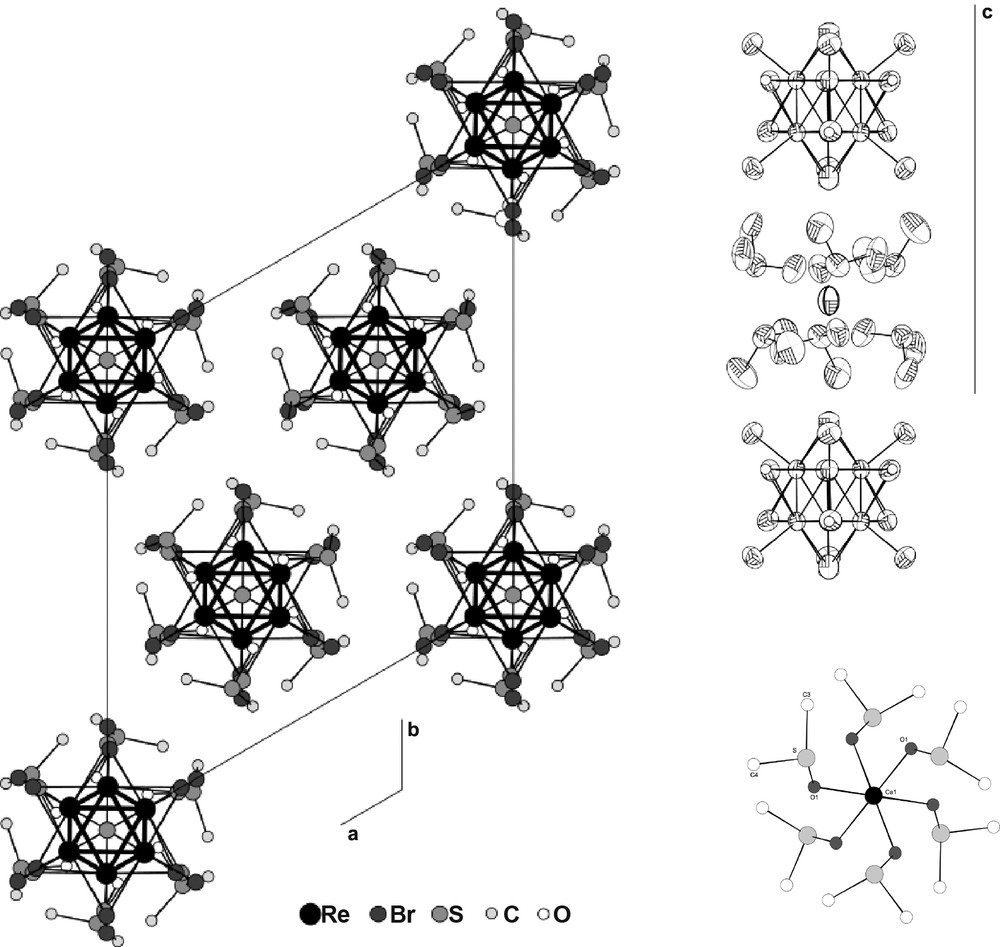
Structure of Ca(DMSO)6[Re6S6Br8] which is built from files of [Re6S6Br8]2– units and [Ca(DMSO)6]2+ cations where Ca is octahedrally coordinated by the oxygen atoms of the solvent. Left: projection in the (a,b) plane; top right: view of one column; bottom right: the Ca environment.
3.2.2 Complex counter cation
An illustration is given by the synthesis of the crown-ether derivative Cs2(18-crown-6)3 Re6S6Br8 obtained in MeCN by direct complexation (Fig. 7) [20]. Because Cs is too large to be accommodated in the cavity of the crown-ether, a 2:3 ‘club-sandwich’ is formed, that presents a rare example of non-rotational disordered crown-ether. Due to the local environment, the central crown-ether molecule has the usual symmetry, while the outer ones, standing in a strongly asymmetric environment, just between the cluster anion and the Cs cation, exhibit a strongly distorted conformation (all the oxygen atoms being pushed on the same side of the mean plane of the molecule), while all the distances and angles maintain their usual values. Again, the similar size of the two partners leads to a homothetic CsCl stacking, in which the inter-cluster distance (center to center) reaches 12 Å, to be compared to the 9.32 Å separation in the Cs2Re6S6Br8 precursor.

