1 Introduction
The mesoporous materials with different compositions, new pore systems, and novel properties have attracted considerable attention because of their remarkably large surface areas and narrow pore size distributions, which make them ideal candidates for catalysis [1]. Recently, much attention has been directed to the synthesis of nanostructured mesoporous oxides using surfactant templating method. It has been developed for the synthesis of materials with a narrow mesopore size distribution and controlled pore structure [2–4]. It is generally anticipated that the use of a high surface area mesoporous oxide support, rather than a commercial, low surface area support, for noble metals or transition metals has some beneficial effects on the catalytic performance [5]. The mesoporous support would give rise to well dispersed and stable metal particles on the surface upon thermal treatments and as a consequence would also show an improved catalytic efficiency.
The interest in platinum-loaded titania (Pt/TiO2), has rapidly increased due to their high activity in a multitude of important reactions [6–9]. Among them, photocatalytic H2 generation from water splitting has recently been attracting rapidly growing interest due to clean, storable, and renewable energy development, aiming to replace exhausting fossil fuels by use of solar energy ever since Fujishima and Honda’s report on the photoelectrochemical water splitting by TiO2 electrode [10]. The photocatalytic activity and especially the stability of platinum strongly depend on both the state and structure of the support and the specific interaction between platinum and support. The type of the support is of crucial importance to obtain highly dispersed platinum particles with good performance [11]. To our knowledge, it has been suggested a good possibility to apply the mesoporous TiO2 for the first time as a support for platinum towards the photocatalytic reaction of H2 evolution.
In our previous articles, the mesoporous TiO2 photocatalyst synthesized via a surfactant-assisted templating sol–gel process was reported [12,13] and used as a support for nickel oxide loaded by single-step sol–gel method [14]. In this work, we present a considerably high photocatalytic performance over platinum supported on such the mesoporous TiO2. The methods of platinum loading namely single-step sol–gel (SSSG), incipient wetness impregnation (IWI), and photochemical deposition (PCD) and their characterizations by XRD, N2 adsorption–desorption analysis, and TEM were described. The photocatalytic H2 evolution activity of the photocatalysts with different platinum contents was tested systematically.
2 Experimental
2.1 Materials
Tetraisopropyl orthotitanate (TIPT, Tokyo Chemical Industry Co., Ltd.), hydrogen hexachloroplatinate(IV) hydrate (Nacalai Tesque, Inc.), laurylamine hydrochloride (LAHC, Tokyo Chemical Industry Co., Ltd), acetylacetone (ACA, Nacalai Tesque, Inc.) and methanol (Nacalai Tesque, Inc.) were used for this study. All chemicals were analytical grade and used without further purification. LAHC was used as a surfactant template behaving as a mesopore-directing agent. ACA serving as a modifying agent was applied to moderate the hydrolysis and condensation processes of titanium precursor.
2.2 Photocatalyst synthesis
Single-step sol–gel (SSSG) made Pt-loaded mesoporous TiO2 photocatalyst was synthesized via a combined sol–gel with surfactant-assisted templating mechanism in a LAHC/TIPT modified with ACA system. In typical synthesis, a specified amount of analytical grade ACA was first introduced into TIPT with the same mole. The mixed solution was then gently shaken until intimate mixing. Afterwards, 0.1 M LAHC aqueous solution of pH 4.2 was added into the ACA-modified TIPT solution, in which the molar ratio of TIPT to LAHC was adjusted to a value of 4. The mixture was kept continuously stirring at 40 °C for 10 h to obtain transparent yellow sol. To the aged TiO2 sol solution, a necessary amount of hydrogen hexachloroplatinate(IV) hydrate in methanol for desired Pt loading of 0.1–0.9 wt% was incorporated, and the final mixture was further aged at 40 °C for 5 days to acquire homogeneous solution. Then, the gel was formed by placing the sol-containing solution into an oven kept at 80 °C for a week. Subsequently, the gel was dried overnight at 80 °C to eliminate the solvents. The dried sample was calcined at 500 °C for 4 h to remove LAHC template and consequently produce the desired photocatalyst. For comparative study, incipient wetness impregnation (IWI) and photochemical deposition (PCD) methods were used to load Pt onto the mesoporous TiO2 support prepared by the same sol–gel method described above. For IWI method, the support was impregnated with an appropriate amount of hydrogen hexachloroplatinate(IV) hydrate aqueous solution, dried at 80 °C, and finally calcined at 500 °C for 4 h. For PCD method, the support was first dispersed in distilled water, ultrasonicated, and charged to the photoreactor. Subsequently, required amounts of hydrogen hexachloroplatinate(IV) hydrate aqueous solution, methanol, and distilled water were added to obtain 50 vol% aqueous methanol solution. The mixture was magnetically stirred and irradiated with a 300 W high-pressure Hg lamp for 1 h. After irradiation, the deposited TiO2 particles were recovered by filtration, washed with hot distilled water, and dried at 80 °C.
