1 General framework
No pigment has been as difficult to characterise as Maya blue. Palygorskite, then sepiolite [1], have been identified on archaeological samples as a major component, but the reason for the blue colour could not be put in evidence. Kleber et al. [2] proposed the presence of indigo, and supposed that indigo might be able to substitute for water in these clays. But nobody has succeeded in extracting indigo from Maya blue with solvents. Kleber et al. were right, because, using Raman microspectrometry, Guineau [3] showed the presence of indigo in Maya blue samples from Dzibilnocac. From the study of palygorskite and sepiolite/indigo complexes, Hubbard et al. [4], using TGA, XRD and RMN concluded that indigo molecules chemisorb, not inside the tunnels, but at their edges, near the external surfaces. A contrario, following a different approach, Chiari et al. [5] and Fois et al. [6] have shown by molecular modelling that indigo molecules could locate and find stable positions in the nanotunnels of palygorskite. Chiari computes adsorption enthalpies onto the internal surfaces of clay, for water and indigo, respectively: indigo cannot displace water, but can substitute for it once the water molecules have been driven away. Their computations support the hypothesis of a hydrogen bonding of indigo to the clay hydroxyls through its C=O groups, the N–H groups remaining free. Finally, Leona et al. [7] have studied Maya blue made from palygorskite by Raman, UV–visible and Fourier transform infrared (FTIR) spectroscopic techniques. Their results seem to confirm Chiari's hypothesis. We do not agree with the proposal of José-Yacamán et al. [8] to consider iron oxide and silica nanoparticles, and the presence of a super lattice, as the true cause for the colour of Maya blue.
Our study concerns the Maya blue made from sepiolite. We observe the evolution of the FTIR spectrum of indigo after the preparation of Maya blue through heating a blend of sepiolite and indigo. The changes are correlated with the departure of the differently bound molecules of water initially present in sepiolite, characterised through TGA.
The interesting features of the FTIR spectrum are as follows. It is well established that indigo molecules are strongly associated, especially in the solid state [9–13]. The molecule is in a trans configuration stabilised by the nitrogen atoms linked to the closest ketone group in the same molecule (intramolecular bonds), but they are also linked to the ketone group of neighbouring molecules. Infrared bands at 1394 and 1408 cm−1 corresponding to the NH in plane bending mode are considered to arise from intermolecular and intramolecular interactions [9,10]. Fortunately, these N–H bands are not hidden by the absorption bands of sepiolite, and remain observable on the Maya blue FTIR spectrum.
2 Experimentation
Synthetic indigo was provided by Aldrich. Its content in crystallised indigo is 95%. It was used to obtain the FTIR reference spectrum.
Pure sepiolite was obtained from Tolsa (S9 grade). It was observed at high magnification by TEM. The half-unity cell of sepiolite is (Si)12(Mg)8(O)30(OH)4(OH2)48H2O [14,15] and the tunnel dimensions are 1.06 × 0.37 nm. The sepiolite was used without special preparation.
In accordance with previous studies [16], dry sepiolite and indigo powders were mixed to obtain a 3% weight indigo blend.
The chemical interactions in Maya blue were studied by FTIR spectroscopy (Perkin Elmer spectrometer Paragon 1000). Sepiolite, indigo and Maya blue were characterised using diffuse reflection infrared spectroscopy (DRIFT, minidiff plus diffuse reflectance acquired from Eurolabo). The powder mixed with KBr was directly placed in the cup and the background spectrum of KBr was subtracted. All the reflectance spectra were taken at a resolution of 4 cm−1 for 16–64 scans from 4000 to 500 cm−1.
Thermal gravimetric analysis (TGA) was performed on a SETARAM TGDT A 92. The powder, approximately 10 mg, was heated from room temperature to 950 °C at a rate of 5 °C min–1. Experiments were carried out under artificial dry air (N/O2 = 80/20). According to the literature, under our experimental conditions, a weight loss corresponds to water departure of the various types of water molecules [17].
Colorimetric measurements were performed with a Minolta Chromameter CR-200b device.
