1 Introduction
The nuclear industry and the progress in the related research and development programs produces high-level liquid wastes containing radionuclides such as actinides and fission products, causing long-term storage problems. One interesting treatment route to solve the actinide question consists in implementing a solid–liquid extraction step followed by an actinide recovery stage or an actinide immobilisation stage (Fig. 1). In this frame, hybrid silica gels obtained by sol–gel techniques [1] under mild conditions are suitable candidates as solid extractants.
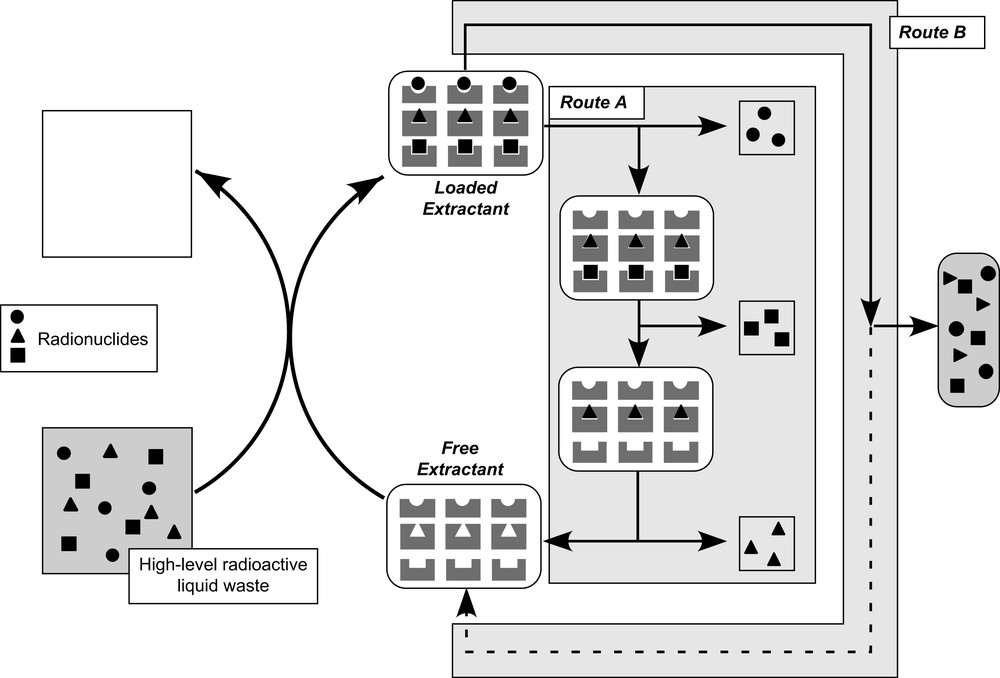
HLLW Treatment: route A leads to a radionuclide recycling, while route B leads to the confinement of the radionuclide in a solid matrix with possible recycling of the solid extractant.
This versatile synthetic approach allows us to take advantage of molecular engineering to modify the silica skeleton by introducing organic functions (such as ligands or extractants) and structural blocks during the synthesis to control the overall reactivity, the texture and the structure of the hybrid material [2]. By this way a wide variety of materials can be produced according to the intrinsic properties of the organic function [2a,j,k]. The incorporation of functionalised ligands opens interesting possibilities for metal complexation or extraction [3].
The mild conditions of the sol–gel approach also allow the tuning of the material properties by the loading of the complexing moieties. Finally, imprinting techniques can also be used in this frame for overall structure–properties relation adjustments [2g,3d,4]. In such a way, actinide extraction as well as catalyst support enhancement has been described [3d,e,5].
In this work we report the synthesis of polyamine-polyether- and pyridine-functionalised hybrid silica materials and their extraction properties for plutonium and americium from nitric acid solutions, with highlights on the influence of the chemical pattern, the textural aspects and the extractant loading on the extraction properties.
The synthetic route leading to the materials consists of the hydrolysis and the condensation between Si(OEt)4 and the functionalised LSi(OEt)n (Fig. 2) where the organic functions L (Fig. 3) were chosen for their ability to complex several metallic cations [6].