2.3 Photocatalyst characterizations
X-ray diffraction (XRD) was used to identify phases present in the samples. A Rigaku RINT-2100 rotating anode XRD system generating monochromated Cu Kα radiation with continuous scanning mode at rate of 2°min–1 and operating conditions of 40 kV and 40 mA was used to obtain XRD patterns. A nitrogen adsorption system (BEL Japan BELSORP-18 Plus) was employed to measure adsorption–desorption isotherms at liquid nitrogen temperature of 77 K. The Brunauer–Emmett–Teller (BET) approach using adsorption data over the relative pressure ranging from 0.05 to 0.35 was utilized to determine surface area. The Barrett–Joyner–Halenda (BJH) approach was used to yield pore size distribution from desorption data. The samples were degassed at 200 °C for 2 h to remove physisorbed gases prior to the measurement. The sample morphology was observed by a transmission electron microscope (TEM, JEOL JEM-200CX) operated at accelerating voltage of 200 kV.
2.4 Photocatalytic activity measurement
Photocatalytic H2 evolution reaction was performed in a closed gas-circulating system. A specified amount of photocatalyst (0.2 g) was suspended in an aqueous methanol solution (200 ml of distilled water, 20 ml of CH3OH) by means of magnetic stirrer within an inner irradiation reactor made of Pyrex glass. A 300 W high-pressure Hg lamp was utilized as the light source. Prior to the reaction, the mixture was deaerated by purging with Ar gas repeatedly. To avoid the heating of the solution during the courses of reaction, water was circulated through a cylindrical Pyrex jacket located around the light source. The gaseous H2 evolved was periodically analyzed by an on-line gas chromatograph (Shimadzu GC-8A, Molecular sieve 5 A, Argon gas), which was connected with a circulation line and equipped with thermal conductivity detector (TCD).
3 Results and discussion
3.1 Photocatalyst characterizations
The XRD patterns of the Pt/TiO2 synthesized by the three methods are shown in Fig. 1a–c. The diffractograms mainly belong to the crystalline structure of anatase TiO2. The presence of Pt could be observed at high loading concentration at diffraction angle of 39.8° indexed to (111) plane, however the peak intensity is relatively weak, presumably due to the combination of its low content and small particle size. The crystallite size of the samples estimated from line broadening of anatase (101) diffraction peak using Sherrer equation is shown in Table 1. The results revealed that the crystallite size of photocatalysts prepared by the three methods is in the range of 10–13 nm. Although significant difference in crystallite size was not observed, the crystallite size of the samples prepared by IWI and PCD methods is in average slightly larger than that of the SSSG samples. This stemmed from the stabilization of the TiO2 support at 500 °C before the impregnation and photochemical deposition processes, respectively.
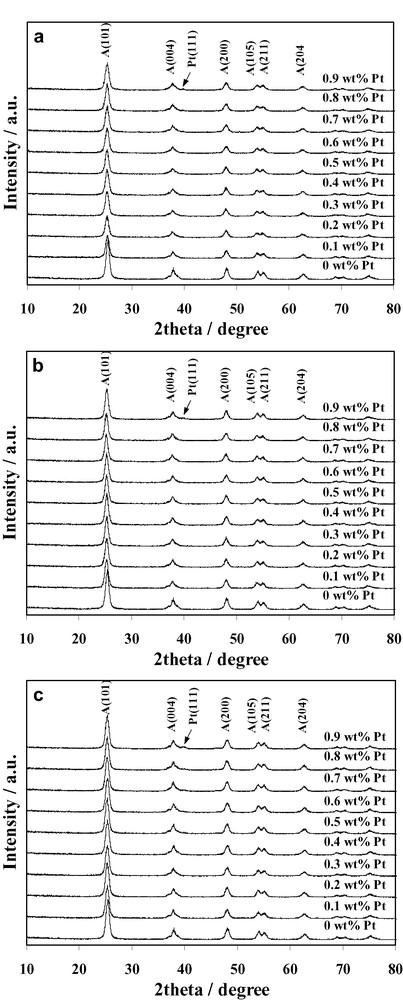
XRD patterns of Pt/TiO2 photocatalysts prepared by (a) SSSG, (b) IWI, and (c) PCD methods at various Pt loadings (A: Anatase).
