1 Introduction
The high-level liquid waste (HLLW) produced in the PUREX reprocessing process of the spent nuclear fuel contains long-lived minor actinides, such as americium and curium. At present, the most studied concept for the management of HLLW is to vitrify it in the glass matrix followed by disposal in deep geological repositories. Since the half-life of the minor actinides concerned ranges between a few hundred and millions of years, the surveillance of this high active waste for such a long period of time is difficult. An alternative or a complementary concept is the partitioning and transmutation option, which envisages the complete removal of minor actinides from HLLW and their consequent transmuting or burning them to short-lived fission products (about 1000 years of storage) in suitable transmutation device [1].
Several extraction processes have been developed worldwide for the recovery of Am and Cm from HLLW [2,3], i.e. TRUEX [3], TRPO [3], DIAMEX [4], and TODGA [5]. In these processes, minors An(III) and Ln(III) are co-extracted. An(III)/Ln(III) group separation has great importance because Ln, due to its high crosssection of neutron absorption, adversely affects the efficiency of the transmutation of An. This separation is one of the most challenging issues because of the similarity of their chemical properties. Problems are particularly posed by the identical oxidation states and the similar ionic radii of the metal ions [6]. Coordination chemists have been focusing on the development of complexing ligands with soft donor atoms like N and S to exploit the increased covalent interaction with f-orbitals of actinide ions besides lanthanide ions which provides the opportunity for the successful An/Ln separation [7–9]. However, in some cases these ligands seem to be sensitive to hydrolysis and/or radiolysis [8].
Besides the synthesis of new soft donor extractants, separation can be improved by associating the nanofiltration process with a selective complexation step of the targeted ions. Polyaminocarboxylic acids are well-known reagents to separate An(III) from Ln(III) because their complex formation constants with An(III) are higher than those with light Ln(III). The addition of water-soluble chelating agents to the aqueous phase to selectively strip An(III) from the organic phase was first investigated in the late 1960s [10]. DTPA has been successfully used as water-soluble chelating agent in the TALSPEAK [6,10], SETFICS [11] and TOGDA [12,13].
In the present paper, nanofiltration assisted by complexation (NAC) has been evaluated for the separation between Am and Eu. NF represents a good alternative for metal ion processes because it generates only wastewater without phase shift or use of solvent. Nanofiltration is a pressure-driven membrane separation process situated between reverse osmosis and ultrafiltration in its separation characteristics. Size effect, membrane solute interaction and electrostatic charge are the major parameters which govern the separation process [14–16]. For charged compounds like metal ions and metal complexes, both steric hindrance and electrostatic interactions are responsible for separation. Membranes in contact with an aqueous solution acquire an electric charge by dissociation of surface functional groups, adsorption of ions and ionised solutes from the solution. The electrostatic interactions can take place on the surface of the membrane or through the pore [17]. Therefore, the NF membrane can reject charged solutes of much smaller size than the dimensions of the pores. This Donnan exclusion mechanism can be particularly used to remove ions from wastewater and to separate ions according to their ionic valences [15]. Applications of NF can be found useful for the removal of organic and inorganic micropollutants from both surface and ground water [18] or for the concentration of metal traces [19]. Additionally, solution chemistry and in particular pH and water-soluble complexing agent, have a significant effect on the membrane charge and on speciation of the solute. This will drastically change the membrane permeability of the solutes in solution [17,20–23]. NAC has been previously studied by our group for the selective removal of different metal ions from radioactive wastes or simulated radioactive wastes [24–27]. The main objective of the present study is to investigate the separation of Am traces from Eu ([Eu]/[Am] = 3720) with an organic spiral-wound membrane (Desal GH, Osmonics) as a function of the pH and the ratio [DTPA]/([Am] + [Eu]).
2 Separation using nanofiltration
2.1 Determination of rejection and selectivity
The rejection Ri (%) of a substance i was calculated according to Eq. (1) where CPi is the concentration of i in the permeate and CFi is the concentration of i in the feed.
(1) |
The transmission Ti (%) of a solute i, is the ability of solute i to pass through the membrane, which can be expressed according to Eq. (2).
(2) |
The membrane selectivity MSi/j of two solutes i and j can be expressed by the ratio of their transmission and thus may be expressed as the function of the concentrations of i and j in the permeate and in the feed according to Eq. (3).
(3) |
It is interesting to note that the membrane selectivity is comparable to the separation factor used in the liquid–liquid extraction. The distribution ratio in liquid–liquid extraction is estimated according to Eq. (4).
