1 Introduction
Heteropolyacids are known to be very acidic and so to display interesting properties in acid catalysis [1–3]. However, if their use in homogeneous catalysis does not present any problem if polar solvents are used, it is more difficult to use them in heterogeneous catalysis for reactions of apolar molecules such as alkanes. Indeed, their surface area is very low (only a few m2 g− 1) and so only a small part of the protons are really useful for the catalytic reaction. As a consequence, a lot of studies were published on the immobilization of heteropolyacids on various supports and their use in catalysis. Supports such as ZrO2 [4,5], carbon [6], silica–alumna [7], clays [8] were used, this list being not limitative, but most studies were devoted to silica supported heteropolyacids [9–12], this support being known as relatively inert. Such catalysts display high acidity, allowing them to be used in reactions such as isomerization or cracking of alkanes [9,13–20].
If it is now generally admitted that the first stage of the “grafting” reaction of the heteropolyacid on the support is the protonation of surface hydroxyl groups [11], only few studies were published on the characterization of these catalysts after a thermal treatment or in catalytic reaction conditions (see for example Refs. [12,21,22]). We have then decided to undertake a detailed study of these systems and we present in this first paper the results of a 1H MAS NMR study of H3PW12O40/SiO2 and H4SiW12O40/SiO2 catalysts as a function of the dehydroxylation temperature. These heteropolyacids were chosen as they display the highest acidity [3] and so are potentially the best candidates for the obtention of highly efficient supported catalysts.
2 Experimental
H3PW12O40 (HPW) and H4SiW12O40 (HSiW) were purchased by Acros and used without further purification. The silica used for these studies was Aerosil silica from Degussa. It is a non-porous solid with a B.E.T. surface area of 200 m2 g−1. The preparation of supported polyanions was made by classical impregnation with an aqueous solution of the heteropolyacid and drying at 80 °C [9,12]. In a first step, three solids were prepared, for each polyanion, containing, respectively, 20, 40 and 60 wt.% of heteropolyacid. These solids were first characterized by microanalysis, infrared spectroscopy (KBr pellets) and X-ray powder diffraction spectroscopy. All results were in agreement with previously reported data [9,12,23].
These catalysts, together with pure polyacids and silica, were studied by solid-state 1H MAS NMR as a function of the temperature. For these studies the catalyst powder (200 mg) was treated under vacuum at the desired temperature during 2 h and kept in a sealed ampoule. In order to achieve relatively quantitative results, all 1H MAS NMR spectra were recorded in the same time period. The ampoule containing the sample was broken in a glovebox and the rotor filled and tightly closed by using a new cap for each experiment (studies with metal hydrides have proved that in this case the rotor was water- and air-tight). The amount of product in the rotor was determined by weighting the rotor before and after filling. The MAS NMR spectrum was then recorded on a Bruker DSX-300 spectrometer operating at 300.13 MHz. The spinning rate was typically 10 kHz. Eight scans were acquired after a π/2 pulse. It was checked with a great attention that the nuclei had completely relaxed between two consecutive scans. This needed recycle delays which could vary up to 1000 s.
3 Results and discussion
3.1 Pure heteropolyacids and silica
Fig. 1 shows the 1H MAS NMR spectra (recorded with a large spectral window, typically 300 000 Hz, corresponding to 1000 ppm) of pure HPW and HSiW heteropolyacids after treatment under vacuum at room temperature. It can be seen that the two heteropolyacids have a quite different behavior, the spectrum of HPW showing numerous sidebands while that of HSiW has only a relatively sharp peak and spinning sidebands of very low intensity. It is well known that H3PW12O40 can be found as various hydrates. The most known is the hexahydrate H3PW12O40·6 H2O where each proton is linked to two water molecules, forming H5O2+ clusters [24]. This hydrate is formed relatively easily, for example by treating the heteropolyacid under vacuum at room temperature and it is relatively stable at room temperature. Powder X-ray diffraction shows that it is the case for the compound of Fig. 1.