Crystallite size of photocatalysts from XRD analysis
Pt loading/ wt% | Crystallite sizea/nm | |||
Unloaded TiO2 (support) | SSSG Pt/TiO2 | IWI Pt/TiO2 | PCD Pt/TiO2 | |
0 | 11.1 | – | – | – |
0.1 | – | 12.1 | 12.0 | 13.4 |
0.2 | – | 11.7 | 12.0 | 13.1 |
0.3 | – | 11.7 | 12.3 | 12.3 |
0.4 | – | 11.5 | 12.3 | 12.2 |
0.5 | – | 11.7 | 12.6 | 12.0 |
0.6 | – | 10.9 | 12.4 | 12.8 |
0.7 | – | 12.1 | 12.2 | 12.4 |
0.8 | – | 12.0 | 12.7 | 13.0 |
0.9 | – | 11.5 | 12.4 | 13.1 |
a Estimated from line broadening of anatase (101) diffraction peak using Sherrer formula.
Fig. 2 depicts the nitrogen adsorption–desorption isotherms and pore size distributions of the unloaded TiO2 and 0.6 wt% Pt/TiO2 prepared by SSSG method, which displayed the highest photocatalytic activity for H2 evolution as explained later. The isotherms exhibit typical type IV pattern with hysteresis loop, characteristic of mesoporous material according to the classification of IUPAC [15]. A sharp increase in adsorption volume of N2 was observed and located in the P/P0 range of 0.45–0.80. This sharp increase can be imputable to the capillary condensation, indicating the good homogeneity of the sample and fairly small pore size since the P/P0 position of the inflection point is related to the pore size. The pore size distribution obtained with BJH method (see inset of Fig. 2) is noticeably narrow, confirming good quality of the sample.
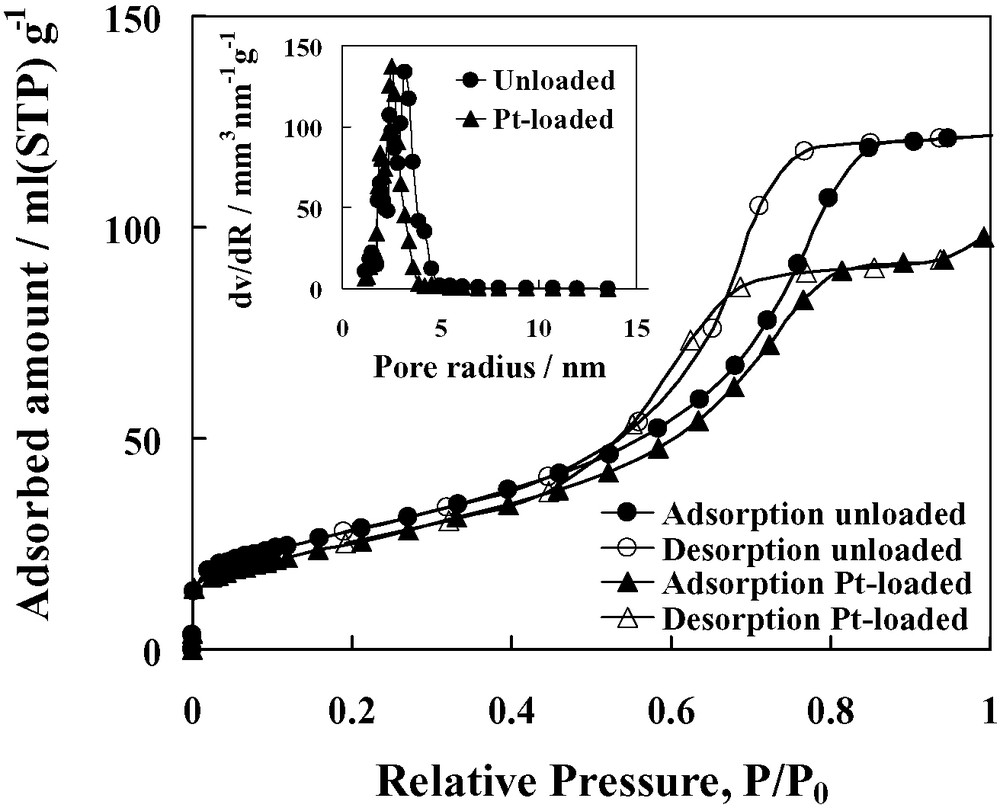
N2 adsorption–desorption isotherms and pore size distributions (inset) of unloaded TiO2 and 0.6 wt% Pt/TiO2 prepared by SSSG method.