Hybrid silica synthetic route.

Triethoxysilylated ligands used to build hybrid silicas.
All the materials obtained by this technique are given in Table 1.
Synthesised hybrid materials
Hybrid silica material | X | Gel reference |
5 | G1a | |
10 | G1b | |
5 | G2a | |
10 | G2b | |
10 | G3 | |
0 | G4a | |
5 | G4b | |
10 | G4c | |
0 | G5a | |
5 | G5b | |
10 | G5c | |
2 | G6a | |
5 | G6b | |
10 | G6c | |
2 | G7a | |
3 | G7b | |
4 | G7c | |
5 | G7d | |
6 | G7e | |
10 | G7f |
A first series of solid–liquid extraction tests were done to establish the best organic function for the actinide extraction, then further studies on the most efficient remaining best-functionalised material were carried out to determine the importance of the loading and the insertion mode of the coordinating organic function on the extraction of Am(III), Pu(IV) and Eu(III) from nitric acid solutions.
2 Results and discussions
These studies are essentially focused on the determination of the distribution coefficients for selected functionalised hybrid materials and will be discussed on a qualitative level.
Owing to the nature of the americium and plutonium species in nitric acid media [7] and the basic nature of the ligands, in particular the amine and the pyridine group, the extraction studies were performed over a large range of nitric acid concentration, usually [HNO3] varying from 10−3 M up to 4 or 6 M. In these operating conditions, americium will be found in oxidation state (III) and plutonium in oxidation state (IV).
2.1 Preliminary scanning for Am(III) extraction
Preliminary experiments were performed to determine the distribution coefficient for a first series of hybrid materials functionalised with polyether, pyridine and diaminoethylene groups with 0.018 M nitric acid solution. The results show that only some diaminoethylene gels extract americium with Kd(Am) values ranging from some hundreds to some thousands mL/g (Table 2a).
Results of preliminary Am(III) distribution coefficient scanning for different functionalised hybrid materials; [HNO3] = 0.018 M
Polyether | Gel | G1a | G1b | G2a | G2b | G3 | |
Kd (mL/g) | 1.1 | 1.8 | 0.8 | 1.6 | 2.7 | ||
Pyridine | Gel | G4a | G4b | G4c | |||
Kd (mL/g) | 2.0 | 0.9 | 2 | ||||
Polyamine | Gel | G5a | G5b | G6b | G6c | G7d | G7f |
Kd (mL/g) | 338 | 3.3 | 2822 | 2.3 | 2226 | 0.9 |
Polyether and pyridine, usually known for their complexing properties for several metals at low acidity do not extract americium in our conditions. The low Kd(Am) values usually point out a very low affinity of the functionalised material for the americium. The structural organisation of the potential complexing sites inside the condensed matter is not favourable to the coordination of the americium species, despite a high number of coordination functions introduced in the silica skeleton, such as in gel G4a. The source of this inefficient organisation of the extractant sites in the polyether and pyridine hybrid materials is probably due to an inadequate self-assembling of the functionalised precursor in the solution during the condensation process, producing a negative template effect leading to non-coordinating sites for americium.
Americium distribution coefficient measurements for gels G1a–G4c over a range of nitric acid concentration from 0.018 M to 1 M show no significant changes in the extraction behaviour (Table 2b).
Distribution coefficient Kd (mL/g) for Am(III) extraction from nitric acid for polyether (G1a–G3) and pyridine (G4a–c) functionalised materials
[HNO3] (M) | Polyether | Pyridine | ||||||
G1a | G1b | G2a | G2b | G3 | G4a | G4b | G4c | |
0.0188 | 1.1 | 1.8 | 0.8 | 1.6 | 2.7 | 2.0 | 0.9 | 2.0 |
0.05 | 1.2 | 2.3 | 1.1 | 1.8 | 1.8 | 2.2 | 2.8 | 2.4 |
0.075 | 1.1 | 1.8 | 0.3 | 1.9 | 1.9 | 1.8 | 1.3 | 2.6 |
0.1 | 1.7 | 1.2 | 0.8 | 1.4 | 1.4 | 1.8 | 1.6 | 2.3 |
0.5 | 1.3 | 1.1 | 1.1 | 1.3 | 1.3 | 1.5 | 1.7 | 2.4 |
1 | 1.2 | 1.2 | 0.9 | 1.6 | 1.6 | 1.8 | 1.3 | 2.8 |
These observations show clearly that the change of the speciation for americium does not affect the magnitudes of the distribution coefficients. In any case, this means that americium will be never coordinated.
