1 Introduction
The structural chemistry of compounds containing reduced molybdenum has grown tremendously over the last two decades so that 22 different types of discrete molybdenum clusters, of which the nuclearities goes from 3 to 36, are known to date. Clusters with nuclearities higher than 8 result generally from the one-dimensional condensation of Mo6 clusters via opposite face- or edge-sharing depending on the ligand environment. The former process is observed when the Mo6 clusters are face-bridged by the ligands (S, Se and Te) and is exemplified, in particular, by the series of compounds Mn–2Mo3nX3n+2 (M = Rb, Cs; X = S, Se or Te; n = 3, 4, 5, 6, 7, 8, 10 and 12) containing Mo3n clusters [1–8]. The final stage of this face-sharing condensation is the infinite chain found in the quasi-one-dimensional compounds M2Mo6X6 (M = Na, K, Rb, Cs; X = S, Se or Te) [9] and Ag Mo6Te6 [44]. The edge-sharing condensation of Mo6 octahedra is observed in reduced molybdenum oxides where the Mo6 clusters are edge-bridged by the oxygen atoms. This process leads to Mo4n + 2 oligomers that are observed for example in the series Mn–xMo4n+2O6n+4 (n = 2, 3, 4 and 5) [10–21]. The ultimate step of the edge-sharing-condensation process corresponds to the infinite chain of trans-edge-sharing Mo6 octahedra that was first observed in NaMo4O6 [22]. More recently, two original high-nuclearity molybdenum clusters, i.e. Mo13 and Mo19, were observed in coexistence with the common triangular Mo3 and the planar Mo7 clusters in the atypical compound Pr4Mo9O18 [23]. Contrary to the previous high nuclearity molybdenum clusters, the Mo13 and Mo19 result, for the first time in solid-state chemistry, from the bi- or tri-dimensional condensation of octahedral Mo6 clusters, respectively. We present here the synthesis, and the crystal and electronic structures of the first compound containing only Mo13 clusters: Nd12.4Mg0.6Mo13O36.
2 Experimental section
2.1 Synthesis
Single crystals of Nd12.4Mg0.6Mo13O36 were obtained in an attempt to synthesize a phase MgNd16Mo20O56 isostructural with Nd16Mo21O56 [24]. Starting reagents were La2O3 (Rhône-Poulenc, 99.999%), MoO3 (Strem Chemicals, 99.9%) and Mo (Cimebocuze, 99.9%), all in powder form. The rare-earth oxide was prefired at 1000 °C before use and the Mo powder was heated under a hydrogen flow at 1000 °C for 6 h. The stoichiometric mixture was pressed into a ca. 5 g pellet and loaded into a molybdenum crucible (depth: 2.5 cm; diam: 1.5 cm) which was previously cleaned by heating at 1500 °C for 15 min under a dynamic vacuum of about 10–5 Torr and then sealed under a low argon pressure using an arc welding system. The crucible was heated at a rate of 300 °C h–1 to 1600 °C and held there for 3 days, then cooled at 100 °C h–1 to 1100 °C and finally furnace cooled to room temperature
2.2 Single crystal structure determination
A black crystal of dimensions 0.26 × 0.060 × 0.026 mm3 was selected for data collection. Intensity data were collected on a Nonius Kappa CCD diffractometer using a graphite-monochromatized Mo Kα radiation (λ = 0.71073 Å) at room temperature. The COLLECT program package [25] was used to establish the angular scan conditions (φ and ω scans) used in the data collection. The data set was processed using EvalCCD [26] for the integration procedure. An absorption correction (Tmin = 0.01131, Tmax = 0.2315) was applied using the description of the crystal faces and the analytical method described by de Meulenaar and Tompa [27]. The structure was solved by direct methods using Sir97 [28] and subsequent difference Fourier syntheses in the space group R3 m. All structure refinements and Fourier syntheses were carried out using SHELXL-97 [29]. Refinement of the occupancy factors of the Nd sites showed that the Nd2, Nd3 and the Nd5 sites are slightly deficient and occupy at 98.6 (4)%, 0.972 (4)% and 0.98 (5)%, respectively. As qualitative microanalyses using a JEOL JSM-35 CF scanning electron microscope equipped with a Tracor energy-dispersive-type X-ray spectrometer indicated the presence of magnesium in the crystals, we could expect that the deficiencies observed on some of the Nd sites results from the presence of magnesium since in the final difference Fourier map we did not observed peaks compatible with Mg positions. Refinements taking into account an occupation of the deficient Nd sites simultaneously by Nd and Mg atoms were unsuccessful. However, if assume a complete occupation of the Nd2, Nd3 and Nd5 sites by Nd and Mg atoms, we get the composition Nd12.4(1)Mg0.6(1)Mo13O36 which is in good agreement with that found by I.C.P. measurement, Nd12.5(3)Mg0.51(5)Mo13.11(4)O36. The positional and anisotropic displacement parameters for all atoms as well as the occupancy factors for the Nd atoms were refined to the values R1 = 0.0311, wR2 = 0.0782 for 126 parameters and 3184 reflections with I > 2 σ(I) and the residual electron densities were 3.310 and –3.517 e Å–3. A summary of the X-ray crystallographic and experimental data are presented in Table 1, and selected interatomic distances are reported in Table 2.