Structure of Cs2(18-crown-6)3Re6S6Br8 built from rows of [Re6S6Br8]2– anions and a so-called ‘2:3 club-sandwich’ complex cation based on two Cs cations inserted between three crown-ether (right).
3.2.3 Organic cations
Examples of cluster anions assembled with tetra-alkyl ammonium cations are known for long time [18]. A further illustration is the recently obtained structure [20] of (n-Bu4N)2[Re6S6Br8], displayed in Fig. 8. As stated above the interest in these hybrids is an increased solubility in organic solvents, opening the way to specific inorganic–organic assemblies. Relevant examples are the hybrids early synthesized with analogues of tetrathiafulvalene by an electro-crystallization route [18].
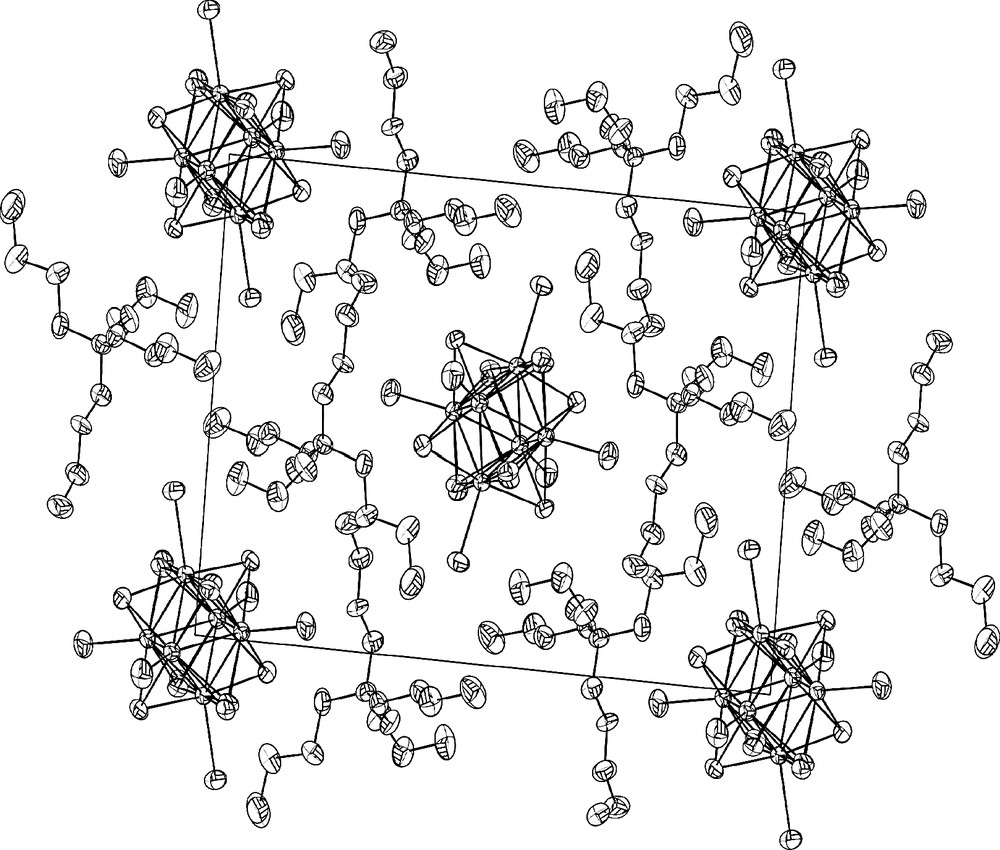
Projection of the structure of (n-Bu4N)2[Re6S6Br8] (inner ligands omitted for clarity).
