1 Introduction
The understanding of the mechanisms of hydrocarbon conversion on heterogeneous catalysts is a prerequisite for a progress in this field. Since 1989 [1], in situ magic angle spinning (MAS) NMR spectroscopy has been established as a useful tool for the study of active catalytic sites, surface complexes formed by the reactants, intermediates and deactivation of solid catalysts. Most of in situ MAS NMR techniques applied during the last decade use samples placed in gas-tight MAS NMR rotors or fused in glass ampoules which are inserted in a conventional MAS rotor [2–8]. The reaction conditions in these cases are comparable with those in batch reactors. However, heterogeneous catalyzed reactions in the chemical industry are often performed under flow conditions. The spectroscopic investigations of equilibrium state in heterogeneously catalyzed reactions then require the use of systems where it is possible to apply a continuous flow of gas reactants through the solid catalyst. This kind of in situ spectroscopy is now often called operando spectroscopy [9]. In 1987, Haddix et al. [10] made the first attempt to develop an in situ NMR probehead that had capabilities of standard micro-reactors, but at that time, the NMR spectra were measured without sample rotation. In 1995, Hunger et al. [11,12] developed a NMR probehead allowing in situ investigations with MAS under a continuous flow of reactant molecules. This MAS NMR probehead allowed the investigation of intermediates occurring on the catalyst surface in the steady state of the reaction. The equipment consisted of a MAS NMR rotor with an axially placed injection tube inserted into the rotor via a hole in the rotor cap. The sample could be heated up to 423 K at ambient flow pressure and the MAS rates was up to 3 kHz. In a subsequent study [13], the authors devised a method for analyzing products flowing out of the MAS reactor, by coupling the system with on-line gas chromatography. Later, Goguen and Haw [14] described a similar in situ MAS NMR system that could be heated up to 523 K. Namara and Raftery [15] described an alternative method for building a probehead with gas flow for in situ NMR, relying on magic angle hopping (MAH) to average chemical shift anisotropy and dipolar interactions rather than MAS. The advantage of this design is that variable pressure up to 13.8 MPa can be used. But unfortunately, the MAH experiment suffers in sensitivity with respect to a conventional MAS experiment, and the time required for such a measurement is likely to be very long [16]. Isbester et al. [17] developed another type of flow MAS NMR probehead that used ceramic ball bearings rather than conventional air bearings to support the rotor. This leads to a very good insulation of the reactant flow from the air drive flow, but mechanical frictions during the rotor spinning can be a problem for long run experiments. Recently that continuous flow probeheads have also been successfully combined with optical pumping process to introduce laser polarized xenon into a powder for the characterization of microporous solids by 129Xe NMR spectroscopy [18–25]. In this work, we described an attempt to realize an operando MAS NMR probehead coupled with mass spectrometry. This probe is designed from a conventional probehead including an adapted MAS system. It allows the continuous flow of gas reactants through the solid catalyst during the NMR experiment at temperature up to ca. 540 K and a direct analysis of the reaction products leaving the MAS NMR rotor reactor using on-line mass spectrometry. The ability of this probe for catalysis has been checked by following the hydrogenation of toluene on Pt/sulfated zirconia.
2 The operando MAS NMR probehead
A MAS NMR probehead has been build based on the original idea and design of Hunger et al. [11–13]. The system is designed in such a way that the rotor acts as a catalytic micro-reactor allowing the measurement of NMR spectra during the reaction. Fig. 1 shows the MAS NMR probehead that has been constructed starting from a conventional Bruker 7-mm MAS NMR double-bearing system (WVT).

Continuous flow MAS NMR probehead design. The construction is based on a standard 7-mm WVT MAS turbine from Bruker. The numbers in circles serve for reference in the text.
In this system, the catalyst powder is packed into the rotor using a special tool in order to make a catalyst bed that has the shape of a hollow cylinder. As the usual insertion/ejection device has been removed, the rotor is manually inserted into the MAS turbine (Fig. 1.). This insertion/ejection device is replaced here by a support (Fig. 1.), the flow insert holder, which maintains the gas injection system (Fig. 1.). This gas injection system is made of cylindrical pieces of ceramic (in MACOR for this prototype) that holds the input and output gas tube line and the flow tube (Fig. 1.). The flow tube, made of glass in this prototype, is accurately aligned along the axis of the MAS rotor through an axial hole in the rotor cap (Fig. 1.). The aim of this flow tube is to inject the reactant molecules inside the rotor, at the bottom of the catalyst bed. During MAS experiments, the catalyst bed then rotates freely around this flow tube. Perfluro alkoxyalkane (PFA) plastic or titania metallic tubes are used to carry on the flow in and out of the probehead.