Experimental table
Chemical formula | Nd12.4Mg0.6Mo13O36 |
Mr | 3671.42 |
Cell setting, space group | Trigonal, R3m |
a, c (Å) | 11.3103 (1), 21.7465 (3) |
V (Å3) | 2409.17 (4) |
Z | 3 |
Dx (Mg m−3) | 7.592 |
Radiation type | Mo Kα |
Number of reflections for cell parameters | 16,985 |
θ Range (°) | 1.0–37.8 |
μ (mm−1) | 24.62 |
Temperature (K) | 293 (2) |
Crystal form, color | Truncated hexagonal block, black |
Crystal size (mm) | 0.26 × 0.06 × 0.03 |
Diffractometer | KappaCCD |
Data collection method | φ and ω scans |
Absorption correction | Analytical |
Tmin | 0.011 |
Tmax | 0.232 |
Number of measured, independent and observed parameters | 23,571, 3203, 3184 |
Criterion for observed reflections | I > 2σ(I) |
Rint | 0.072 |
θmax (°) | 37.8 |
Range of h, k, l | –18 → h → 19/–19 → k → 19/–37 → l → 37 |
Refinement on | F2 |
R[F2 > 2σ(F2)], wR(F2), S | 0.031, 0.078, 1.09 |
Number of reflections | 3203 reflections |
Number of parameters | 126 |
Weighting scheme | Calculated w = 1/[σ2(Fo2) + (0.0354 P)2 + 109.0437 P] where P = (Fo2 + 2 Fc2)/3 |
(Δ/σ)max | 0.095 |
Δρmax, Δρmin (e Å−3) | 3.31, –3.52 |
Extinction method | SHELXL |
Extinction coefficient | 0.000255 (19) |
Selected interatomic distances (Å) for Nd12.4Mg0.6Mo13O36
Nd1–O2 (×6) | 2.832 (5) | Nd4–O3 | 2.836 (8) |
Nd1–O4 | 2.339 (11) | Nd4–O4 | 2.373 (4) |
Nd1–O5 (×3) | 2.406 (7) | Nd4–O6 (×2) | 2.345 (5) |
Nd2/Mg–O1 (×2) | 2.773 (4) | Nd4–O7 (×2) | 2.883 (5) |
Nd2/Mg–O10 | 2.345 (9) | Nd4–O8 (×2) | 2.400 (5) |
Nd2/Mg–O11 | 2.148 (2) | Nd5/Mg–O10 | 2.318 (11) |
Nd2/Mg–O2 (×2) | 2.437 (5) | Nd5/Mg–O5 (×2) | 2.330 (4) |
Nd2–O6 (×2) | 2.441 (5) | Nd5/Mg–O6 (×2) | 2.329 (5) |
Nd3/Mg–O1 | 2.903 (6) | Nd5/Mg–O9 | 2.928 (7) |
Nd3/Mg–O10 (×2) | 2.316 (6) | Nd5′/Mg–O10 | 2.834 (19) |
Nd3/Mg–O7 | 2.332 (6) | Nd5′/Mg–O5 (×2) | 2.214 (9) |
Nd3/Mg–O8 (×2) | 2.464 (5) | Nd5′/Mg -O6 (×2) | 2.200 (9) |
Nd3/Mg–O9 (×2) | 2.633 (5) | ||
Mo1–Mo2 (×6) | 2.7324 (5) | Mo4–Mo3 | 2.7567 (6) |
Mo2–Mo2 | 2.6918 (10) | Mo1–Mo4 (×3) | 2.8478 (12) |
Mo2–Mo2 | 2.7715 (9) | Mo2–Mo4 | 2.7858 (7) |
Mo3–Mo2 (×2) | 2.7137 (7) | Mo3–Mo4 | 2.7566 (6) |
Mo4–Mo2 | 2.7858 (7) | Mo3–Mo4 | 2.7566 (6) |
Mo2–Mo3 | 2.7137 (7) | Mo4–Mo4 (×2) | 2.7471 (12) |
Mo4–Mo3 | 2.7566 (6) | ||
Mo1–O9 (×3) | 2.128 (7) | Mo3–O2 (×2) | 1.969 (5) |
Mo2–O1 | 2.135 (5) | Mo3–O5 | 2.101 (6) |
Mo2–O2 | 2.076 (5) | Mo3–O8 (×2) | 2.065 (5) |
Mo2–O6 | 2.043 (5) | Mo4–O1 | 2.119 (6) |
Mo2–O7 | 2.079 (5) | Mo4–O3 | 2.079 (8) |
Mo2–O9 | 2.122 (5) | Mo4–O8 (×2) | 2.105(5) |
2.3 Theoretical calculations