3.2.4 Organometallic compounds
The chemistry of transition metal organometallic complexes, dominated by the classical 18-electron rule, is interesting to study with complexes with 17 electrons, possibly leading to the discovery of new electron transfer reactions and the access to new physical properties. However, experience shows that small anions, like PF6–, BF4– or even BPh4– commonly used in organometallic chemistry often lead to crystals of quite poor quality. For this reason, we have explored the association of organometallic cations and large-sized anionic metal clusters. In this type of synthesis, the metallic cluster plays the role of a ‘crystallization improver’ and helps for the structural determination by X-ray diffraction due to well organized heavy atoms. Very recent illustrations of this strategy are the synthesis and the structure determination of two organometallic–inorganic hybrids:
- – (i) [Cp*(dppe)Fe–NCCH3]2[Re6S6Br8] has been prepared by simple ionic metathesis from Cs2 Re6S6Br8 and [Cp*(dppe)Fe–NCCH3]Cl in MeCN and elimination of the formed CsCl precipitate [21]. The structure is shown in Fig. 9. The two entities keep their own individuality and interact by coulombic forces.
- – (ii) The [FeCp2]2[Re6S6Br8]2(OC(CH3)2) ferricinium salt has been prepared in order to replace, for the same reasons as above, the standard [FeCp2][PF6] for the oxidation of organometallic complexes. It is again obtained by a simple metathesis in MeCN followed by a recrystallization from a pentane–acetone mixture [22]. This reaction was possible because the redox potentials of the two entities are such that no electrochemical reaction can occur between them. The structure (Fig. 10) can be described as a succession of planes occupied by the anions and strongly corrugated ladders built from the ferricinium ions.
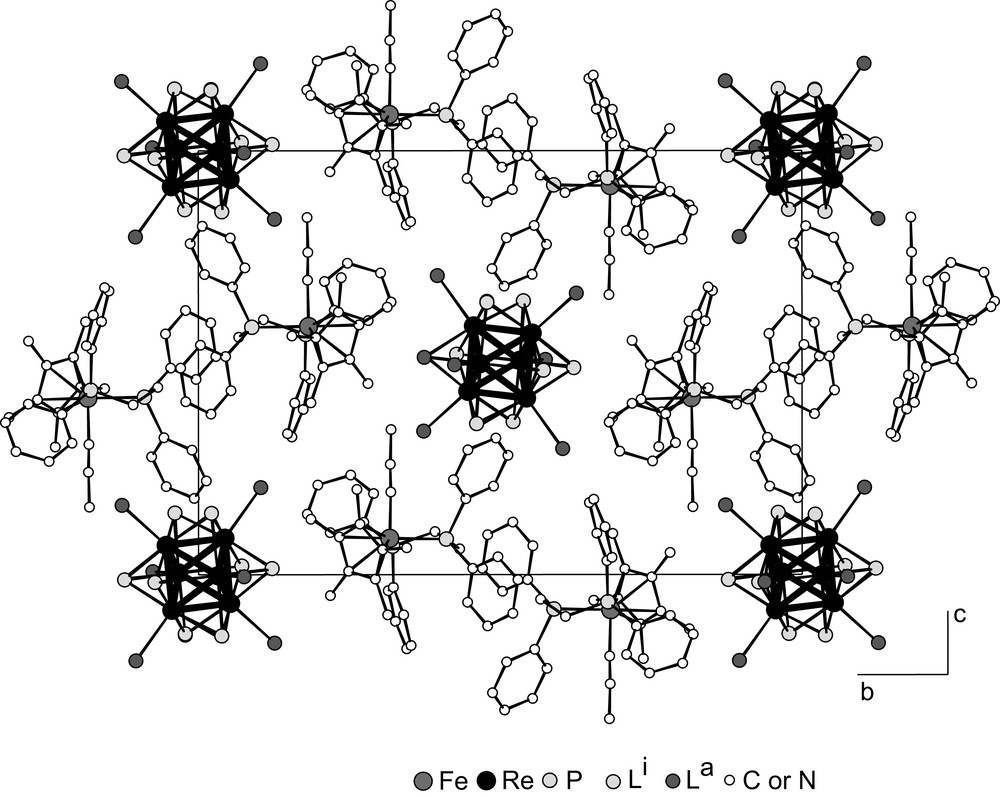
[Cp*(dppe)Fe–NCCH3]2[Re6S6Br8] structure projected in the (b,c) plane of the unit-cell (inner ligands omitted for clarity).