This MAS NMR micro-reactor can be heated to a maximum temperature of ca. 540 K under atmospheric pressure when titania metallic tubes are used but only to 473 K when the PFA tubes are used. The handling of the insert system (Fig. 1.) is however less easy when titania tubes are used due to the rigidity of the metal. For the reaction performed in this work, which does not need high temperature, the plastic PFA tubes were thus preferred for sake of simplicity. The maximum spinning speed of the MAS rotor that has been checked on this system was 3 kHz.
Gases exiting the spinning rotor are collected with a pump and analyzed on-line with a mass spectrometer. The overall scheme of the in situ MAS NMR flow system coupled with the mass spectrometer is shown in Fig. 2.

Scheme of the in MAS NMR flow system coupled with on-line mass spectrometry.
3 Experimental
In this work, the hydrogenation of toluene was used to validate the operando MAS NMR system. The catalyst, 0.3% Pt-loaded sulfated zirconia (noted Pt/ZrO2–SO4), can be activated at relatively low temperature. The synthesis and characterization of the catalyst have been described elsewhere [26]. A saturator is used to load the carrier gas with toluene (Carlo Erba, 99.5% purity). A mass flow controller (Brooks) allows adjusting the flow rate (ml min−1) of the carrier gas. The saturator is cooled to 283 K, whereas the tube connecting the saturator to the NMR probehead is heated approximately at 323 K. The lines connecting the output of the probehead with the mass spectrometer are also heated at the same temperature to minimize condensation. In these conditions, the vapor pressure of the toluene is around 1600 Pa. The weight of the catalyst in the rotor is approximately 400 mg. The contact time (W/F) is 13 700 g min−1 mol−1 and the hydrogen to toluene ratio (FH2/FTol) in the feed flow is 64. The total flow is 50 ml min−1.
1H MAS NMR investigations were performed at 400 MHz on a Bruker Avance 400 spectrometer, with a sample spinning rate of 2.1 kHz. For each 1H spectrum 16 free induction decays were accumulated with a repetition time of 10 s. The 1H NMR chemical shifts are referenced to tetramethylsilane (TMS). The reaction products were analyzed on-line by mass spectrometry (Pfeiffer Omnistar) and out-of-line by gas chromatography (Varian 3900). The temperature inside the MAS rotor was calibrated using the 207Pb MAS NMR shift of Pb(NO3)2 [27]. The spectra were measured by accumulating 64 scans with a 90° pulse of 3.8 μs and a relaxation delay of 10 s. The 207Pb chemical shifts are referenced to tetramethyllead (TML).
Benzoic acid (Fluka purum) and adipic acid (Aldrich, 99% purity) were used to calibrate the sensor temperature.
To compare the conversion results with a conventional catalytic measurement, a fixed bed reactor was packed with 400 mg of catalyst. For the catalytic measurements, a flow of toluene, corresponding to a contact time of 13 700 g min–1 mol, was introduced and products were analyzed by gas chromatography.
4 Results and discussion
4.1 Calibration of rotor temperature
Since in the probehead design, the temperature sensor is not in close contact with the sample, there is a systematic error in the temperature reading. In addition, significant temperature gradients within the rotor are expected [28]. A calibration of the thermocouple and determination of the correction factors to compensate the reading errors was therefore performed by using the melting point of benzoic acid (395 K) and adipic acid (425 K). First, the 1H NMR spectra were recorded while heating the sample slowly without spinning the rotor. The change in proton-line width and the sudden increase in intensity of the signal indicate the melting point of the compounds. From this data, it was found that the actual temperature of the rotor is related to the temperature reading on the sensor by the following equation:
(1) |
The variable temperature of the probehead were then tested using the 207Pb resonance chemical shift in Pb(NO3)2. This allows measuring the temperature inside the rotor with or without rotation and the temperature gradient over the whole sample volume. The 207Pb MAS NMR spectra of Pb(NO3)2 were first recorded for different target temperatures without spinning the sample. As expected, a linear relation was found between the chemical shift and the temperature of the rotor:
(2) |
The slope of 0.820 ppm K–1 is however slightly higher than those reported in literature: 0.775 ppm K–1 in Ref. [29] and 0.753 ppm K–1 in Ref. [30].