Further studies were essentially carried out on polyamine-functionalised hybrid materials to determine the influence of structural and extractant loading aspects on the extraction properties of americium and plutonium.
2.2 Am(III) extraction by polyamines
2.2.1 Influence of the polyamine pattern
Gels G6b and G7d show very high coefficient distribution, respectively, around 3 × 105 mL/g and 7 × 104 mL/g, for a nitric acid concentration less than 0.01 M (Fig. 4). This value drops drastically to less than 10 mL/g for [HNO3] higher than 0.1 M.
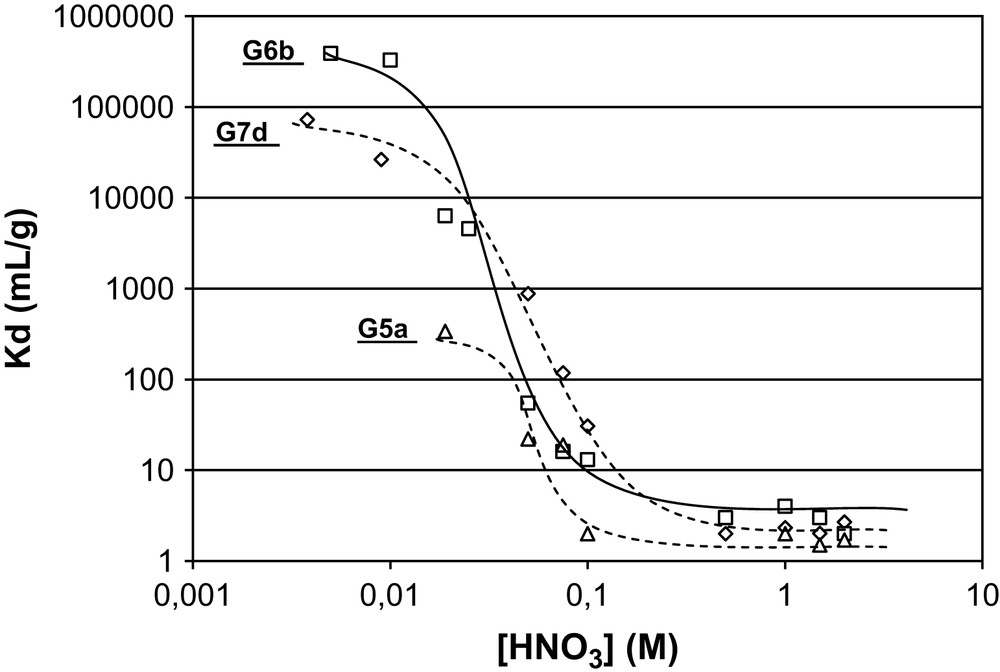
Kd(Am) for gels G5a, G6b and G7d for [HNO3] ranging from 0.0038 M to 2 M.
The same behaviour is observed for gel G5a with much lower Kd values. This type of results is usually observed in the case of a typical metallic complexation behaviour. In fact, at low acidity, the non-protonated amino functions are able to coordinate the americium core with high affinity, leading to a high value of the distribution coefficient. At higher [HNO3] concentration, the competitive extraction of H+ by the amino group as well as the complexation of the Am(III) cation by the nitrate anion in the solution, is more efficient than the complexation of the Am(III) by diaminoethylene moieties, decreasing the distribution coefficients.