Description of the [FeCp2]2[Re6S6Br8]2·(OC(CH3)2) ferricinium salt structure (inner ligands omitted for clarity).
3.3 Exchange of apical ligands
Another important field of research is the use of the above solid state compounds as cluster precursors in order to prepare Re6Li8(CN)a6 units in which the apical ligands are replaced by ambidentate cyanide groups ([23] and references therein).The first example of a rhenium cluster with apical CN ligands was KCs3Re6S8(CN)6 obtained by reaction at medium temperatures (500 °C) of the cluster-based Re6Te15 with KSCN [24]. Subsequently an extensive study of the cyano compounds, including especially octahedral rhenium clusters has been carried out in the group of V.E. Fedorov, as reviewed in [23].
The use of the longer and symmetric N3 chain instead of the asymmetric CN group should allow access to relevant M6Li8(N3)a6 building blocks that should greatly influence the structure and the properties of the resulting compounds. The interaction of the above, water soluble, Cs4Re6S8Br6,CsBr solid state compound with aqueous solutions of sodium azide has been investigated, giving access to the new cluster compound Cs4Re6S8(N3)6·H2O. The latter constitutes the first example of a Re6 compound containing N3 apical ligands [25]. Its structure consists in three crystallographic independent azide (N3)– anions that are linked to the cluster in such a way that the value of Re–N–N angle is close to 120°. This value is obviously related to the sp2 hybridization of the nitrogen atoms of the azide chain. In the resulting Re6Si8(N3)a6 unit, four of these ligands are ordered, while the two other ones are randomly distributed on two positions with roughly equal occupancies (Fig. 11a). According to a schematic description, the stacking of the structure (Fig. 11b) can be described as a succession of layers containing one half of the Cs+ countercations and the water molecules for the first one and the other half of the Cs+ cations and the [Re6Si8(N3)a6]4– units for the second.
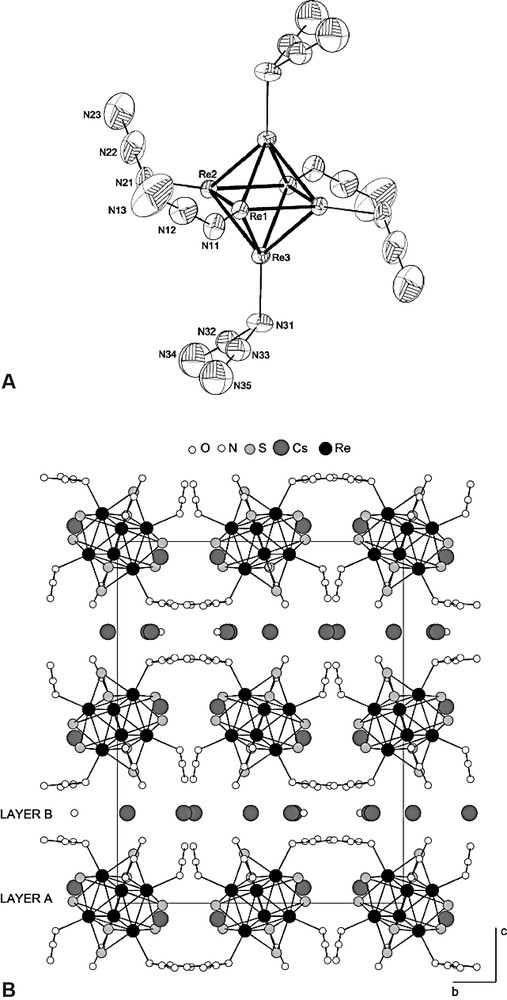
(A) Description of the Re6Si8(N3)a6 unit with four of N3– ligands which are ordered, while the two other ones are randomly distributed on two positions with roughly equal occupancies in the Cs4[Re6Si8(N3)a6]·2 H2O structure. (B) Description of the structural packing of Cs4[Re6Si8(N3)a6]·2 H2O. Projection in the (b,c) plane of the unit-cell (inner ligands omitted for clarity).