Since the MAS can result in a small heating even at low speeds, it is also necessary to calibrate the temperature of the rotor and temperature gradients for the spinning rate of 2.1 kHz that has been chosen to check the catalytic performance of the probehead. Fig. 3 shows the 207Pb MAS NMR spectrum of Pb(NO3)2 when it is heated in the flow MAS system using variable temperature gas from 298 to 473 K. As the temperature is increased, the line becomes progressively broader, indicating thermal gradients across the rotor. At 473 K, the line is ca. 8 ppm broad, corresponding to a temperature gradient of ca. 7 K. The rotor temperature determined from the chemical shift value of the Pb(NO3)2 is found equal to 0.715 times the temperature of the sensor.
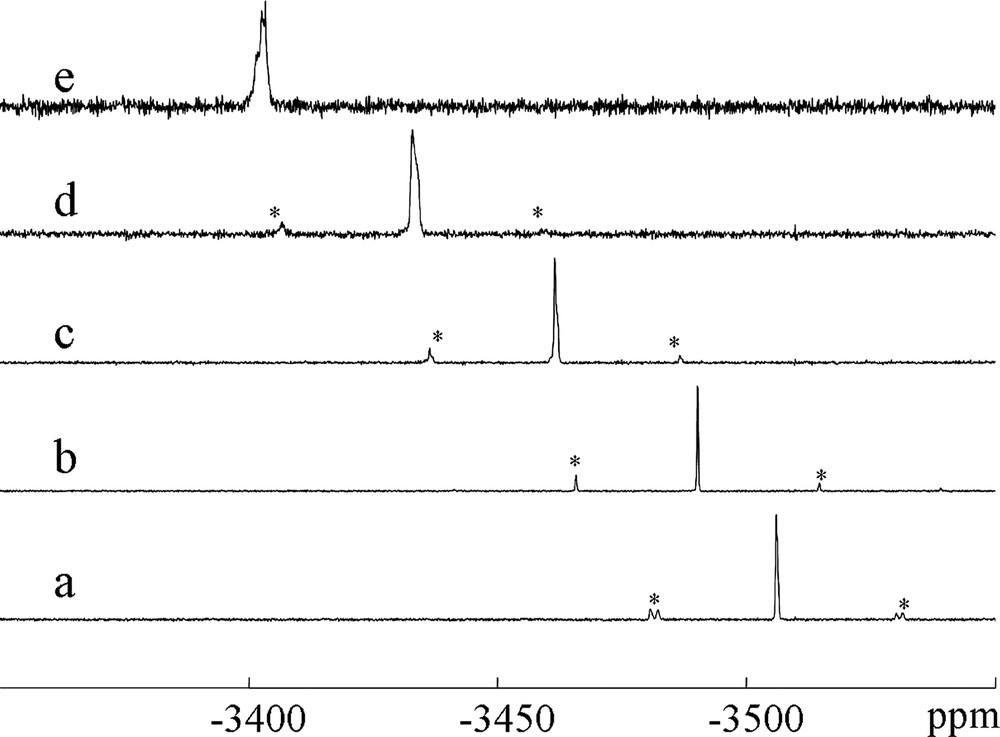
The 207Pb MAS NMR spectra of Pb(NO3)2 for various target temperatures as measured with the sensor of the VT controller: 298 K (a), 323 K (b), 373 K (c), 423 K (d) and 473 K (e). Asterisks denote spinning sidebands.
To determine whether or not the gas flow may affect the temperature of the rotor, the same measurements were repeated by spinning the sample first without any flow and secondly in the presence of a N2 flow of 50 ml min–1. Clearly, the introduction of a N2 flow into the rotor results only in a very small change of the rotor temperature. These data are reported in Table 1.
Temperature measured in the rotor with and without flow of N2 gas. The spinning rate was 2.1 kHZ. The N2 flow was 50 ml min–1
Sensor temperature (K) | Rotor temperature (K) | |
Without flow | With nitrogen flow | |
295 | 299 | 299 |
383 | 361 | 360 |
393 | 368 | 368 |
403 | 375 | 375 |
443 | 405 | 402 |
453 | 411 | 410 |
463 | 419 | 417 |
473 | 426 | 424 |
4.2 In situ 1H MAS NMR investigation on toluene hydrogenation
Toluene hydrogenation is a well-established reaction. This reaction was thus chosen as a simple reference reaction in order to check the ability of the continuous flow MAS NMR probe to carry out catalysis studies. The hydrogenation of toluene was performed on a 0.3% Pt-loaded sulfated zirconia (Pt/ZrO2–SO4) catalyst. To carry out the necessary initial activation of the catalyst, the powder was packed inside the rotor, inserted in the MAS turbine and subsequently heated to 423 K under a nitrogen flow of 43 ml min–1. The catalyst was then activated at this temperature for 2 h under a hydrogen flow of 40 ml min–1.