1H MAS NMR spectra with a large spectral window of pure H3PW12O40 and H4SiW12O40 heteropolyacids after treatment under vacuum at room temperature during 2 h.
This spectrum corresponds then to the hexahydrated heteropolyacid and more exactly to the H5O2+ ion, the spinning sidebands corresponding to the dipolar coupling between the various protons. In contrast, the absence of spinning sidebands in the spectrum of HSiW corresponds to the absence of dipolar couplings between protons and so to isolated protons. This feature is in agreement with a complete dehydration of the heteropolyacid and the formation of anhydrous H4SiW12O40.
From a quantitative point of view, the number of protons per Keggin unit (as it can be evaluated by comparison with a reference sample of silica dehydroxylated at 500 °C for which the number of protons is known) is in agreement with these interpretations.
Fig. 2 shows the 1H MAS NMR spectra of the two heteropolyacids and of silica as a function of the dehydroxylation temperature. In the case of silica, the spectra can be decomposed into two components corresponding to isolated silanol groups (sharp peak at 1.8 ppm) and a broad resonance between 2 and 10 ppm corresponding to hydrogen-bonded silanol groups. These data were discussed in detail in a previous paper [25] and allowed a quantitative determination of the number of silanol groups per nm2.
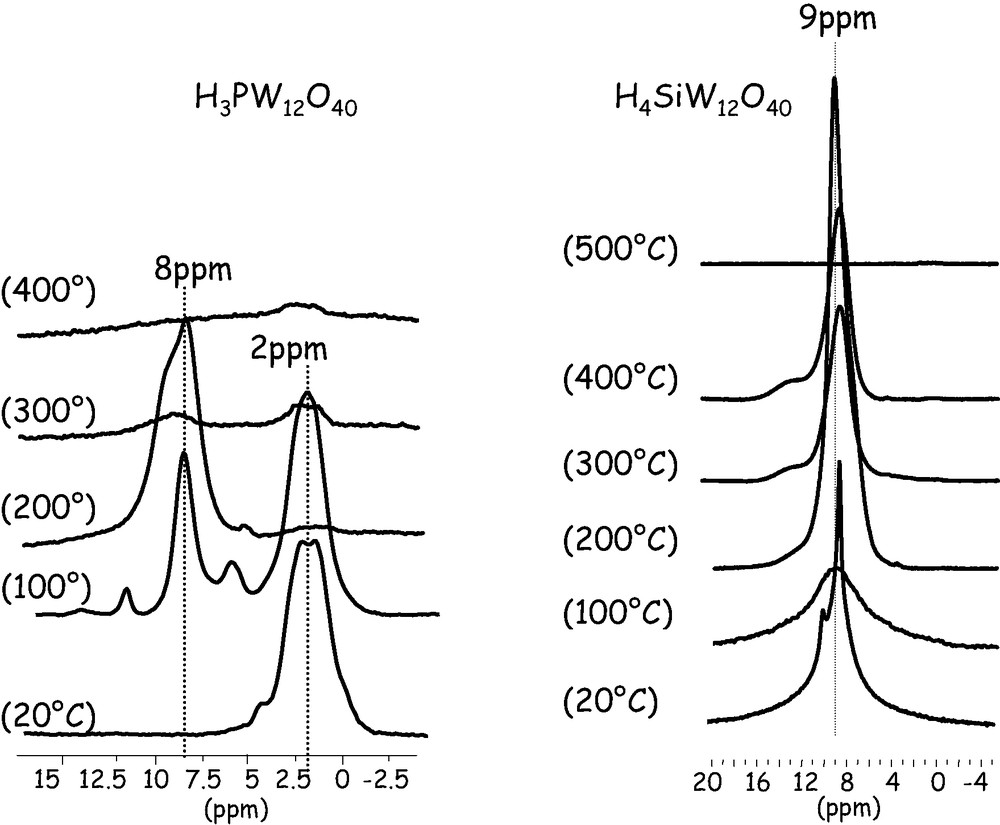
1H MAS NMR spectra of pure H3PW12O40, H4SiW12O40 and silica after dehydroxylation at various temperatures: 20 °C; 100 °C; 200 °C; 300 °C; 400 °C; 500 °C.