3 Sepiolite thermal dehydration study
Before making Maya blue, we must determine the thermal ranges of the water departures out of sepiolite. We use TGA.
Fig. 1 shows that water departure proceeds in different stages. When the temperature increases from ambient to 130 °C, the first stage (I) is an important weight loss (10%) corresponding to the departure of zeolitic water located in the tunnels and water adsorbed on surfaces. The second stage (II) ranges from 130 to 300 °C. This 3% weight loss corresponds to the departure of one of the two magnesium bound water molecules (coordinated water). In the third stage (III), between 300 and 550 °C, the second water molecule leaves. Sepiolite is dehydrated. Until 550 °C, the dehydration is reversible. Above 550 °C, the constitutive water (hydroxyl groups associated with the octahedral sheet) is removed, and one observes the non-reversible destruction of the sepiolite structure.
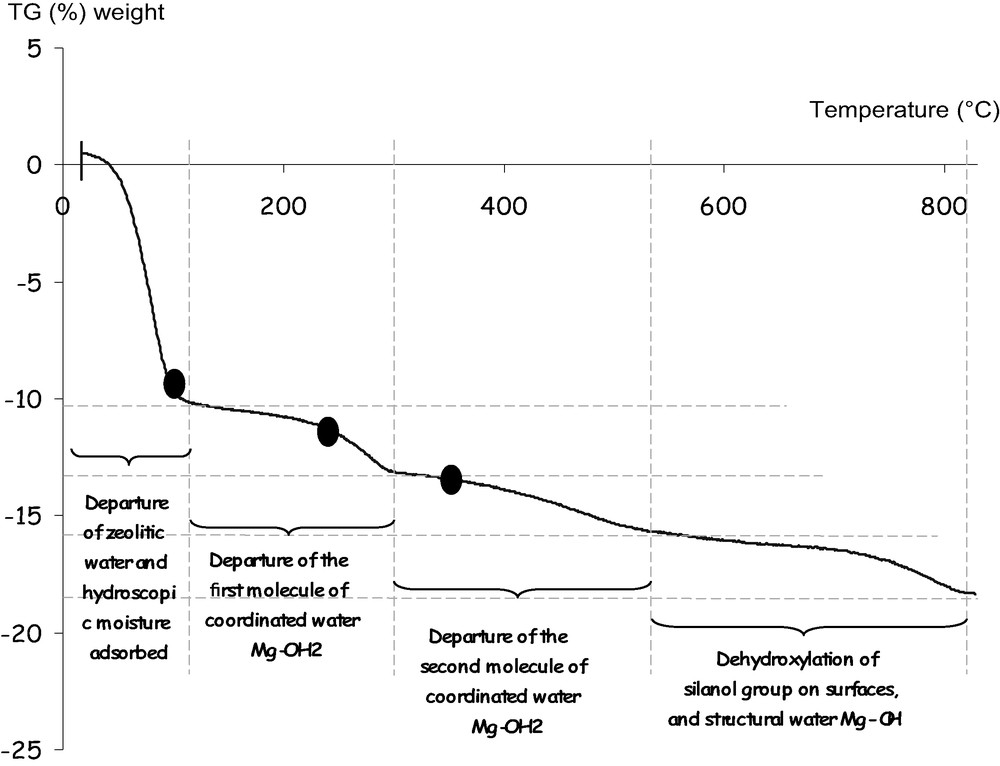
Thermogravimetric analysis of a sepiolite. Water departure proceeds in stages corresponding to the different types of water.
4 Maya blue preparation
In temperature ranges I–III, we chose a set of heating temperatures to prepare Maya blue: 120 °C for the stage I; 180, 220 and 280 °C for the stage II; for stage III: 310, 350, 400, 450 and 500 °C. The initial blend of sepiolite and indigo was heated in air. Samples were set apart minute by minute to constitute a data base.
Colorimetric measurements brought out the kinetics of the phenomenon and permitted us to select typical conditions to obtain reference samples of Maya blue with fully developed shade. So we chose study samples after heating for 30 min to be sure of having reached thermodynamical equilibrium.