It means that Am(III) is extracted as neutral complex. Preliminary comparative EXAFS studies on Nd(NO3)3·6H2O and Nd(NO3)3(ethylenediamine) extracted by the gels G5a, G6b, G6c, G7a and G7b1 and the speciation of americium in this nitric acid range let us expect that for the extracted complex, one nitrate remains probably in the first coordination sphere, while hydroxide ligands guarantee neutrality and the ethylenediamine pattern coordinate as chelate Am(III). The fact that the gels G5a and G6b show high Kd(Am) means that the ethylenediamine pattern is sufficient for extraction of Am(III) compared with the triaminodiethylene moiety. No significant differences are observed between hybrids G6b and G7d. The low Kd(Am) for gel G5a compared with hybrids G6b and G7d lets us assume that the complexing pattern needs to be cleared of some conformational restrictions for efficient extraction. In gel G5a, the doubly bonded ligand to the silica skeleton is probably too structurally constrained for effective coordination showing lower affinity for Am(III), while in the case of the G6b and G7d prepared from the monosilylated diamino and triamino ligands, these pendant ligands are more flexible in the hybrid material.
2.2.2 Influence of the ligand loadings
The influence of the extractant loading on the americium distribution coefficient from dilute nitric acid media was studied for the free diaminoethylene-functionalised hybrid materials G6a–c, G7a and G7c–f (Fig. 5).
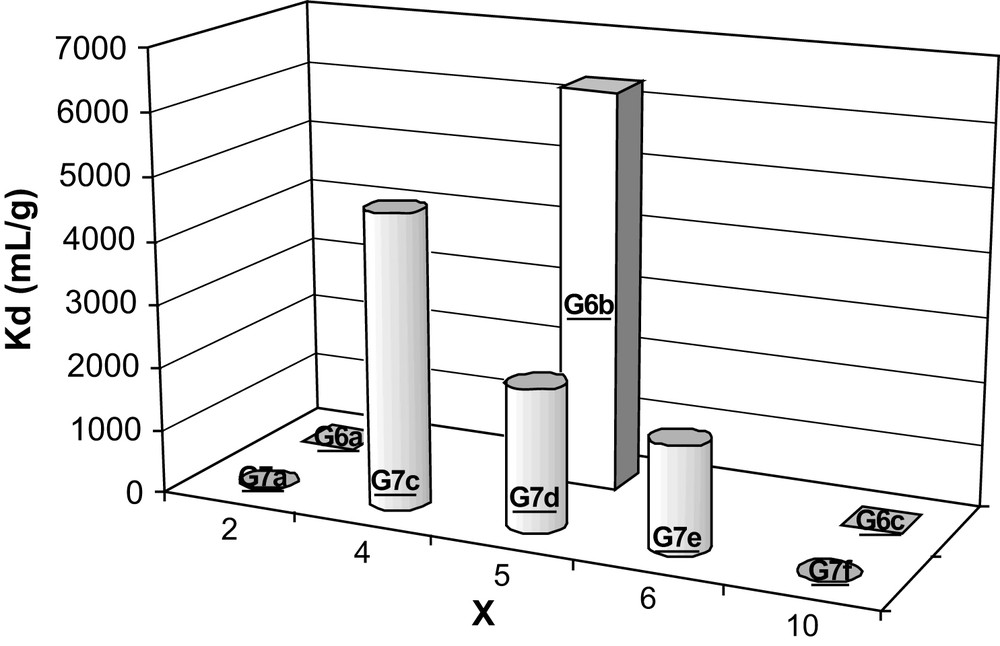
Effect of the extractant loading on the Am(III) distribution coefficient. X is the ratio Si(OEt)4/Si(OEt)3L/expected after synthesis. [HNO3] = 0.018 M.
In these experiments, the initial ratio between the functionalised molecular precursor, LSi(OEt)3, and the tetraethoxysilane Si(OEt)4 is modulated, producing hybrid silica gels with varying extractant loading with Si/L from 3 to 11.
The measurement of the Kd(Am) shows high values for hybrids G6b, G7c, G7d and G7e. This corresponds to the gel with observed Si/L ratios of 5, 6 and 7. A low Kd(Am) value for the high Si/L ratio is somewhere expected owing to the dilution of the extraction function during the condensation process in the material, although a value around 2 is a surprisingly very low value. Also for the material with a high extractant loading such as hybrids G6a and G7a, the Kd(Am) value is less than 3.