3.4 Exchange of both cations and ligands
The M6Li8(CN)a6 units mentioned above were subsequently involved in the preparation of compounds with various dimensionalities by recrystallization with 3d transition metal cations (M′ = Mn, Fe, Co, ...) through the formation of Re–CN–M′–NC–Re linkages [23]. The coordination chemistry of octahedral rhenium cluster chalcocyanide complexes is in a stage of rapid development. Interaction of these anions with aqua-cations of different transition metals [M′(H2O)6]n+ results in a partial or total replacement of their coordination water molecules by nitrogen atoms of the more nucleophilic CN ligands. As a rule such interaction leads to 3-D polymeric structures where four or all six CN ligands of the cluster anions coordinate to cations via Re–CN–M′ bridges, leading, in the latter case, to giant homothetic structures of Prussian blue [23].
The interaction of [Re6Y8(CN)6]4– anions (Y = S, Se, Te) with transition metal cations in the presence of a chelating ligand (ethylenediamine) reduces the available number of coordination sites of the transition metal ion and afforded a series of new compounds with lower dimensionality [26]:
- – (i) [{Mn(H2O)(en)2}{Mn(en)2}Re6Te8(CN)6]·3 H2O is built from a polymeric ‘zigzag’ chain where a {Mn(en)2}complex coordinated by two trans nitrogen atoms belonging to cis apical cyano ligands of the cluster unit links the latter, while the {Mn(H2O)(en)2} complex coordinates a third CN ion of each cluster unit (Fig. 12). The chains are neutral and connected to each other by hydrogen bonds through solvate water molecules;
- – (ii) [Ni(NH3)2(en)2]2[{Ni(NH3)4}Re6Se8(CN)6]–Cl2·2 H2O and its telluride homologue are isostructural, differing only by an additional water molecule in the latter. They are built from a strictly linear dianionic chain ···N≡C–[Re6Se8(CN)4]–C≡N–[Ni(NH3)4]–N≡C–[Re6Se8(CN)4]–C≡N··· of cluster units and nickel tetra-amino complexes (Fig. 13), compensated by two counter-cations Ni(NH3)2(en)2;
- – (iii) (NH4)2[{Ni(en)2}3{Re6Te8(CN)6}2]·6 H2O is built from wavy layers ···{Ni(en)2}3{Re6Te8(CN)6}2··· where each cluster is coordinated by three nickel diamine complexes while each of the latter bridges two clusters. Because these wavy layers are packed exactly one under another, they form channels extending along the c-axis of the trigonal unit-cell, with diameter varying from 4 to 7.2 Å (Fig. 14), where are hosted the ammonium counter-cations and water molecules (not shown for clarity);
- – (iv) finally, (Et4N)2[Cu(NH3)(en)2]2[{Cu(en)2}{Re6Te8(CN)6}2]·2 H2O illustrates the synthesis of the large molecular anion [{Cu(en)2}{Re6Te8(CN)6}2]6–, formed by the dimerization of cluster units via a copper ethylene diamine complex bridge, as shown in Fig. 15. Formally, the formation of these dimers can be described as resulting from the cleavage of the above linear infinite chains, may be in relation with the insertion of the large tetaethylammonium counter cation.