(4) |
The separation factor used in liquid–liquid extraction is defined as the ratio of the distribution ration according to Eq. (5)
(5) |
By analogy with the nanofiltration experiments, the concentration of i in the aqueous phase, Caqi can be considered as the concentration of i in the feed CFi and the concentration of i in the organic phase, Corgi can be considered as the concentration of i in the permeate CPi. Thus, the separation factor estimated using Eq. (5) is equivalent to the membrane selectivity estimated according to Eq. (3) 241Am and 152Eu concentrations were determined by gamma spectrometry using an HPGe detector (Eurisys Mesures).
2.2 Filtration system
To study the Am/Eu separation by NAC, a filtration system was designed and implanted in a glove box in the Atalante facility of CEA/Marcoule in collaboration with the local team of safety. A schematic flow diagram and a picture of the system are shown in Fig. 1.

Schematic flow diagram of the lab-scale membrane system (1: feed tank; 2: pump; 3: cell body and membrane; 4: feed pressure gauge; 5: feed flow control valve; F: flow meter; pH: pH meter.
The commercial Desal GH membrane (Osmonics, USA) selected for this study was a spiral-wound membrane. The active membrane area is 0.25 m2 and the nominal molecular weight cut-off (MWCO) is estimated at 2500 Da using polyethylene glycol (PEG) [28]. During all experiments, the membrane is used under steady-state operating parameters: tangential velocity feed flow of 0.43 m s−1 with an applied pressure of 1.0 bar which corresponded to a permeate flux of 0.80 μm s−1. The feed is maintained at constant composition by totally recycling the permeate and the feed. In this study isotopic dilution method was used to investigate the rejection of Eu. This method therefore includes the following assumptions: (i) the 152Eu spike is in chemical equilibrium with the Eu carrier; (ii) Eu and 152Eu have the same interaction with all the components of the system.
2.3 Influence of pH
The permeability of NF membranes is influenced by the speciation in solution. The solute rejection can be explained both by the sieving effect (i.e. discrimination according to the size of membrane pores and species to be retained) and the electrostatic charge effect rising from electrostatic interaction between charged species of the filtered solution and membrane material. Membrane Desal GH is characterized by a high permeability of free metal ions like Gd3+ and a low permeability of DTPA complexed ions like (DTPA–Gd)2− [22]. The rejection of equimolar mixture of Eu(NO3)3 and DTPA containing 241Am traces was studied as the function of pH (Fig. 2).
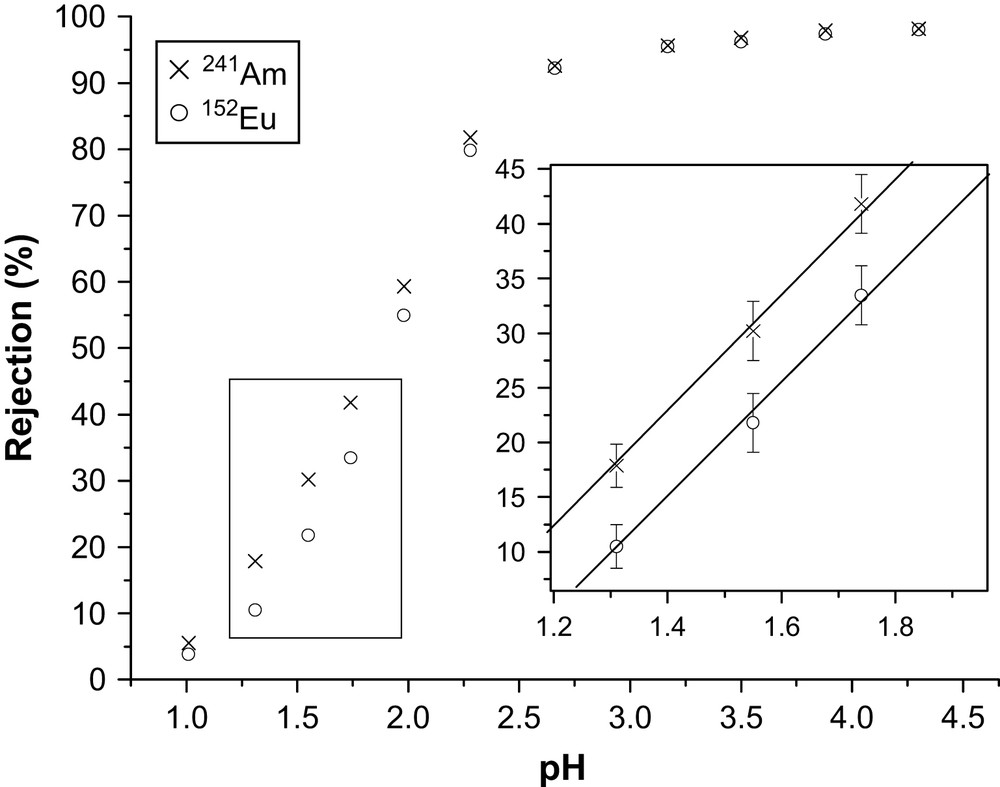
Experimental 241Am and 152Eu nitrate rejection as a function of pH: [Eu(NO3)3] = 2.5 mM L−1, [241Am] = 6.6 × 10−4 mM L−1 (20 MBq L−1); [152Eu] = 1.2 × 10−6 mM L−1 (1 MBq L−1), DTPA/∑(Am + Eu) = 1.
