1 Maintaining and acquiring competence in separation chemistry
For the future use of fission technology in a long-term perspective, it is crucial to maintain and develop competence for the safe and economic operation of the present and future nuclear power plants, including waste management and decommissioning. Advanced reactor systems and fuel cycle modeling projects should help attract the bright young professionals needed not only to keep the fission option open, but also to maintain the high level of scientific competence for development and safety of new concepts for energy production.
Market forces work to reduce industry support to long-term R&D at universities and other research institutions serving as a training and recruitment base. Today, the priorities of the scientific community regarding basic research lie elsewhere than in nuclear sciences. Taken together, these circumstances create a significantly different situation from three to four decades ago when much of the present competence base was in fact generated. Also, many of the highly competent engineers and scientists, who helped create the present nuclear industry and its regulatory structure, are approaching retirement age. These competence issues need to be addressed at national level and the “Institut de chimie séparative” is one element of the strategy to be deployed for acquiring knowledge and for building expertise.
This will be done by tightening links between the nuclear research centers and the scientific community and giving access to installations, especially Atalante. The present paper deals with illustrations on key issues for modern chemistry, and with the way problems will be tackled, leading to the creation of seven research teams planned to operate at the Institute of Separation Chemistry.
Any technological problem solving or improvement process requires a thorough understanding of the basic phenomena. Typically an evolution from macroscopic to meso- and microscopic investigation is required and a coupling between the different disciplines and scales of research within one institute is of vital importance. A good knowledge of fundamental physical, chemical and materials science data on actinides and actinide-containing products, i.e. nuclear fuels and nuclear waste, is the basis for addressing nuclear issues at ICS. More knowledge is needed in chemistry at the “nano” scale, concerning the chemical evolution in complex fluids (alias fluids containing “nanoreactors” and materials with large specific surface, alias “nanomaterials”) and the processes associated, such as dissolution, separation and cohesive aggregation.
Acquiring knowledge on actinide behavior is of interest for the whole scientific community. For instance, the recent identification and characterization of the superconductivity of PuCoGa5 can be taken as a revolution, challenging new progresses, since none of the currently accepted descriptions of the 5f orbitals can be used to model these experimental findings. This means that the study of oxides containing actinides may open new routes in the understanding of superconductivity. Here as in other aspects of physical chemistry, the 5f electrons cannot be described by an extrapolation of theories developed for 4f electrons. The study of actinides in this case can result in returns not directly linked to energy production.
The needs for knowledge in the prediction of the evolution of active materials, as well as in separation chemistry have been summarized in the report edited by the French Academy of Sciences [1] in 2000; core of the recommendations is to focus on the mesoscale species of materials separating from the bulk under the action of radioactivity: colloids created under irradiation, ill-defined by extremely present surface activation under radioactivity, coupled reactions by synergy between defects and radicals left along a unique trace due to a radioactive decay.
Extending the questions from the concentrated radioactive material “in ponderal quantity” and the usage materials needed for handling active material in the fuel cycle, another report points to important need in knowledge of material in contact with active fluids. The report by Zaoui and Blanzat points out that these effects can only be modeled efficiently by a multi-scale approach. Interactions between atoms and molecules, including not only the covalent or complexing bond implying an actinide, but also long range interactions not restricted to first neighbour, such as van der Waals have to be considered. This implies that it is crucial to consider the “meso” scale, the scale relevant when a network of cracks and defects has to be modeled with some predictive power [2].
The mesoscale, when active species are linked by weak bonds only, when fluids organize at a supramolecular or colloidal scale, is the modern aspects of chemistry as a nanoscience. As pointed out by Corriu [3], the «nanoscience» alias «colloidal» approach of chemistry is relevant when reactive species are not implied via a simple stoichiometry, but atoms or molecules react as nano-objects, with stoichiometric quantities from ten to millions. Moreover, concepts developed in solid-state chemistry, with crystallographic-plane dependent reactivity, are also not relevant to colloidal chemistry dealing with nano-objects, since «weak interactions» are at work in the chemical transformation of nanoparticles or nanodroplets. Effect of radioactivity is always to produce large amounts of fluid–fluid or fluid–solid or solid–solid interface, typically hundreds of square meter per gram. Therefore, chemistry of radioactive solids or fluids most of the time deals with submicron sizes of materials or droplets: these are nano-objects, the new word for colloids. It is remarkable that the first French Nobel prize in chemistry was obtained by Jean Perrin for investigating colloids and that his son, Francis Perrin, was the leading scientist in the early development of science at the “Commissariat à l'énergie atomique”.
Even nowadays, colloidal aspects are still crucial for the nuclear fuel cycle. For instance, it has been shown less than 10 years ago that efficiency and selectivity of tributylphosphate, the ubiquitous extractant used to separate uranium and plutonium from nitric acid solutions since the Manhattan project, are only because molecules which are being extracted in apolar solvents aggregate in small “water in oil” micelles. This means that each extracted ion is in interaction not only via complexation with one given complexing molecule, but with all molecules in a micellar aggregate. When this aggregate is present in solution, activity of the extractant is highly non-linear and the extraction is largely enhanced [4]. Phase transition and stability of these extracting fluids, such as those used in columns at the La Hague nuclear plant, can only be understood and predicted once it is realized that these micelles, considered as individual nano-objects, are the actors of liquid–liquid phase transitions driven by weak attractions. This “nanoscience” approach, for which each micelle is a nanomachine, allows making bridges and similarities with other micelles, used in detergency or assisted extraction of petrol. The instability recently identified in nuclear fluid extraction is equivalent to “Winsor II–Winsor III” equilibriums identified in the early 1950s in the field of formulation and emulsification by Winsor [5].