Extended Hückel [30] molecular calculations were carried out using the program CACAO [31]. The exponents (ζ) and the valence shell ionization potentials (Hii in eV) used were, respectively: 2.275, –32.3 for O 2s; 2.275, –14.8 for O 2p; 1.956, –8.34 for Mo 5s; 1.921, –5.24 for Mo 5p; 2.14. The Hii value for Mo 4d was set equal to –10.50. A linear combination of two Slater-type orbitals of exponents ζ1 = 4.542 and ζ2 = 1.901 with equal weighting coefficients was used to represent the Mo 4d atomic orbitals.
Self-consistent ab initio band structure calculations were performed on the model compound Nd13Mo13O36 with the scalar relativistic tight-binding linear muffin-tin orbital (LMTO) method in the atomic spheres approximation including the combined correction [32–36]. Exchange and correlation were treated in the local density approximation using the von Barth and Hedin [37] local exchange correlation potential. Within the LMTO formalism interatomic spaces are filled with interstitial spheres. The optimal positions and radii of these additional ‘empty spheres’ were determined by the procedure described in Ref. [38]. Twenty-four non-symmetry-related ‘empty spheres’ with 0.62 Å ≤ rES ≤ 1.60 Å were introduced for the calculations. The full LMTO basis set consisted of 6s, 6p, 5d and 4f functions for Nd spheres, 5s, 5p, 4d and 4f functions for Mo spheres, 2s, 2p and 3d functions for O spheres, and s, p and d functions for ‘empty spheres’. The eigenvalue problem was solved using the following minimal basis set obtained from the Löwdin downfolding technique: Nd 6s, 5d, 4f, Mo 5s, 5p, 4d, O 2s, 2p and interstitial 1s LMTOs. The k space integration was performed using the tetrahedron method [39]. Charge self-consistency and the average properties were obtained from 46 irreducible k points. A measure of the magnitude of the bonding was obtained by computing the crystal orbital Hamiltonian populations (COHP) which are the Hamiltonian population weighted density of states (DOS) [40]. As recommended [41], a reduced basis set (in which all ES LMTO's have been downfolded) was used for the COHP calculations. DOS and COHP curves are shifted so that εF lies at 0 eV.
3 Results and discussion
3.1 Structure description
A perspective view of the crystal structure of Nd12.4Mg0.6Mo13O36 along the b axis is represented in Fig. 1. It shows that this structure can be viewed as a stacking along the c-axis of two different slabs interconnected through oxygen atoms. The A slab is made up of molybdenum forming Mo13 clusters, neodymium and oxygen atoms, and the B slab of neodymium and oxygen atoms.

A perspective view of the crystal structure of Nd12.4Mg0.6Mo13O36 along the b axis.