These strong differences for the Kd(Am) values are usually characteristic of a strong affinity variation for the americium. The structural organisation of the complexing site inside the materials will be probably at the origin of this observation. The structure of the site in the final material is greatly dependent on the organisation in solution of the molecular silicon species during the condensation process as well as the collapse process which occurs during the drying stage. Most likely, the heavy extractant loading materials G6a and G7a have a strong organic nature which will be easily reorganised during the drying period, leading to non-complexing sites, even when the high Si/L ratio for the light extractant loading G6c and G7f materials is favourable to a surrounding of the autoassembled organic function by a silica layer, shielding the complexing sites. The medium extractant loaded materials G6b and G7c–e will probably have a rigid enough mineral character avoiding a site collapse as well as a Si/L ratio capable of generating surface accessible complexing sites.
However, the measured textural parameters (pore mean diameter, pore volume and specific surface areas) show clearly no influence on the distribution coefficient for Am(III) (Table 3).
Pore volume (Vp), specific surface areas (S) and pore diameter (Øp) for diaminoethylene based hybrid materials by BET calculation and the Kd(Am) for [HNO3] = 0.018 M
Gel | Vp (mL/g) | S (m2/g) | Øp (Å) | Kd(Am) (mL/g) |
G5a | – | 4 | – | 338 |
G5b | 0.84 | 382 | 87 | 3.3 |
G5c | 1.04 | 287 | 144a | 0.5 |
G6a | – | 9.6 | – | 2.0 |
G6b | 0.97 | 269 | 145 | 6291 |
G6c | 1.32 | 354 | 148 | 2.3 |
G7a | – | 9.2 | – | 3.1 |
G7d | 0.39 | 110 | 142a | 2226 |
G7f | 0.32 | 109 | 118 | 2.0 |
a macroporous.
Hybrid G5a has a specific surface area of 3 m2/g with a Kd(Am) of 338 mL/g, whereas gels G5b and G5b, which are not able to extract Am, exhibit high specific surface areas, respectively, 382 m2/g and 287 m2/g. For the gels G6b and G7d, the specific surface areas are, respectively, 269 m2/g and 110 m2/g and the Kd(Am) of 6291 mL/g and 2226 mL/g. From the same family, the hybrids G6c and G7f have a specific surface area of the same order of magnitude, respectively, 353 m2/g and 109 m2/g with a Kd(Am) of 2.3 mL/g and 2.0 mL/g. It looks likely that the Si/L ratio plays an important role in the extracting properties of the solids.
2.3 Am(III) and Eu(III) concomitant extractions
Aiming Am(III)/Eu(III) separation, distribution coefficient measurements for these two elements were done for hybrid material G7d, which shows a good extraction behaviour for Am(III). A solution containing a mixture of Am(III) and Eu(III) (see Section 4) was used for all extraction experiments (Fig. 6).