5 FTIR study
The next step consists in observing the evolution on the FTIR spectrum of inter- and intramolecular hydrogen bonding bands of the pure indigo (respectively, 1408 and 1394 cm−1).
On the samples prepared at temperatures up to 130 °C (stage I), no changes were observed. The NH inter and intramolecular bands remain identical, as in pure indigo (Fig. 2). The sepiolite colour turns to a light turquoise blue. Indigo can be extracted from the blue powder by tetrahydrofuran (THF) washing at room temperature. The contact with boiling nitric acid induces a discolouration, more pronounced for the 5-min-heated sample than for the 20 h sample. Moreover, a 6-month ageing of the powder in the presence of light and air shows a certain fading of the shade. The interaction of indigo and sepiolite is not sufficient to obtain a true Maya blue.
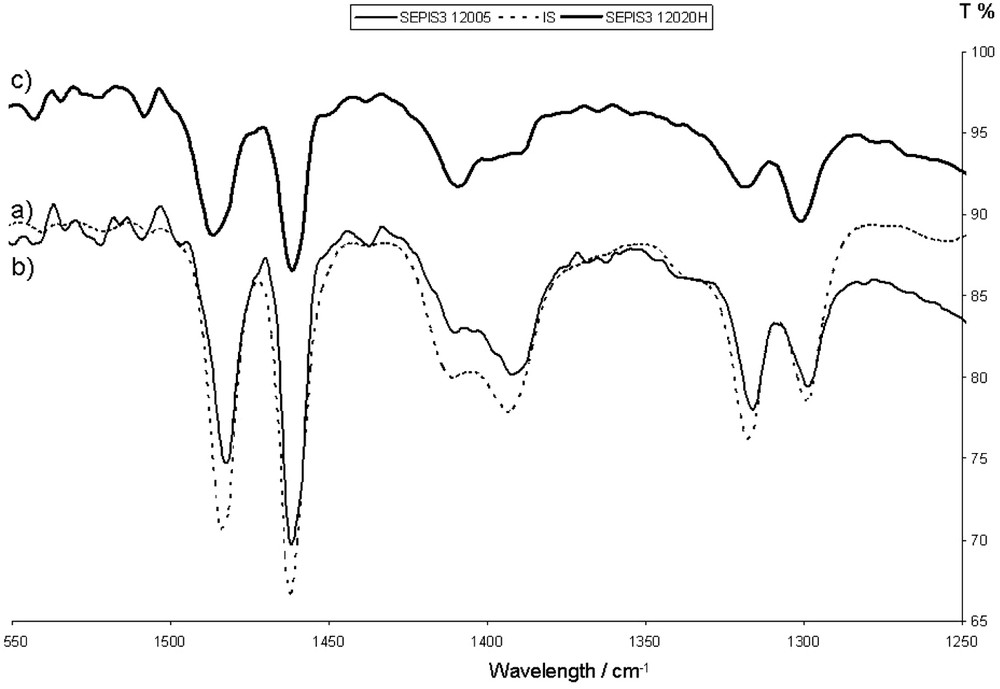
FTIR. (a) Part of a pure indigo spectrum (dotted line) bringing out the two peaks corresponding to intermolecular (1394 cm−1) and intramolecular (1408 cm−1) bonds. The other spectra (b and c) correspond to the indigo/sepiolite blend heated at 120 °C during 5 min and 20 h, respectively. The two peaks remain quite constant.
With the samples prepared in the 130–300 °C range (stage II), the IR band corresponding to intermolecular bonds peak at 1394 cm−1 vanishes (Fig. 3). The shade remains turquoise blue, but is more saturated. Indigo cannot be extracted from the blue powder by THF washing at ambient temperature. The colour remains stable in contact with boiling nitric acid. After the 6-month ageing of the powder in the presence of light and air, most of the samples do not show any fading. The bonding of indigo to sepiolite is sufficient to obtain a Maya blue.