As said above, the NMR spectrum of HPW dehydroxylated at 25 °C corresponds to the hexahydrate H3PW12O40·6 H2O. When treating at higher temperatures, water molecules are removed and anhydrous HPW is formed. After treatment at 200 °C, only one signal is observed at ca. 8 ppm, which can be attributed to protons in anhydrous HPW, in agreement with literature data [26,27]. The spectrum after treatment at 100 °C shows the presence of the anhydrous and hexahydrate forms of the heteropolyacid. The determination of the number of protons per Keggin unit by integration of the spectra is in agreement with these conclusions. What is more interesting is the evolution at higher temperatures. Indeed, in contrast to what is usually written, after treatment under vacuum at 300 °C most of the protons have disappeared, indicating that the heteropolyacid is not stable, under our conditions, at this temperature. These results cannot say if the polyanion has retained its structure as we proposed it some years ago [28] in order to explain the increase of the surface area but the fact that the heteropolyacid can be easily recovered by addition of water should be in agreement with this hypothesis.
The behavior of the silicotungstic acid is quite different: First of all, even after treatment at room temperature the anhydrous polyacid is obtained, as evidenced by the signal at ca. 9 ppm. Second, the signal of the protons remains until 400 °C and disappears only at 500 °C. This shows that this polyanion, in its protonated form, is stable at a higher temperature than the phosphotungstic acid. The discrepancy between our results and the commonly accepted stability order is probably related to the formation of the anhydride phase, where the phosphotungstic entity retains its integrity.
3.2 Supported heteropolyacids
Figs. 3 and 4 show the 1H MAS NMR spectra of the supported phosphotungstic and silicotungstic acids, respectively, as a function of the temperature. As for the pure heteropolyacids, there is a completely different behavior for phosphorus and silicon.

1H MAS NMR spectra of supported HPW/SiO2 catalysts (20, 40 and 60 wt.% HPW) after dehydroxylation at various temperatures: 25 °C; 100 °C; 200 °C; 300 °C; 400 °C; 500 °C.
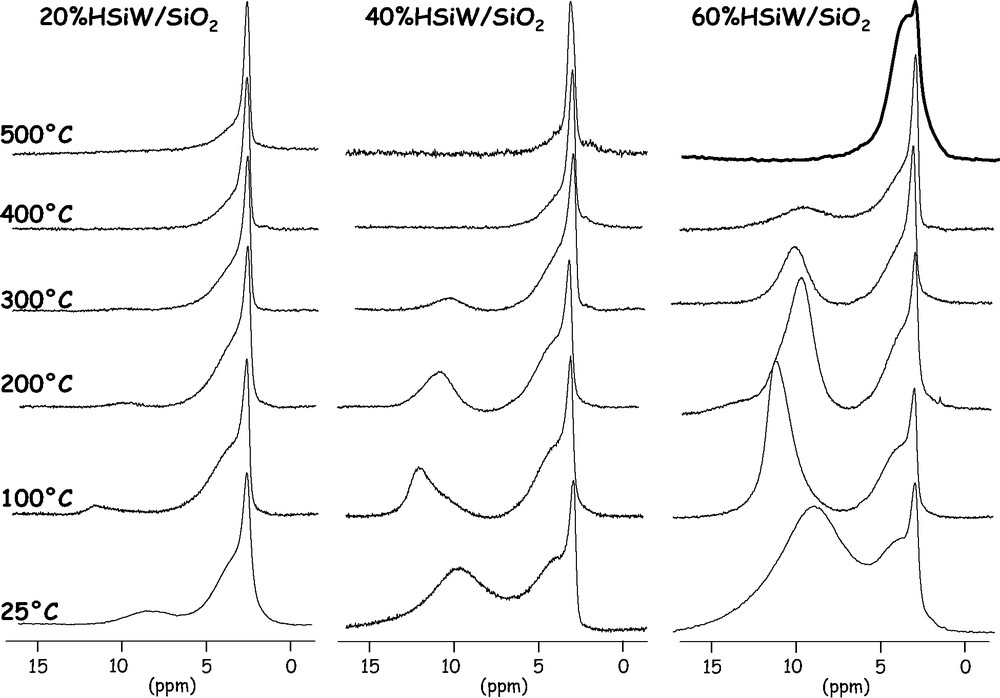
1H MAS NMR spectra of supported HSiW/SiO2 catalysts (20, 40 and 60 wt.% HPW) after dehydroxylation at various temperatures: 25 °C; 100 °C; 200 °C; 300 °C; 400 °C; 500 °C.