The textural properties of the photocatalysts prepared by the three methods are given in Table 2. The higher the Pt content was loaded, the larger the decrease in the BET surface area of the samples for all methods was observed. In the investigated range of Pt loading content, the surface area obtained from the different methods decreased in the following order: SSSG > IWI > PCD. The mean pore diameter of the IWI and PCD photocatalysts remained almost identical to that of the TiO2 support, while the total pore volume decreased in similar trend to the surface area. It may be concluded that such the small amount of loaded Pt disturbed the mesopore volume by depositing at the outer and near the outer surface of the TiO2 aggregates, while maintaining the general pore size characteristic. However, it is surprisingly found that the mean pore diameter of the SSSG photocatalysts fairly decreased with the increased amount of Pt loading and was lower than that of the IWI and PCD ones. This implied that in case of the SSSG method, the loaded Pt might play a significant role during the TiO2 matrix formation. In sol–gel process, it is well known that the loaded metal incorporates into the matrix of support. However, in this investigated SSSG system, the Pt precursor was added after the complete hydrolysis of TIPT during the preparation. Most of Pt crystallites are, therefore, expected to deposit on the surface of the TiO2 throughout the mesopore network (some of which were inevitably buried inside the TiO2). By considering the diameter of micellar LAHC hydrophobic part of approximately 3.34 nm, the mean pore diameter of the SSSG samples (see Table 2) seems to get in closer proximity with increasing the Pt content as compared with the other two methods, in which bare TiO2 from the same sol–gel process was used for Pt loading. This also implies that introducing Pt precursor into the completely hydrolyzed TiO2 sol by the SSSG method can bring about the thermal stability of mesoporous framework during the crystallization of the walls separating mesopores upon thermal treatment, subsequently resulting in the less loss of surface area.
Textural properties of photocatalysts from N2 adsorption–desorption analysis
Photocatalyst | Pt loading/wt% | BET surface area/m2 g−1 | Mean pore diameter/nm | Total pore volume/cm3 g−1 |
Unloaded TiO2 (support) | – | 100.9 | 6.34 | 0.216 |
SSSG Pt/TiO2 | 0.1 | 92.2 | 6.34 | 0.189 |
0.2 | 91.6 | 5.34 | 0.184 | |
0.3 | 89.5 | 5.06 | 0.182 | |
0.4 | 91.2 | 5.34 | 0.182 | |
0.5 | 87.9 | 5.34 | 0.178 | |
0.6 | 89.0 | 5.06 | 0.162 | |
0.7 | 87.1 | 4.82 | 0.161 | |
0.8 | 87.0 | 4.82 | 0.154 | |
0.9 | 83.3 | 4.36 | 0.144 | |
IWI Pt/TiO2 | 0.1 | 85.9 | 7.22 | 0.199 |
0.2 | 84.7 | 6.34 | 0.200 | |
0.3 | 82.7 | 7.22 | 0.190 | |
0.4 | 79.7 | 6.76 | 0.190 | |
0.5 | 81.7 | 6.34 | 0.189 | |
0.6 | 79.1 | 6.76 | 0.188 | |
0.7 | 79.7 | 5.96 | 0.185 | |
0.8 | 78.7 | 6.34 | 0.179 | |
0.9 | 76.4 | 5.96 | 0.168 | |
PCD Pt/TiO2 | 0.1 | 84.0 | 5.96 | 0.192 |
0.2 | 71.7 | 6.34 | 0.175 | |
0.3 | 70.7 | 6.34 | 0.180 | |
0.4 | 65.6 | 6.76 | 0.163 | |
0.5 | 62.7 | 6.76 | 0.160 | |
0.6 | 60.8 | 6.34 | 0.158 | |
0.7 | 55.1 | 6.34 | 0.154 | |
0.8 | 57.1 | 6.76 | 0.152 | |
0.9 | 52.7 | 6.34 | 0.153 |