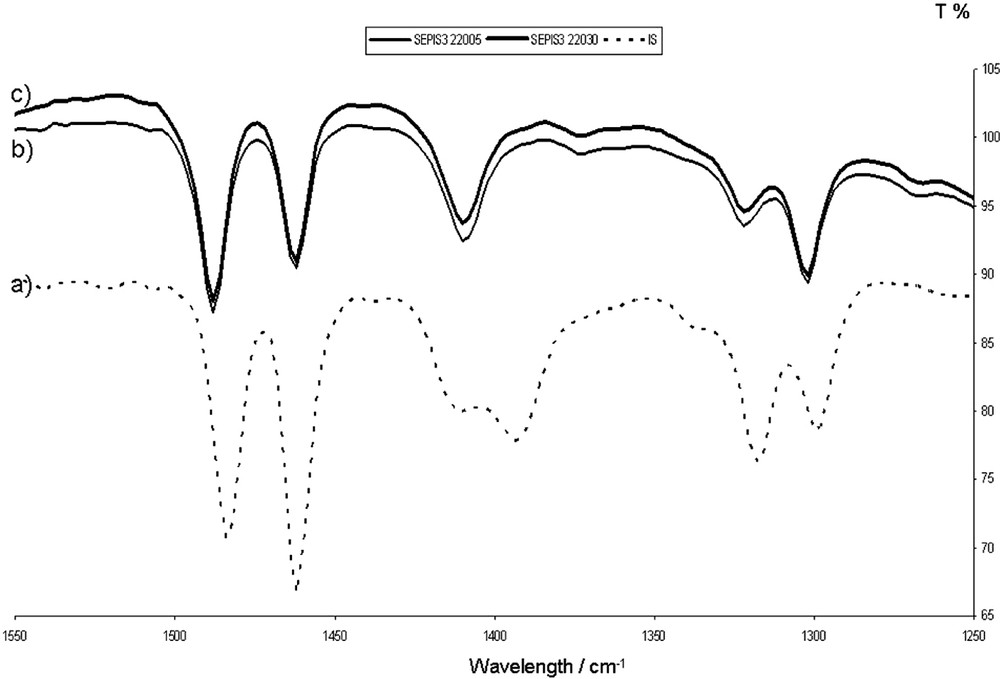
(a) FTIR spectrum of pure indigo. (b, c) FTIR spectra of the indigo/sepiolite blend heated at 220 °C during 5 and 30 min, respectively. The 1394-cm−1 peak (intermolecular bonds) has disappeared.
On the samples prepared in the 300–550 °C range (stage III), Fig. 4 shows that the band corresponding to intramolecular bonds at 1408 cm−1 disappears also. Simultaneously there appears a new peak at 1498 cm−1. The colour is now blue, without a green shade. It is perfectly stable with respect to THF washing, boiling nitric acid test or the 6-month ageing through light exposition.
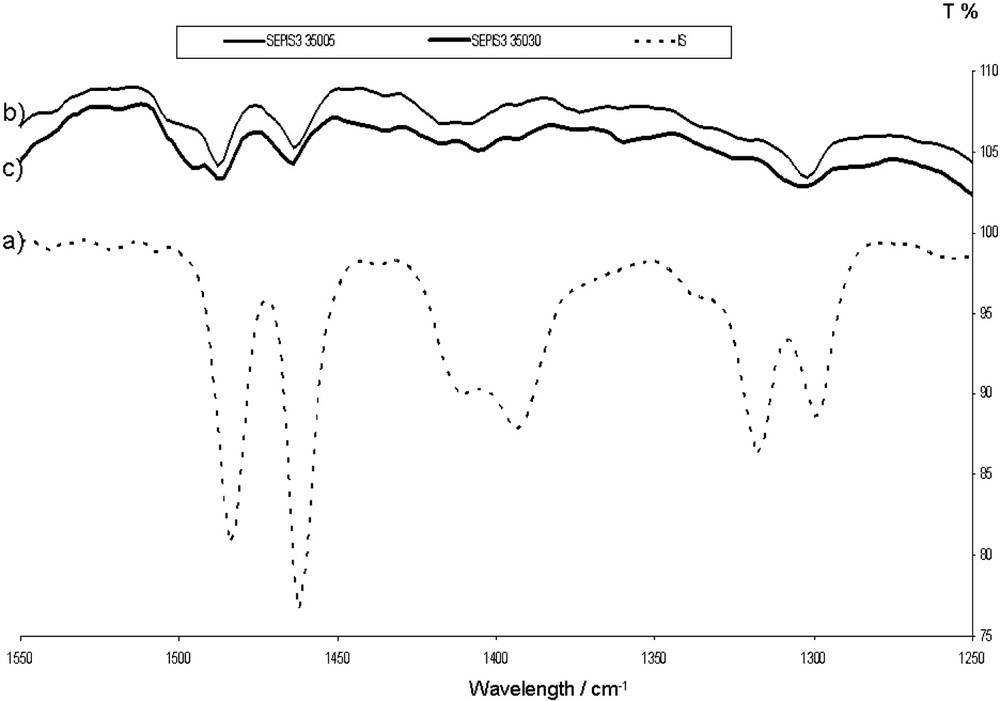
(a) FTIR spectrum of pure indigo. (b), (c), FTIR spectra of the indigo/sepiolite blend heated at 350 °C during 5 and 30 min, respectively. Both 1394 and 1408 cm−1 peaks (inter and intramolecular bonds) have disappeared. A new peak has appeared at 1498 cm−1.
6 Interpretation
When the heating temperature is below 130 °C, indigo molecules, wherever they may be, remain associated. Inter and intramolecular bonds exist, hence there are no new bonds created with sepiolite surfaces (external or internal). That is in good accordance with the reversibility of the process.
In the second temperature range, between 130 and 300 °C, intermolecular bonds vanish. Indigo trimers and dimers are dissociated into monomers capable of diffusing more easily. Nitrogen molecular orbitals released by the breaking of intermolecular links bond to sepiolite, probably via aqua-ligand magnesium or just magnesium. Let us remember that in this range of temperature, the first water molecule bonded to magnesium is lost, which can let the magnesium interact with indigo molecule. Hence the non-reversibility of the process.
In the third temperature range, between 300 and 550 °C, the intramolecular bonds disappear, which suggests that the indigo polar groups create new bonds with sepiolite surfaces, whether external or internal, possibly also via magnesium.