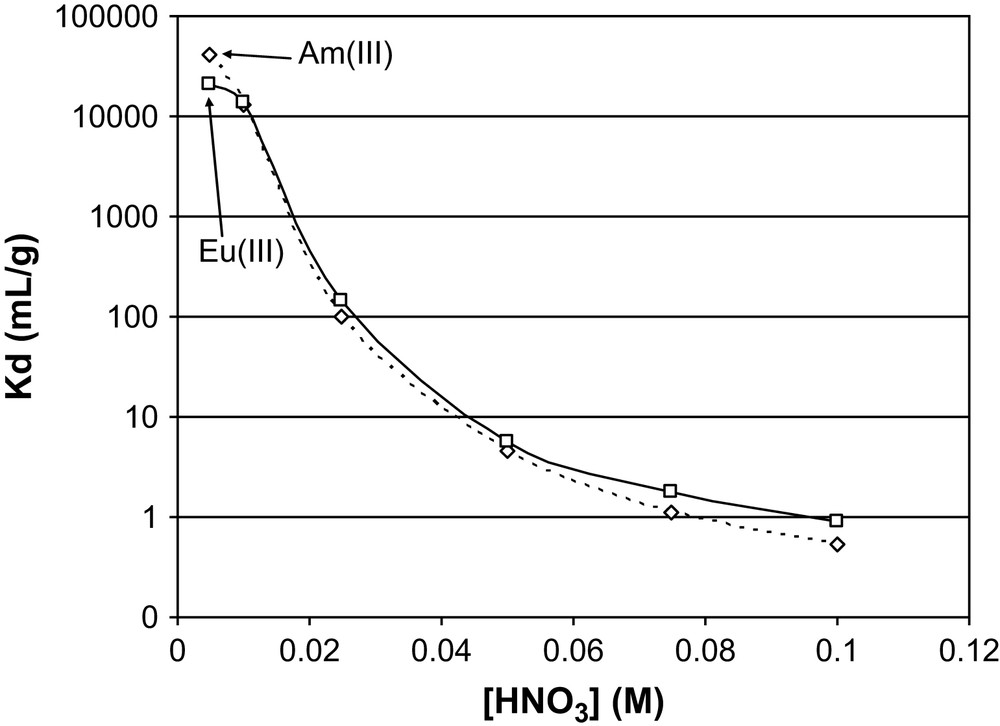
Distribution coefficients of Am(III) and Eu(III) vs nitric acid concentration for gel G7d.
Unfortunately, no significant differences for the distribution coefficient values were observed whichever the HNO3 concentration may be. In these conditions the behaviour of both elements is identical, preventing an efficient separation. The material needs to be improved in order to increase the separation factor between Am and Eu. This can be envisaged by the use of template effects like the imprinting techniques using metal complexes, successfully undertaken for diamide hybrid material to increase not only the overall extraction performance, but also the separation factor between Eu, Nd and Am [3d].
2.4 Pu(IV) extraction by polyamines functionalised materials
The distribution coefficient for Pu(IV) in nitric acid for hybrid material G7d was measured to determine fundamental differences between two actinides, such as Pu(IV) and Am(III), showing different speciation along a nitric acid concentration range [7]. To avoid plutonium hydrolysis problems, the study has to be limited to a nitric acid concentration ranging from 0.5 M to 6 M.
For gel G7d, the plutonium experiments show a maximum distribution coefficient around 66 mL/g, which is very low compared with the americium experiments where the maximum distribution coefficient is between 104 mL/g and 105 mL/g (Fig. 7). This value of 66 mL/g is observed for [HNO3] from 4 M to 6 M. At these acidities, Pu(IV) nitrate species exist as Pu(NO3)5− and Pu(NO3)62− anions, while the ethylenediamine functions form protonated cationic entities. Under these conditions, the extraction mechanism of Pu(IV) is certainly an anion exchange process. This is confirmed by the low [HNO3] back extraction conditions where Pu(IV) forms a neutral tetranitrate complex.