The chemical shift of the toluene and methylcyclohexane were first measured by following the adsorption of both molecules on the Pt/ZrO2–SO4 catalyst in flow condition and at different temperatures, using N2 as a carrier gas (43 ml min–1). The corresponding 1H MAS NMR spectra obtained at temperatures of 333, 363 and 423 K are shown in Figs. 4 and 5 for toluene and methylcyclohexane, respectively.

1H NMR spectra of toluene continuously adsorbed on Pt/sulfated zirconia at 333 K (a), 363 K (b) and 423 K (c) under a flow of 43 ml min–1 of nitrogen. Asterisks denote spinning sidebands.

1H NMR spectra of methylcyclohexane continuously adsorbed on the Pt/sulfated zirconia at 333 K (a), 363 K (b) and 423 K (c) under a flow of 43 ml min–1 of nitrogen. Asterisks denote spinning sidebands.
The spectra of adsorbed toluene show signals at 6.99–7.63 and at 2.12–2.61 ppm. The former signal is due to aromatic protons and the latter is caused by methyl protons. The spectra of methylcyclohexane show signals at 1.76–1.80 ppm (equatorial-H), at 1.35–1.42 ppm (axial-H) and at 0.95–1.01 ppm (methyl-H). In both Figs. 4 and 5, shoulder is observed on the upfield side of all peaks. The main line and the shoulders could be related to two kinds of toluene and methylcyclohexane molecules present in the rotor, one being in the gas phase and the other in exchange with the absorption sites in the catalyst. As the sample temperature was increased, the chemical shifts of both toluene and methylcyclohexane shifted downfield. Such change in chemical shift with increasing temperature has also been observed in the studies of dimethylether reacting with zeolite HZSM-5 [31] and in methanol adsorption on HZSM-5 [17] with continuous flow MAS NMR flow probe.
In a second step, the toluene hydrogenation on Pt/sulfated zirconia under flow conditions was performed using H2 as reactant and carrier gas. 1H MAS NMR spectra obtained at various temperatures are shown in Fig. 6. As the temperature is increased, the resonances of methylcyclohexane grow in intensity, showing an enhancement of the conversion. The 1H chemical shifts of toluene and methylcyclohexane obtained during toluene hydrogenation are very similar to those of the adsorbed toluene and methylcyclohexane at the same temperatures. At 423 K, the spectra are clearly dominated by the signals at 1.1–1.8 ppm due to the adsorbed methylcyclohexane. These spectra are fully reversible. Indeed, the intensity of the methylcyclohexane signals decreases and the intensity of those corresponding to toluene signals increase when the temperature is lowered because of smaller toluene conversion.
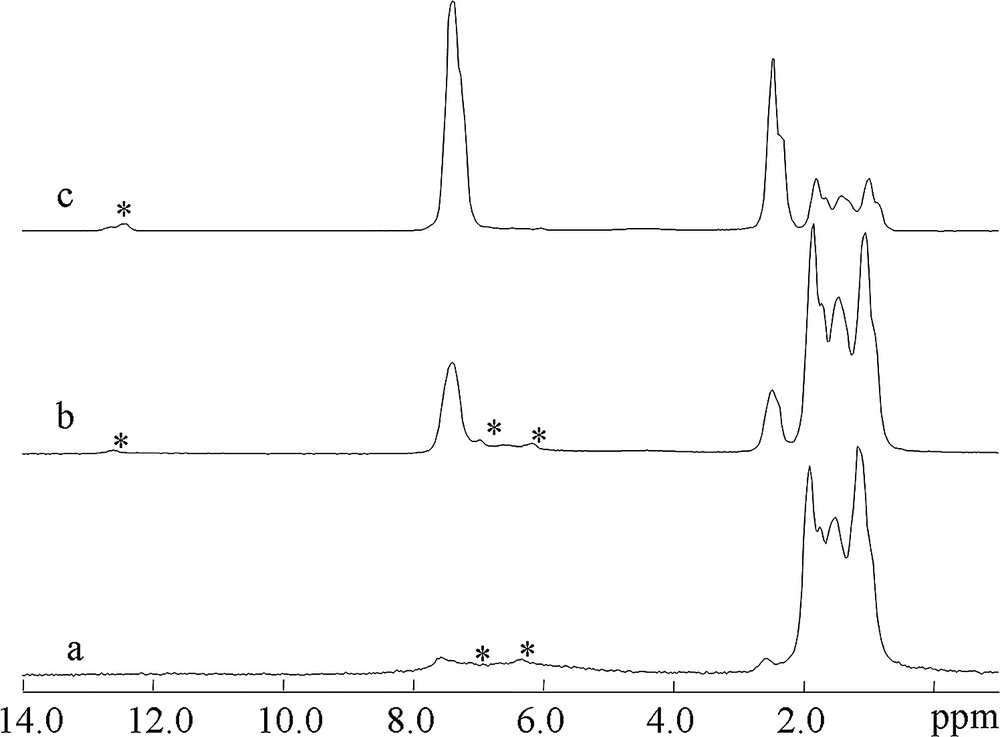
1H MAS NMR spectra recorded during toluene hydrogenation at 423 K (a), 363 K (b) and 333 K (c). Asterisks denote spinning sidebands.