In the A slab, the Mo13 cluster is similar to that encountered in the compound Pr4Mo9O18. It has 3 m (C3v) symmetry and may be regarded as resulting from the edge-sharing-condensation of three Mo6 octahedra having the same apex in common, as depicted in Fig. 2. The Mo–Mo bond distances within the Mo13 cluster range from 2.692(1) to 2.848(1) Å with an average value of 2.74 Å and are thus quite comparable to those found in one-dimensional condensed Mo clusters. The molybdenum atoms are linked to three (Mo1), four (Mo4) or five (Mo2, Mo3) oxygen atoms. These bridge the Mo-Mo edges (O2, O7, O8), cap the triangular Mo faces (O1, O4, O9) or are bonded exo to the Mo apices of the Mo13 polyhedron (O5, O6) leading to the Mo13O31 unit shown in Fig. 3. The Mo–O bond distances lie between 1.969(5) and 2.135(5) Å with an average value of 2.08 Å. In the A slab, we also find the neodymium atoms Nd1 and Nd2 that are surrounded by 10 and 8 oxygen atoms, respectively. The coordination polyhedra around both neodymium atoms are shown in Fig. 4. The Nd1O10 polyhedron may be described as the stacking of a triangle formed by the O5 on a hexagon of O2 atoms which is capped by the O4 atom. The environment of the Nd2 atoms may be viewed as a distorted bicapped trigonal prism. The Nd–O distances range from 2.339(11) to 2.832(5) for the Nd1 site and from 2.148(2) to 2.774(4) for the Nd2 site. The arrangement of the Mo13O31 units and the Nd1O10 and Nd2O8 polyhedra in the A slab is shown in Fig. 5.
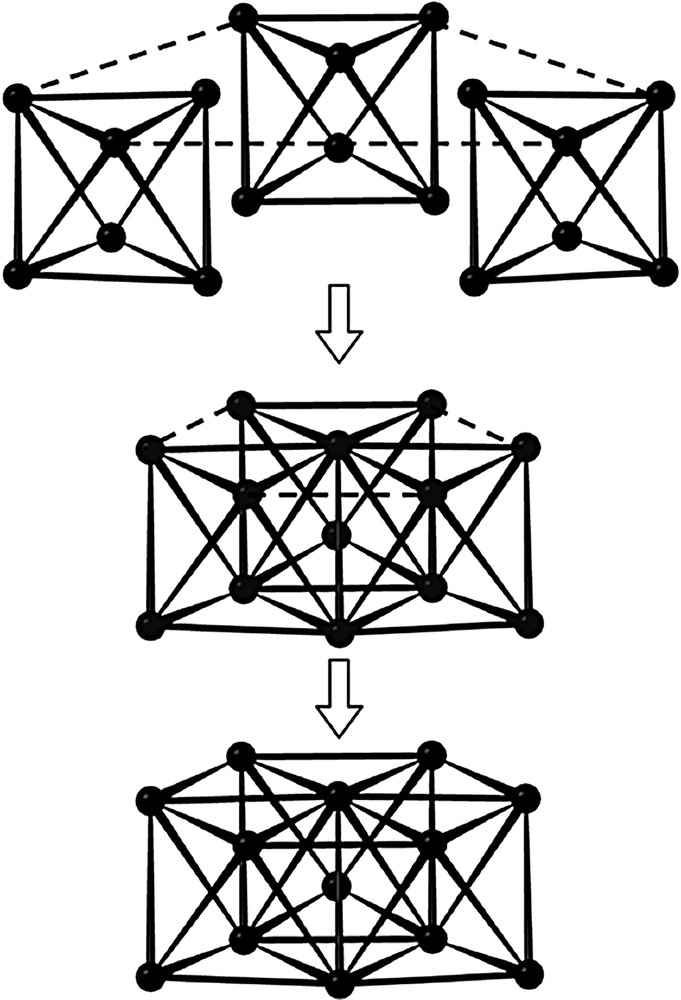
Condensation process for the Mo13 cluster.

The Mo13 cluster with its oxygen environment (97% probability ellipsoids).