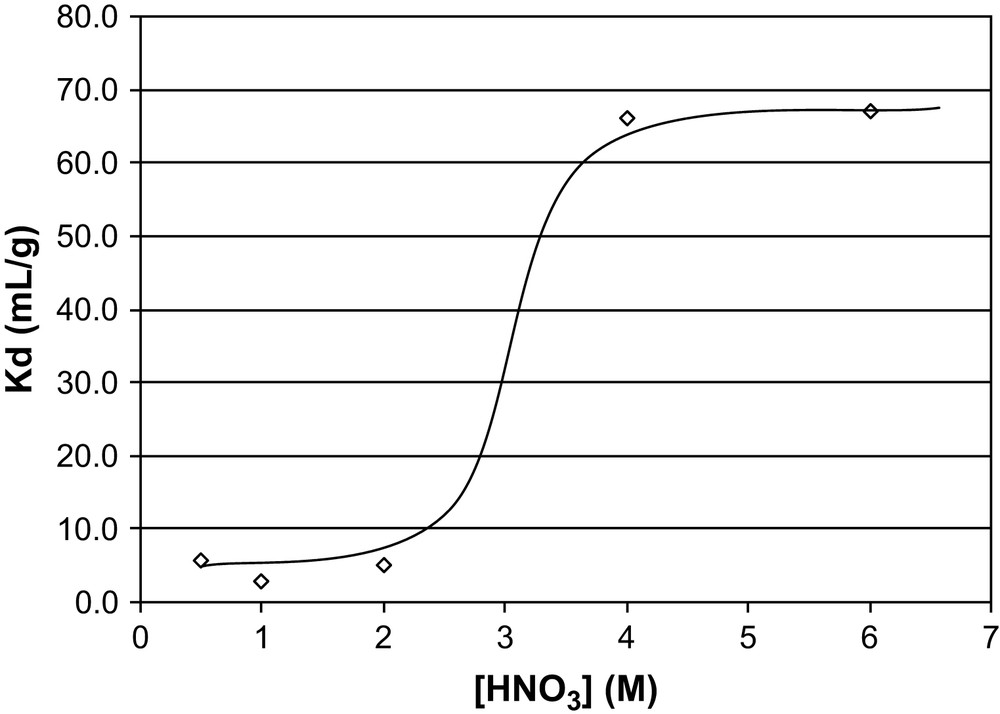
Distribution coefficient for gel G7d for P(IV).
3 Conclusions
In this work it is shown that the synthesis of hybrid materials using sol–gel techniques is suitable to obtain high-performance solid material to extract americium from low-concentrated nitric acid solutions. A first tuning of the extraction properties can be done by the use of different chemical patterns and through the control of the extractant loading in the material. Extended control of the extraction behaviour can be considered by the use of molecular engineering such as template techniques.
We have also synthesised hybrid materials having two extraction mechanisms for two different actinides:
- - metal complexation at low acidity for Am(III);
- - anion exchange at high acidity for Pu(IV).
4 Experimental
All experiments related to the synthesis of the hybrid material were carried out under nitrogen atmosphere. Ethanol was dried by distillation over magnesium and under nitrogen atmosphere before use. All reagents and functionalised triethoxysilane (1–7) were available commercially from Gelest™ or Sigma-Aldrich™ and used without further purification.
241Am, 152Eu and Pu were provided by CEA (France) and, after purification by chromatographic methods [8], starting solutions were prepared. The americium experiments were performed using a solution with [Am] = 0.0261 g/L and [HNO3] = 0.037 M. The plutonium experiments were realised using a solution with [Pu] = 0.323 g/L and [HNO3] = 0.111 M. For the americium–europium experiments, the 152Eu tracer was added to an Am/Eu solution with [Am] = 0.029 g/L, [Eu] = 0.016 g/L and [HNO3] = 0.01 M.
Infrared spectra were recorded on a PerkinElmer FT 1000. Solid-state NMR was performed on a FTAM 300, with a 29Si frequency of 59.62 MHz, an acquisition delay of 10 s, a rotation speed of 5000 Hz and from 300 up to 18,000 scans. Chemical shifts δ were in ppm with TMS as internal reference. Specific surface, pore volume and mean pore diameter were determined by BET calculation after nitrogen adsorption–desorption measurements on a Micrometrics 2375 or a Coulter SA 3100 apparatus. Alpha activity was determined on a Camberra model 7401 and gamma counting was performed on a Cobra II counter, 59 keV for 241Am and 122 keV for 152Eu.
All batch distribution coefficient measurements' experiments were realised in 2-mL microtubes. A mixture of 1 mL of the metallic solution and 50–100 mg of hybrid material was shaken for 15 min. After filtration by centrifugation over a 45-μm filter, the filtrate was analysed by alpha or gamma counting. Before all extraction experiments, the material was contacted with a solution showing the same HNO3 concentration.