TEM images of the Pt/TiO2 prepared by the three methods (at the Pt loading content, which showed the highest photocatalytic activity for each method) are shown in Fig. 3a–c. It is made evident that the nanocrystalline TiO2 aggregates was formed with three-dimensional disordered primary particles. The observed TiO2 particle size of 10–15 nm is in good accordance with the crystallite size estimated from XRD, elucidating that each grain can be reliably considered in average as a single crystallite. From visualization of the images, there are a few dark patches in the images, indicating high electron density, and they correspond to the deposition of Pt nanoparticles. They are relatively and homogeneously dispersed in the case of SSSG and IWI methods rather than the PCD method, of which aggregation of Pt particles seems to more easily take place on the TiO2 surface. Average Pt particle size of the SSSG and IWI photocatalysts is approximately 1 nm, while it becomes larger and more diverse up to 5 nm due to some aggregations in the case of PCD method.

TEM images of (a) 0.6 wt% Pt/TiO2 prepared by SSSG method, (b) 0.4 wt% Pt/TiO2 prepared by IWI method, and (c) 0.5 wt% Pt/TiO2 prepared by PCD method.
3.2 Photocatalytic activity
Photocatalytic activity of the Pt/TiO2 prepared by the three methods was quantitatively examined by photocatalytic H2 evolution from the suspension of the photocatalysts with various Pt loadings (0.1–0.9 wt%) in an aqueous methanol solution for 5 h irradiation using a 300-W high-pressure Hg lamp (The lamp emits mostly UV light.). Concisely about the mechanism of photocatalytic H2 evolution over Pt/TiO2, the reaction is initiated by the photoexcitation of TiO2 particles, which leads to the formation of electron–hole pairs. Photogenerated conduction band electrons can be transferred to electron acceptor, H+. With Pt on TiO2 photocatalyst, Pt can trap electrons and hydrogen can be produced on Pt by reduction reaction. Likewise, valence band holes can be mainly filled by the electron donor, CH3OH, as well as inevitable surface hydroxyl groups on TiO2, resulting in the great prevention of photoinduced charge recombination [16].
Control experiments showed that no appreciable hydrogen was formed, by stirring the aqueous suspensions in the dark. In addition, water alone did not show any evidence of H2 evolution upon the irradiation. However, the irradiation of TiO2 or Pt/TiO2 suspensions containing methanol led to the observable evolution of H2 gas. Fig. 4a–c illustrate the time course of photocatalytic H2 evolution from the photocatalysts prepared by SSSG, IWI, and PCD methods, respectively. Comparatively small H2 evolution was observed when unloaded TiO2 was utilized. The deposition of Pt on TiO2 resulted in a substantial improvement in the H2 evolution. The amount of H2 produced increased almost proportionally to the irradiation time in the investigated period, especially in the initial period. However, a slight decrease in the H2 evolution rate at long irradiation period was detected. This has been previously explained that in photocatalytic batch systems, long irradiation periods regularly cause a progressive decline in the H2 generation rate due to back reactions of products generated in the reaction system as well as pressure buildup in the gas phase [17].