As expected by our previous work on the Gd/DTPA system [22], the observed 241Am and Eu rejection is increasing with increasing pH. Furthermore, at low pH (<2.5) the rejection of 241Am is higher than the rejection of Eu even with a 3700-fold excess of Eu. At pH 1.7, the rejection of 241Am and Eu is 41% and 33%, respectively (Fig. 2). At low pH, the proton competition limits the metal ions' complexation by DTPA and the difference observed can be explained by the chemical speciation in solution. The membrane selectivity is thus determined by the difference of the stability constants of the 1:1 complexes of Am and Eu with DTPA, i.e. log KAm/DTPA = 23.10 and log KEu/DTPA = 22.39 [29]. At pH greater than 2.5, the proton competition is limited and both 241Am and Eu are complexed. Under this condition the rejection of both the metal ions reaches a maximum, i.e. 98%, and no difference between 241Am and Eu rejection was noticed.
2.4 Influence of DTPA concentration
In Fig. 2, at pH 1.7 we can see that the rejection of both metal ions is low (<40%) and a difference of 8% between 241Am and Eu rejection was noticed with a DTPA/∑(Am + Eu) = 1. A membrane selectivity (or separation factor) of 1.2 can thus be calculated according to Eq. (3) under those conditions. Thus, the influence of the DTPA concentration on metal ion rejection at this particular pH was studied (Fig. 3).
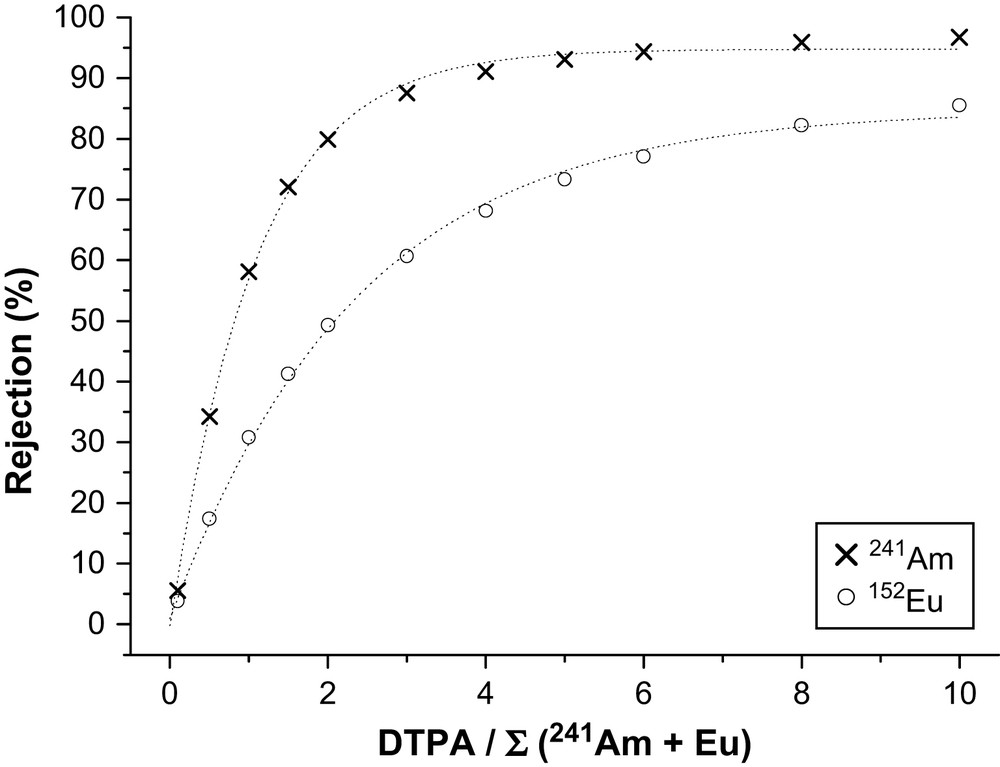
Experimental 241Am and 152Eu nitrate rejection as a function of the ratio DTPA/∑(241Am + Eu) at pH = 1.7; [Eu(NO3)3] = 2.5 mM L−1; [241Am] = 6.6 × 10−4 mM L−1 (20 MBq L−1); [152Eu] = 1.2 × 10−6 mM L−1 (1 MBq L−1).