In solid–liquid extraction, the distribution coefficient for a given metal (Kd) is defined as the ratio of the amount of the metal in the solid to the amount of the metal in the solution at equilibrium, if the amount of the extracting group in the solid is assumed to be constant. This is achieved by using initial concentrations of metal around 0.1% of the total amount of extracting group in the solid. The initial and final concentrations of metal in solution were determined by alpha counting for the Am and Pu experiments and gamma counting for the Am and Eu experiments. Kd is given by the formula:
The hybrid silicas were obtained according to the same general procedure [3f]: in a Schlenck tube, the chosen precursor (from 1 to 7) and Si(OEt)4 are added (in the desired ratio) to dry ethanol, with a concentration of Si around 1 M. Under magnetic stirring, the corresponding amount of water is added (0.5 mole equivalent/mole of ethoxy group). The condensation process stars in less than 12 h. After an aging period of one week, the solid is dried under vacuum at 80 °C, washed with acetone and diethylether and dried again under vacuum at 80 °C for 24 h.
4.1 Selected experimental data for some polyamines functionalised materials
4.1.1 Hybrid G5a
White powder; IR (KBr, cm−1): νSi–O = 1086; νC–H = 2939; NMR 29Si CP-MAS (δ, ppm): −58.9 (T2), −66.1 (T3); BET S = 4 m2/g.
4.1.2 Hybrid G5b
Yellow powder; IR (KBr, cm−1): νSi–O = 1119; νC–H = 2957 and 2854; NMR 13C CP-MAS (δ, ppm): 9.5 (CH2Si), 22.4 (CH2CH2CH2), 50.4 (CH2N); NMR 29Si CP-MAS (δ, ppm): −57.2 (T2), −63.1 (T3), −100.9 (Q3), −108.3 (Q4); BET S = 382 m2/g, Vp = 0.84 cm3/g, Øp = 87 Å.
4.1.3 Hybrid G5c
White powder; IR (KBr, cm−1): νSi–O = 1109; νC–H = 2953 and 2851; NMR 13C CP-MAS (δ, ppm): 9.5 (CH2Si), 22.4 (CH2CH2CH2), 48.9 (CH2N); NMR 29Si CP-MAS (δ, ppm): −57.9 (T2), −64.3 (T3), −101.1 (Q3), −108.4 (Q4); BET S = 287 m2/g, Vp = 1.04 cm3/g, Øp = 144 Å.
4.1.4 Hybrid G6a
White powder; IR (KBr, cm−1): νSi–O = 1095; νC–H = 2940; NMR 29Si CP-MAS (δ, ppm): −65.8 (T3), −101.3 (Q3), −109.6 (Q4); BET S = 9.6 m2/g.
4.1.5 Hybrid G6b
White powder; IR (KBr, cm−1): νSi–O = 1120; NMR 13C CP-MAS (δ, ppm): 9.5 (CH2Si), 21.4 (CH2CH2CH2), 40.3 (CH2N), 50.6 (OCH3); NMR 29Si CP-MAS (δ, ppm): −66.4 (T3), −101.4 (Q3), −108.4 (Q4); BET S = 269 m2/g, Vp = 0.97 cm3/g, Øp = 145 Å.
4.1.6 3Hybrid G6c
White powder; IR (KBr, cm−1): νSi–O = 1115; NMR 13C CP-MAS (δ, ppm): 9.5 (CH2Si), 21.4 (CH2CH2CH2), 50 (CH2N); NMR 29Si CP-MAS (δ, ppm): −64.0 (T3), −101.0 (Q3), −106.9 (Q4); BET S = 354 m2/g, Vp = 1.32 cm3/g, Øp = 148 Å.
4.1.7 Hybrid G7a
White powder; NMR 13C CP-MAS (δ, ppm): 8.9 (CH2Si), 25.4 (CH2CH2CH2), 49.4 (CH2N); NMR 29Si CP-MAS (δ, ppm): −66.9 (T3), −102.1 (Q3), −110.7 (Q4); BET S = 9,2 m2/g.
4.1.8 Hybrid G7b
White powder; IR (KBr, cm−1): νSi–O = 1054; νC–H = 2948; NMR 29Si CP-MAS (δ, ppm): −68.4 (T3), −101.1 (Q3), −109.6 (Q4); BET S = 98 m2/g; Vp = 0.25 cm3/g, Øp = 103 Å.
1 Unpublished work under progress.