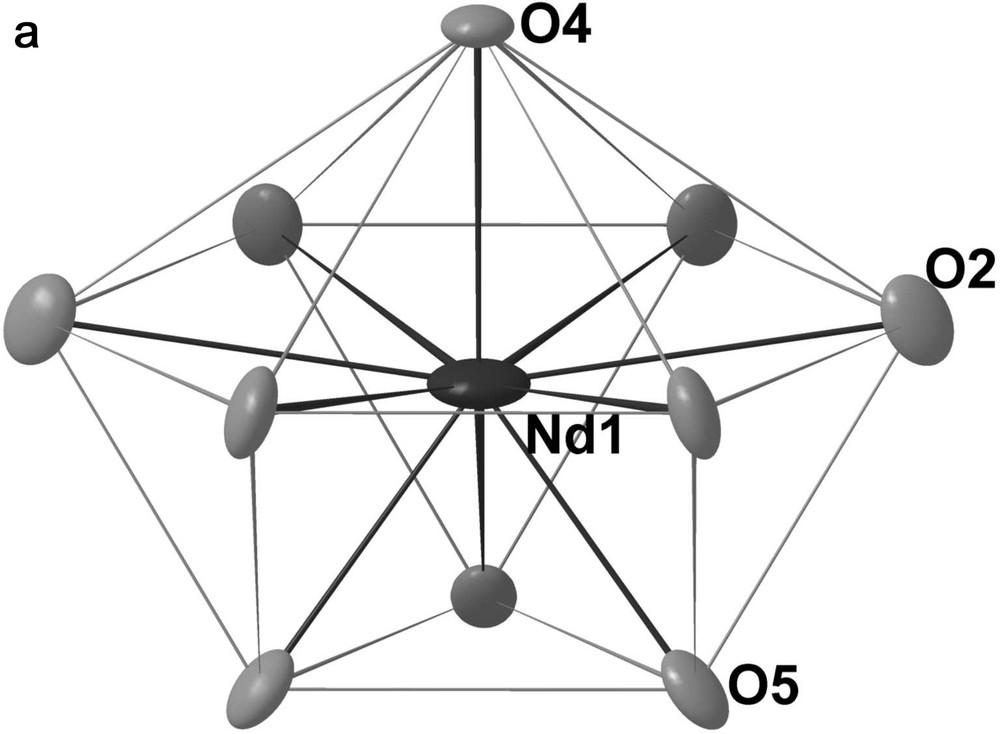
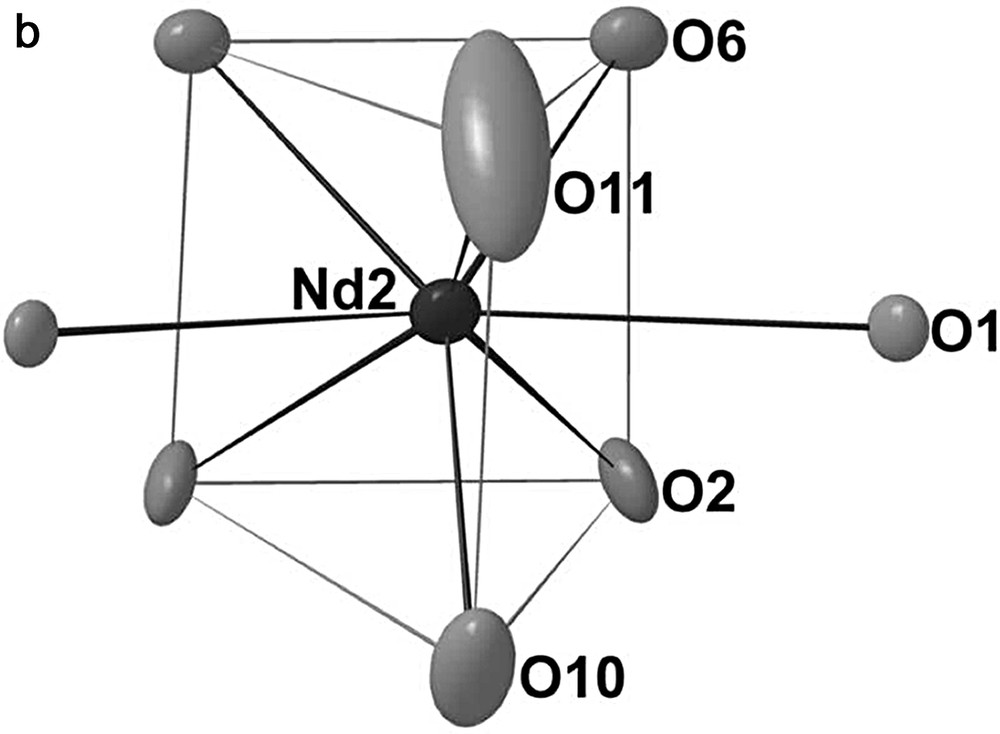
Oxygen environments for the Nd1 and Nd2 atoms.