In this temperature range, there is a stronger indigo/sepiolite bond. Is it not strange that indigo–sepiolite bonding should increase with temperature? If, as in the case of the indigo/palygorskite compound, indigo molecules must wait for water departure to bond to the clay, it remains that indigo can only establish its new bonds with sepiolite surfaces, whether external or internal. Chemisorption theories indicate that, at constant adsorption enthalpy, an increase in temperature causes a decrease of the mean residence time of an adsorbed molecule on a surface. External adsorbed indigo molecules would desorb, which is not the case. So, chemisorption cannot take place on the external surfaces. The adsorption of indigo on sepiolite can only be possible onto the internal surfaces, inside the nanotunnels. This explains the atypical high thermal stability of this organo mineral compound, and also the fact that the indigo cannot be extracted from the sepiolite with any solvent. That is why the blue complex, the so-called Maya blue, is remarkably stable in time.
We can deduce that, for the temperature ranges II and III studied, indigo chemisorbs onto the sepiolite tunnel internal surfaces, and never on the sepiolite external surfaces.
7 Conclusion
Using FTIR, we have shown that indigo molecules could bond to sepiolite surfaces in the 180–550 °C range. The adsorption process closely follows the departure of magnesium-coordinated water. Hence, the higher the temperature, the stronger the bonding of indigo to the sepiolite. The simplest explanation for this atypical behaviour lies in the diffusion and chemisorption of indigo inside sepiolite nano tunnels. This is in good accordance with the unusual chemical stability of Maya blue with respect to indigo solvent and nitric acid.
So indigo behaviour seems to be similar with sepiolite and palygorskite, which explains their traditional use for making Maya blue.