All spectra of supported HPW display a quite similar feature with essentially two components, a sharp one at 1.8 ppm which can be attributed to isolated silanol groups as above and a broad one from 2 to 5 ppm which could be attributed to hydrogen-bonded hydroxyl groups. The peak at ca. 8 ppm which is attributed to protons in anhydrous HPW is not observed even for the highest HPW loading (60 wt.%) and whatever the dehydroxylation temperature. This observation is very important as the high acidity of HPW is related to this proton. We can then conclude from these data that the supported heteropolyacid is less acidic than the pure one. These results are in agreement with other methods such as microcalorimetry [12,23].
For supported HSiW/SiO2 catalysts, the behavior is very different, the spectra showing clearly the signal at 8–9 ppm which can be attributed to strong acid sites. So we can conclude from these data that HSiW/SiO2 will be more acidic than HPW/SiO2 and that they will display acid catalytic activities quite similar (in terms of strength) to those of the pure heteropolyacids. It can also be pointed out that the surface area of the signal near 8 ppm increases with the amount of heteropolyacid on the support. These results are in agreement with our previous results on n-hexane cracking [9] where we found that the catalytic activity increased as a function of the HSiW loading while it showed a maximum as a function of the HPW loading. Another interesting feature of this signal is that its position seems to vary upon the dehydroxylation temperature, the signal after treatment at 100 °C being shifted. It is difficult to interpret this variation with only these data and additional experiments such as spin countings and 1H multiquanta experiments are needed to interpret them.
The highest polyacid loading (60 wt.%) corresponds to ca. 1.5 polyanion per nm2 on the silica surface, a value which corresponds to a few more than a monolayer. Even in these conditions, whatever the heteropolyacid the 1H MAS NMR spectrum shows the presence of isolated silanol groups, in a non-negligible amount. If the distribution of the heteropolyacid was completely homogeneous on the surface, no free silanol groups should be observed: hydroxyl groups should be in interaction with the heteropolyanion or with the protons, leading to a signal corresponding to hydrogen-bonded protons. As it is not the case, we must suppose that the distribution of the polyanions is not fully regular. X-ray powder diffraction showing no peaks attributable to crystalline heteropolyacid, more probably there is agglomeration of some polyanions on the surface.
4 Conclusion
We have shown that pure and supported phosphotungstic and silicotungstic acids have a quite different behavior as a function of the dehydroxylation temperature: Pure HPW gives the hexahydrate at room temperature and at higher temperatures the anhydrous polyacid while HSiW gives directly the anhydrous species, even at room temperature. In addition HPW losses its protons at a lower temperature than HSiW. For supported heteropolyacids, strongly acid protons are observed only in the case of HSiW/SiO2 catalysts, in agreement with previously reported catalytic data. The presence of a non-negligible amount of remaining silanol groups even for polyanion loadings higher than the monolayer suggests that the polyanions are not located homogeneously on the surface. Further studies including a more quantitative interpretation of these data will be published elsewhere.