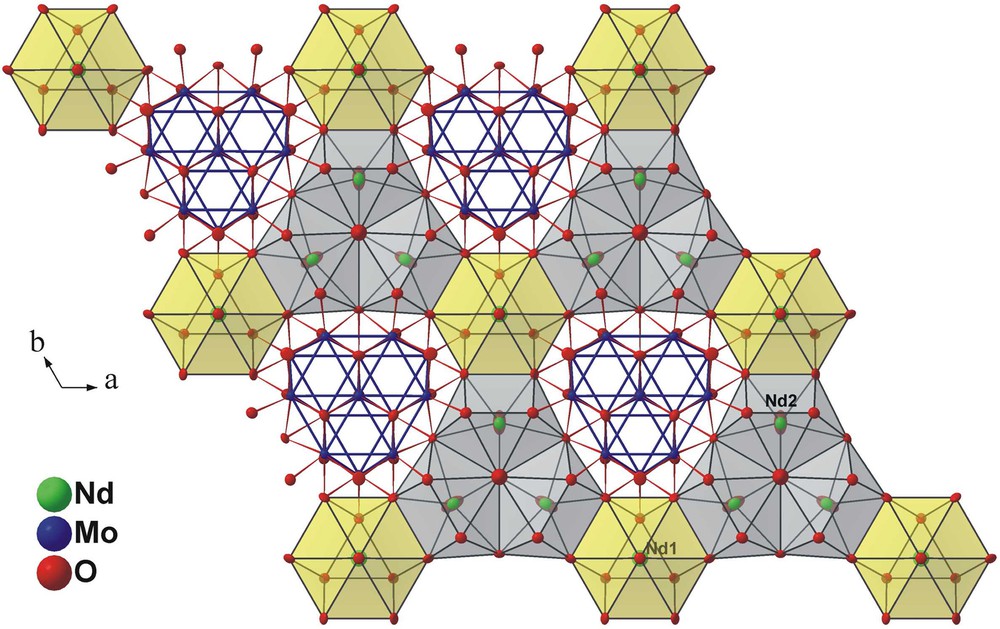
A polyhedral representation of the A slab viewed along the c axis.
In the B slab, we find the remaining Nd atoms, i.e. Nd3, Nd4, Nd5 and Nd5′ (Fig. 6). The Nd3 and Nd4 atoms are eight-coordinate in oxygen but present different environments. Nd3has a bicapped trigonal prismatic environment and Nd4 is at the center of a tricapped square-based pyramid. The Nd3–O bond distances lie between 2.331(6) and 2.904(6) Å and the Nd4–O between 2.345(5) and 2.883(5) Å. The last Nd atom is delocalized over two the two positions Nd5 and Nd5′ in a monocapped square-based pyramid. The Nd5–O and Nd5′–O distances vary between 2.313(11) and 2.927(7) Å and between 2.210(17) and 2.750(10) Å, respectively. The Fig. 7 shows the arrangement of the Nd3O8, Nd4O8 and (Nd5, Nd5′) O6 polyhedra within the B slab.

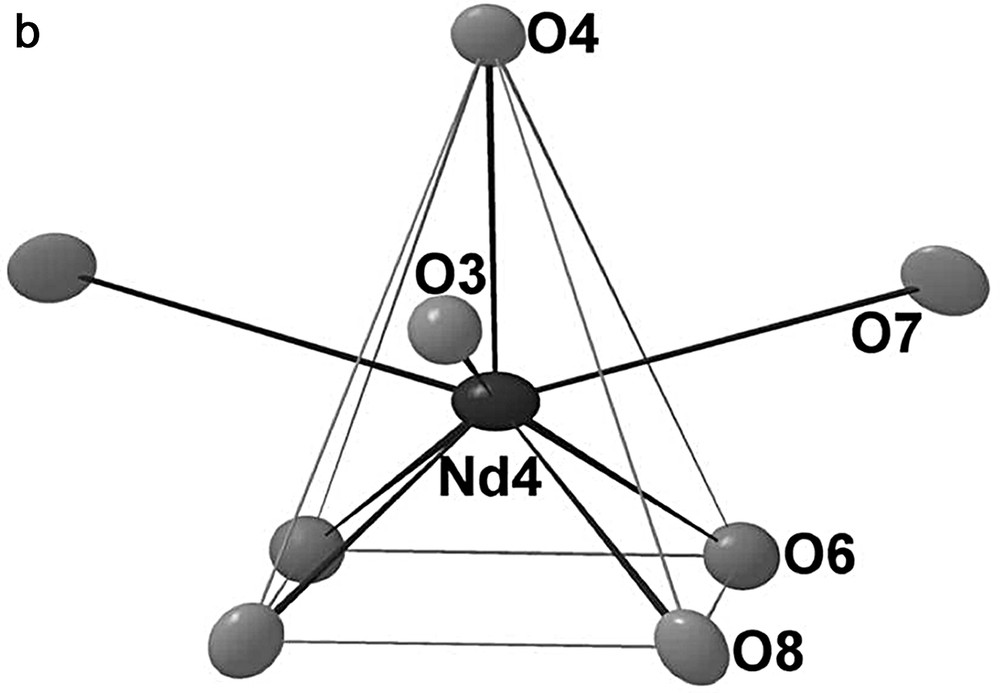
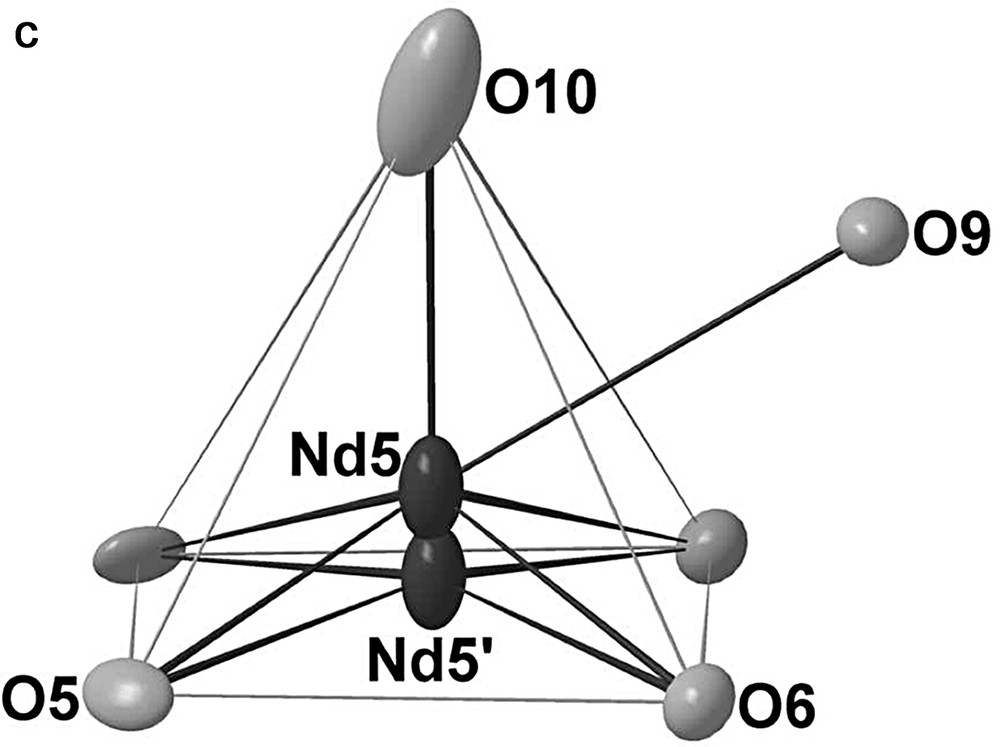
Oxygen environments for the Nd3, Nd4 and Nd5 atoms.

A polyhedral representation of the B slab viewed along the c axis.
3.2 Bond-length bond-strength formula
The estimation of the oxidation states of molybdenum and, consequently, of the number of electrons per Mo13 cluster could be performed on this compound using the empirical bond length-bond strength relationship developed by Brown and Wu [42] for Mo–O bonds:
In the above formula, s(Mo–O) is the bond-strength in valence units, d(Mo–O) is the observed Mo–O bond distance in Å, 1.882 Å corresponds to a Mo–O single bond distance, and the exponential parameter-6 is characteristic of the Mo atom. Thus, the sum of the Mo–O bond strengths s (in valence units) about a particular Mo atom is equal to the oxidation state of that Mo atom. For the four crystallographically independent Mo atoms, these calculations lead to the number oxidation states of +1.44(2), 2.67(4), 3.19(5) and +2.06(3) for Mo1, Mo2, Mo3 and Mo4, respectively. From these values, we could estimate the metallic electron (ME) count per Mo13 cluster to 44.7(4), which is in good agreement with the value of 44.4(3) e– based on the stoichiometry.