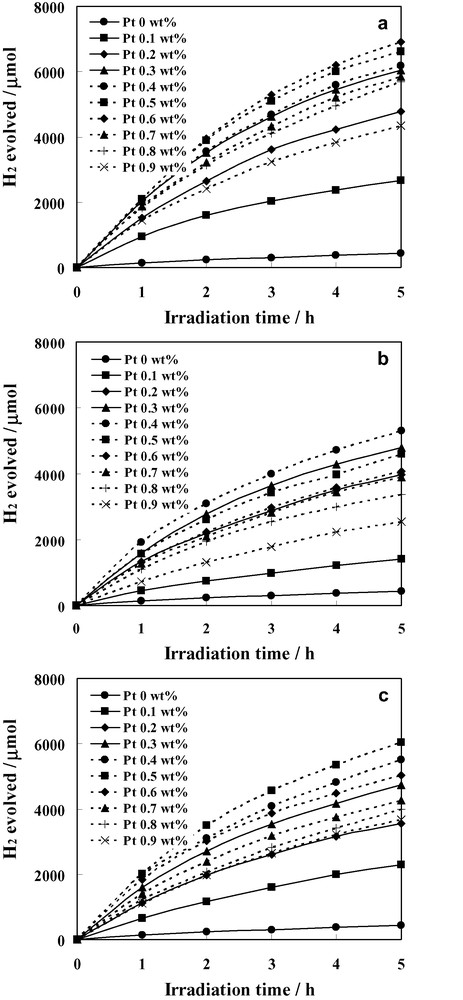
Time course of photocatalytic H2 evolution over Pt/TiO2 photocatalysts prepared by (a) SSSG, (b) IWI, and (c) PCD methods (Photocatalyst, 0.2 g; distilled water, 200 ml; methanol, 20 ml).
As seen in Fig. 4, the H2 evolution from the Pt/TiO2 prepared by the three methods are in the same order of magnitude, nonetheless the differences in the evolution rate can be clearly observed. For better clarity, the H2 evolution rate is represented as a function of Pt loading as shown in Fig. 5. It is manifest that optimum amount of Pt loading for each preparation method exists. Irrespective of preparation methods, with the initial increase in the Pt loading amount, TiO2 particles may carry more Pt nanoclusters, which are indispensable for the removal of photogenerated electrons from TiO2 for the reduction reaction and lead to an increase of the photocatalytic activity. After reaching a maximum, the decrease in the activity with further increase in the Pt content beyond a certain optimum value may be, at least partly, due to too much Pt nanoclusters on TiO2. These clusters would shield the photosensitive TiO2 surface, scatter the UV light to decrease the absorption by TiO2, and subsequently diminish the surface concentration of the electrons and holes available for the reactions. Another explanation is that at high metal loadings, the deposited metal particles may act as the recombination centers for the photoinduced species [18].
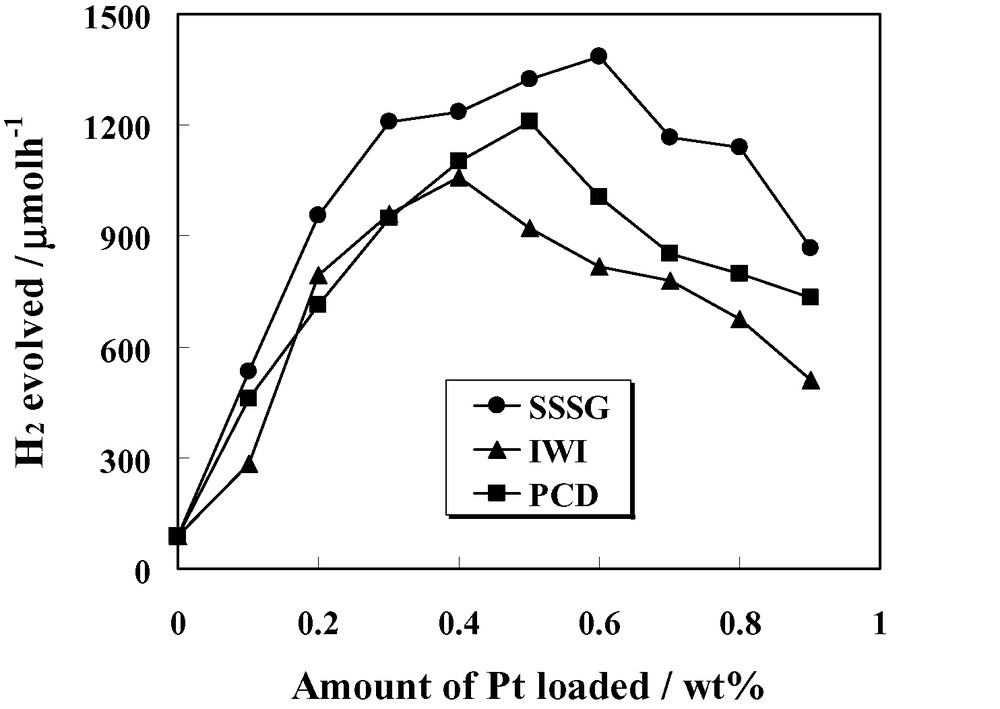
Dependence of photocatalytic activity of H2 evolution on photocatalysts prepared by three methods on Pt loading content.