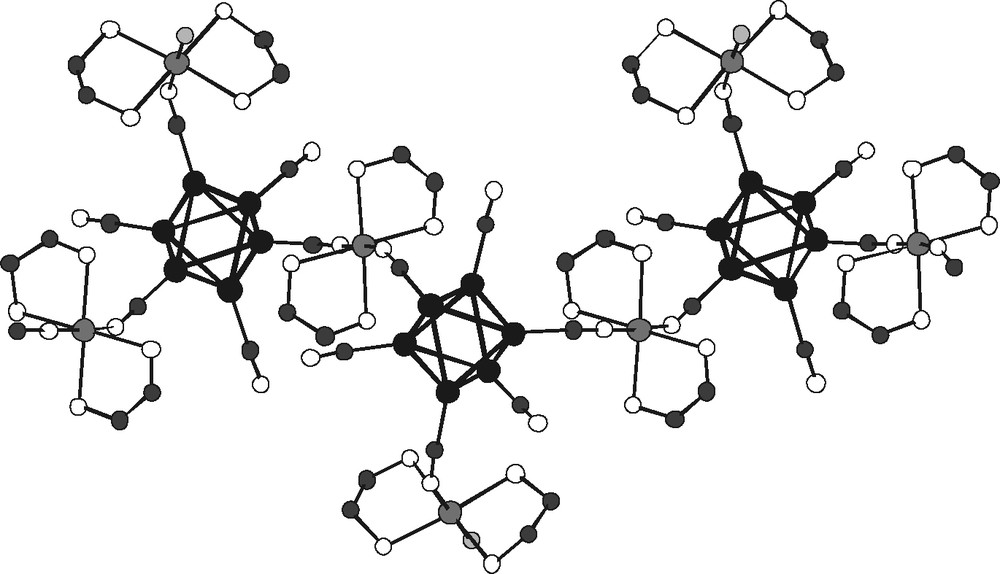
Fragment of the polymer chain {{Mn(H2O)(en)2}{Mn(en)2}Re6Te8(CN)6}∞. (all hydrogen and tellurium atoms omitted for clarity).
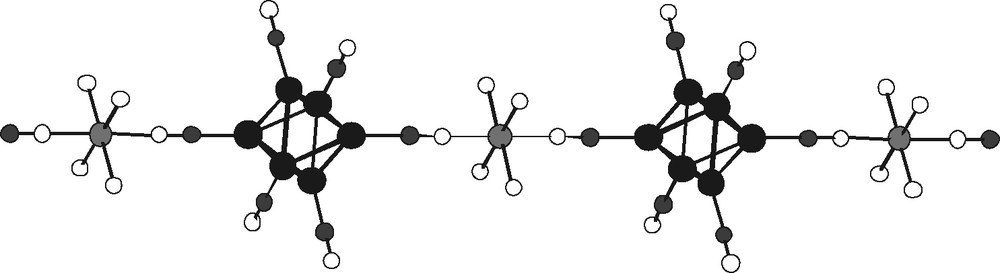
Fragment of the negatively charged linear chain {–N≡C–[Re6Se8(CN)4]–C≡N–Ni2–N≡C–[Re6Se8(CN)4]–C≡N–} (all hydrogen atoms of ammonia and chalcogen atoms of cluster core omitted for clarity).
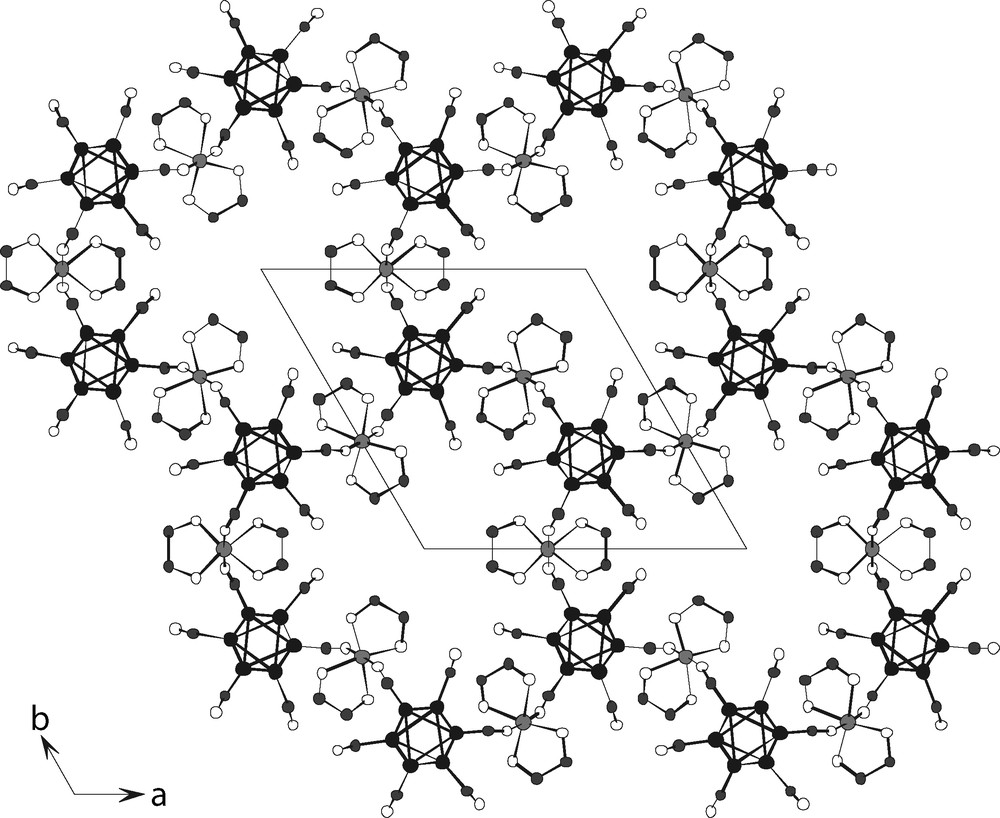
View down [0001] of the crystal packing in (NH4)2[{Ni(en)2}3{Re6Te8(CN)6}2]·6 H2O (all hydrogen and tellurium atoms, ammonium cations and water molecules omitted for clarity).
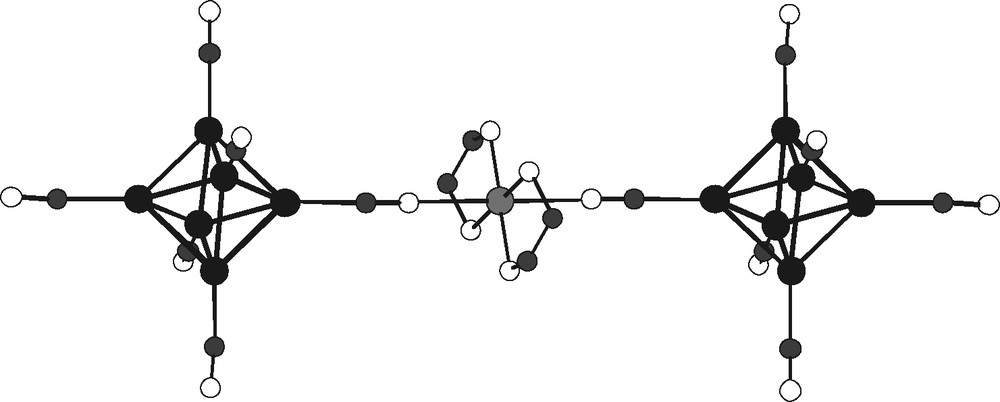
The anionic dimmer [{Cu(en)2}{Re6Te8(CN)6}2]6– (all hydrogen atoms of ethylenediamine and tellurium atoms of cluster core omitted for clarity).
4 Conclusions and perspectives
This short account illustrates the richness of the rapidly developing solution chemistry of Re6 cluster based materials. Recent approaches are oriented towards supramolecular assemblies taking advantage from substitution chemistry on the apical sites. Prominent illustrations are, for instance, molecular squares where the clusters are linked by various linear or pseudo-linear pyridyl compounds [27], act as the nucleus of dendrimers [28] or, conversely, are the arms of star-shaped multicluster molecules [29]. One of the latter examples involves a porphyrin derivative, opening a window towards biochemistry of clusters. In this context one should mention the use of clusters (up to now restricted to tantalum ones) as ‘phasers’ in order to help and improve the structure determination of proteins [30].
Acknowledgements
This work is a part of the PhD dissertation of G. Pilet and was supported by a doctoral grant from the French Ministry of Education and Research. Dr. N. Naumov is acknowledged for providing the single crystals of cyano-cluster transition-metal ethylenediamine complexes in the frame of the INTAS Grant No. 00-00689 and for helpful discussions.