At pH 1.7 without DTPA, the rejection of both metal ions is less than 6%. When increasing the DTPA concentration in the feed at constant pH, the rejection of 241Am and Eu is increasing. As expected from the stability constants the increase of the rejection of 241Am is superior to the increase of the rejection of Eu. Thus, DTPA selectively decreased the permeability of 241Am through the membrane. The membrane selectivity (or separation factor) calculated according to Eq. (3) is superior to 3.5 using the following experimental conditions: pH 1.7 and [DTPA] = 10 mM L−1 or a DTPA/∑(Am + Eu) ratio of 4. Such separation factors obtained with initial Eu/Am ratio of 3700 are comparable to the results obtained using liquid–liquid experiments with DTPA as masking agent but with initial Ln/Am ratio of 100 [12].
In Fig. 4, the permeation flux of the membrane Desal GH is plotted as a function of the DTPA/∑(Am + Eu) ratio at pH 1.7. No variation of the flux was noticed until a DTPA/∑(Am + Eu) ratio of 5 was reached. When further increasing the concentration of DTPA, a decrease of the permeation flux was observed. An increase of DTPA concentration from 15 mM L−1 to 20 mM L−1 (i.e. an increase of DTPA/∑(Am + Eu) ratio from 6 to 8) induced a decrease in permeation flux of 17%. The flux decline can be attributed to the fouling of the membrane. This process is due to the deposition of solutes on membrane external surface, at its pore opening or within its pores. Fouling is irreversible and the flux can only be recovered by chemical cleaning. Such interactions are complex and are, to date, not well understood [30]. At pH 1.7, it can be suggested that adsorption between the positively charge membrane [31] and the negatively charged DTPA complex, i.e.[DTPA–M]2 [22] is the main contributor to the flux decline. In our case, membrane fouling does not modify membrane characteristics and the rejection was not modified with concentration of DTPA up to 25 mM L−1.

Effect of the ratio DTPA/∑(241Am + Eu) at pH = 1.7 on permeation flux; [Eu(NO3)3] = 2.5 mM L−1; [241Am] = 6.6 × 10−4 mM L−1 (20 MBq L−1); [152Eu] = 1.2 × 10−6 mM L−1 (1 MBq L−1).
2.5 Radiolytic behaviour of membrane Desal G10
A JEOL 5600 LV scanning electron microscope was employed to analyse the macroscopic structure of the membrane (Fig. 5). The Desal GH membrane is composed of three layers: ultrathin active layer, microporous support and support characteristic of composite reverse osmosis and nanofiltration membranes. The active layer of Desal GH (1 μm) provides the properties as to its semipermeabilities (solvent flux and solute rejection).

Structure of the Desal GH membrane (top: ultrathin active layer; middle: microporous support; bottom: support).
The membrane was then irradiated in air using 137Cs gamma source with a dose rate of 2.5 kGy h−1 in a gamma irradiator of CIS Bio international (IBL 637). Fig. 6 exhibits the ATR-FTIR spectra (Attenuated Total Reflexion-Fourier Transform Infrared) of the top layer of the Desal GH membrane as a function of the integrated doses. However, the active layer is too thin and IR light permeates the ultrathin top layer and also detected the microporous sulfone support. Almost all of the peaks were those of the microporous sulfone support except the small-broad amide (1650 cm−1). The peaks characteristic of polysulfone were at 1321 and 1145 cm−1 (sulfone), 1233 and 1012 cm−1 (ether), 1583, 1485 and 830 cm−1 (aromatic).

ATR-FTIR spectra using Bruker Equinox 55 with a 45° diamond top plate crystal of the Desal GH membrane (normalized to the 1485 cm−1 peaks) as a function of the integrated dose (0 kGy, 50 kGy, 100 kGy, and 340 kGy).
For integrated dose up to 340 kGy, the visual aspect of the Desal GH membrane (colour, mechanic resistance) does not change. The ATR-FTIR spectra of the membrane only show little change at 923, 1043, 1660 and 1719 cm−1 (Fig. 6). The increased intensity of the peak at 1650 and 1715 cm−1 could be explained by oxidation of the polyamide active layer. However, these changes are limited for this rather high integrated dose (340 kGy) which is representative of average integrated dose of a solvent used in reprocessing process of nuclear fuels (MOX + UOX2 at UP2-800) during one year. A more detailed study is in due course to know if the hydrodynamic properties of the membrane in terms of rejection and permeation flux are modified by irradiation.
3 Conclusion
The results presented here further demonstrate the potential of nanofiltration/complexation in chemical industrial processes and particularly for traces removal and ion' separation. An effective Am/Eu separation was achieved using nanofiltration assisted by complexation. A separation factor superior to 3.5 was obtained at pH 1.7 using DTPA.