3.3 Electronic structure
Extended Hückel calculations were performed on the experimental Mo13O31 cluster of C3v symmetry. The corresponding molecular orbital (MO) diagram is shown in Fig. 8. Because of the electronegativity difference of molybdenum and oxygen atoms, MOs that lie in the HOMO/LUMO part of MO diagram shows a major metallic d character. The 48e, 49e and 33a1 MOs show an overall Mo–Mo non-bonding character as revealed by the metal–metal overlap populations examination, whereas lower and higher metallic MOs are Mo–Mo bonding and antibonding, respectively. The 48e, 49e and 33a1 MOs become the HOMO for the ME count of 42, 46, and 48, respectively. No well-defined HOMO/LUMO gaps are observed for those ME counts. Such a situation has been frequently encountered for metal clusters with a high metal–metal connectivity [43]. Spin-polarized periodic density functional (DF) calculations were carried out on the model compound Nd13Mo13O36 where no Mg atoms are considered and all Nd5 and Nd5′ positions are fully occupied and vacant, respectively. Since no close metal–metal contacts are observed between the Mo13 clusters in the crystal, no significant perturbation of the Mo13 clusters' MO pattern is expected in the three-dimensional solid. Spin-polarized total, Nd and Mo projected DOS and Mo–Mo COHP are sketched in Fig. 9. The Fermi level cuts a spin-up DOS peak mainly centered on Nd 4f. Since three electrons occupy this DOS peak inducing a + 3 oxidation state for the Nd atoms, the ME count of the Mo13 cluster is equal to 45. The Fermi level cuts also a rather large Mo projected spin-up DOS peak and a narrow Mo projected spin-down DOS peak that are both weakly Mo–Mo antibonding. Theses bands are separated by an energy of more than half an eV from lower Mo–Mo bonding bands. Assuming a rigid band model, this gap becomes the band gap for the ME count of 42. Although some Mo–Mo antibonding bands are occupied, the C3v symmetry architecture is preserved for this cluster because of the high connectivity of the different atoms constituting the cluster. Periodic DF calculations suggest that an open-shell configuration is encountered for the Mo13O31 unit in this model compound. Because of the absence of significant HOMO/LUMO gaps, strong Jahn–Teller distortions of the cluster could occur for the low spin state configuration that is less stable than the high spin state. Magnetic properties consistent with the presence of Nd3+ and some polarized electrons on Mo atoms are expected.

Extended Hückel molecular orbital diagram of Mo13O31.
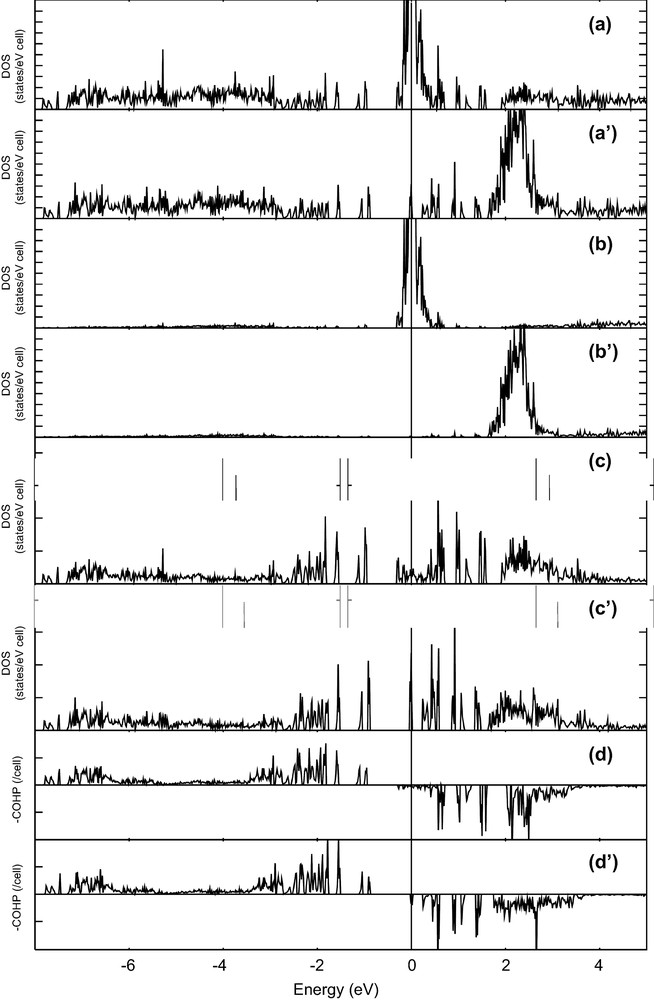
Spin-polarized DF calculations for the model compound Nd13Mo13O36: (a) spin-up and (a′) spin-down total DOS, (b) spin-up and (b′) spin-down Nd projected DOS, (c) spin-up and (c′) spin-down Mo projected DOS, (d) spin-up and (d′) spin-down averaged COHP for Mo–Mo bonds of the cluster.
4 Supplementary material
Further details of the crystal structure investigation may be obtained from the Fachinformationszentrum Karlsruhe, D-76344 Eggenstein-Leopoldshafen, Germany (Fax: +49 7247 808 666; e-mail: crysdata@fiz-karlsruhe.de), on quoting the depository number CSD- 414275.