Even though the existence of optimum Pt loading amounts was observed, such the amounts somewhat varied depending upon the preparation methods. The optimum Pt loading amounts for SSSG, IWI, and PCD methods are 0.6, 0.4, and 0.5 wt%, exhibiting the H2 evolution rate of 1385, 1060, and 1210 μmol h−1, respectively. As mentioned earlier that the loaded Pt particles in the SSSG method may deposit on the surface of TiO2 through the mesoporous network instead of the outer and near the outer surface of TiO2 aggregates, the light shielding effect becomes less pronounced than the IWI and PCD methods, leading to the highest observed optimum amount of Pt loading. Additionally, some aggregations of Pt particles in the PCD method may be plausibly ascribed to a slightly higher value of the optimum Pt content than the IWI method.
A particularly successful point to be underscored in this work is that over the investigated range of Pt loading amount, the SSSG Pt/TiO2 provided the highest photocatalytic activity, followed by the PCD and IWI Pt/TiO2, respectively. The interaction between excited species (TiO2) and trapping species (Pt) can be considered as a key mechanism in this reaction system because it signifies the capability of transferring the photoexcited electrons from conduction band of TiO2 to the Pt active sites and initiating the photocatalytic reaction. Therefore, the enhancement of the photocatalytic activity for H2 evolution over the largest surface area SSSG photocatalyst may be due to at least two possibilities: (1) the increased accessibility of reactant, water molecules, to the photocatalyst surface active sites existing along the mesopore network and (2) the high dispersion of Pt particles deposited through the mesopore structure of TiO2 support, leading to the better electronic interaction between the cocatalyst and support in the present system. Previous works also demonstrated the good interaction between cocatalyst and support in the sol–gel process [19,20]. Despite expected less such the interaction than the SSSG method, it convincingly becomes more considerable in the case of the PCD method than the IWI method, as pointed out by Karakitsou and Verykios [17]. Besides, for the PCD method some aggregations of Pt particles, which may be useless, may affect the lower photocatalytic activity than the SSSG method.
Another noticeable observation is the photocatalytic activity window of the Pt/TiO2 prepared from different methods. From Fig. 5, it is clearly shown that the SSSG Pt/TiO2 provided the broadest photocatalytic activity window towards the Pt loading amount. This also verifies the superior performance in photocatalytic H2 evolution of the Pt/TiO2 prepared by the SSSG method to the other two conventional methods.
Overall, the utilization of mesoporous TiO2 as the support for platinum was proved to be an effective means contributing to the considerably high photocatalytic activity of H2 evolution. Owing to the fact that the differences in the photocatalytic activity were observed for each preparation method, the Pt loading by single-step sol–gel method helps improve the physical characteristics directed to the enhancement of the photocatalytic functioning. This would suggest us a great opportunity to design and select new highly active photocatalysts.
4 Conclusions
The mesoporous TiO2 with uniform and narrow pore size distribution prepared by a surfactant-assisted templating sol–gel process was utilized to support 0.1–0.9 wt% Pt by three loading methods, namely single-step sol–gel (SSSG), incipient wetness impregnation (IWI), and photochemical deposition (PCD) methods. The resulting Pt/TiO2 photocatalysts were systematically evaluated their performance via the photocatalytic H2 evolution. The SSSG method provided the superior photocatalytic activity over the entire range of Pt loading amounts to the other two conventional methods. The broadest photocatalytic activity window towards the content of Pt loading was also observed with the mesoporous Pt/TiO2 prepared by SSSG method. It should be, therefore, remarked that the coupled single-step sol–gel process with mesopore-controlling surfactant has unveiled as a useful technique for the preparation of metal-loaded mesoporous photocatalyst with high photocatalytic activity.
Acknowledgements
This work was financially supported by the Grant-in-aid for Scientific Research under the 21COE Program and the Nanotechnology Support Project. Gratefulness is forwarded to (1) Professor S. Isoda and Professor H. Kurata and (2) Professor T. Yoko at Institute for Chemical Research, Kyoto University for their supports of TEM and XRD apparatus, respectively.