The gases coming out of the MAS NMR rotor were simultaneously analyzed using the mass spectrometer. When toluene was injected into the MAS rotor at 423 K, the mass spectrum shows that a large amount of methylcyclohexane is selectively formed. The amount of methylcyclohexane decreases as expected, with a simultaneous increase of the toluene concentration, when the reaction temperature is reduced to 333 K, due to the decrease of toluene conversion. Oxygen and nitrogen are always detected in large amount in the mass spectrum, showing that the product/reactant gas coming from the MAS micro-reactor mixes with the air flow used as drive and bearing in the MAS system. This observation shows that in case of air sensitive reaction studies, it will be mandatory to use neutral gas to spin the MAS rotor.
4.3 Comparison with the catalytic hydrogenation of toluene carried on a fixed bed reactor
To clarify the significance of in situ MAS NMR investigations under continuous flow conditions for the study of hydrogenation of toluene on Pt/sulfated zirconia, the catalytic investigations were performed under similar conditions in a conventional fixed bed reactor and in the continuous flow MAS NMR reactor. The analysis of the products was done by gas chromatography.
To compare quantitatively the conversion obtained with the fixed bed reactor and those obtained with the MAS reactor, the exit gases from MAS NMR rotor were sampled using a gas syringe and products analysis was also done by gas chromatography.
At 423 K, 100% conversion was obtained with the fixed bed catalytic reactor, whereas 94.4% conversion was obtained with the MAS reactor. However, the high conversion level reached at this temperature makes comparison impossible. At 333 K, values of conversion for fixed bed reactor and MAS reactor are more different (respectively, 12% and 32%). This difference can be explained by condensation of the products (toluene or methylcyclohexane) in the line. Actually, to avoid product condensation, the lines outside the probe are heated at 323 K. But, up to now, no-heating of in and out-lines inside the probehead body is done, which could be the reason for some product condensation. Hunger et al. [32] also observed such differences in conversion of methanol on SAPO-34 and SAPO-18 with their probe when it was compared with fixed bed reactor.
5 Conclusions
A conventional MAS NMR probehead was modified to perform operando MAS NMR experiments under continuous flow conditions. The feasibility of this probe design for studying heterogeneous catalysis reactions under flow conditions was demonstrated by studying hydrogenation of toluene on Pt/sulfated zirconia. This probehead design permits the simultaneously analysis of the collected products while recording the spectra, while the reaction occurs in the NMR rotor. Although improvements are possible, we believe this probe design is a useful piece of equipment for studying heterogeneous catalytic reactions under relevant conditions.
These studies show that the reactant diffusion and interaction with the catalyst in MAS NMR micro-reactor are good, this reactor performing similarly to fixed bed catalytic reactor.
Improvements are currently made mainly concerning the materials used for the flow gas injection system, and also to be able to reach higher temperatures.
Acknowledgments
The authors thank Région Basse-Normandie for financial support. The authors are very grateful to Michael Hunger (Stuttgart, Germany) for his help in the design of the probehead. We also thank Dr. Jacob van Gestel (Laboratoire Catalyse et Spectrochimie, Caen, France) for fruitful discussions about this work.