These “nanomachines” are nucleated around polar nanometre-sized cores, with specific properties, and the equilibrium between this core and the hydrated ions in aqueous solution controls selectivity (Fig. 1). The situation is reminiscent to the core of water channels, in the proton pump of photosynthetic transmembrane protein systems, or other membrane channels. In these biological systems, selectivity and efficiency, as well as control of separation of species are orders of magnitude higher than in ion extraction-desextraction using complexing molecules. It is not only in electronics or mechanical engineering that there is still ‘‘a large room at the bottom’’, to remind the prediction of Richard Feynman.
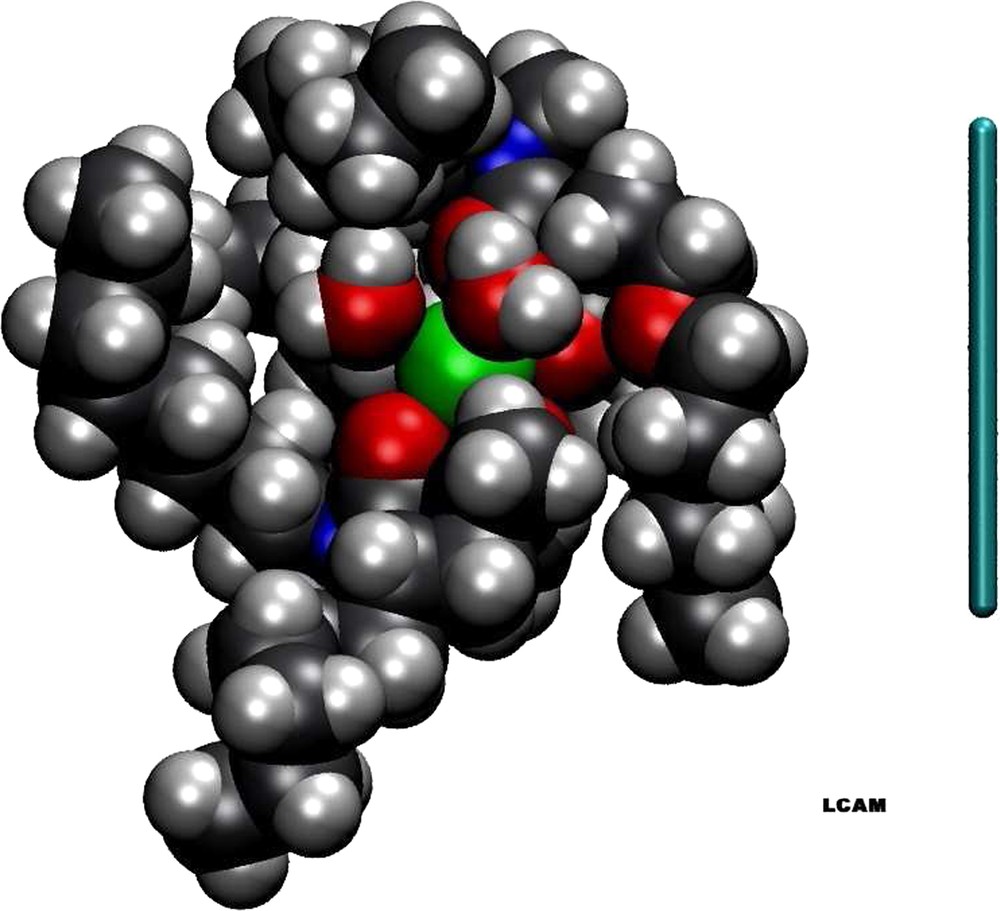
Synergeic localization of complexing molecules around an ion, seen as a reverse micelle formed by four complexing molecules, around the polar core containing the ions to be extracted as well as the co-extracted water molecules. The bar is 1 nm (thanks to Philippe Guilbaud).
2 Selected topics and teams
In relation with these questions, linked to various sub-fields of physical chemistry, three axes of research need to be developed for the needs of a sustainable nuclear energy.
- A – Combination of nanostructure and thermodynamic aspects in the physical chemistry of complex fluids used for extraction, decontamination, precursor solution for nanomaterials, or matrices for interim storage.
- B – Characterization and modeling at the nanometre supramolecular chemistry level, which is the scale relevant to ‘‘colloidal science’’, now more widely designed as «nanoscience»’. Solid--liquid as well as liquid--liquid interfaces have to be characterized and modeled when chemical separation occurs through the interface (simple transfer, corrosion or oxido-reduction).
- C – Modern solid-state chemistry of nanomaterials and hierarchically based materials, including bio-inspired hybrid materials with controlled shape at sub-micronic levels. This includes characterization by structural methods of all scales involved for materials at the preparation stage as well as during the corrosive evolution at high temperature or in extremely “corrosive” situations, enhanced by the presence of radioactivity and high fluxes of ionizing radiation.
More specifically, seven fields need to be urgently addressed.
Soft condensed matter chemistry of complex fluids, weak long range forces such as dispersion/van der Waals forces are at work in liquid–liquid extraction, with its application in hydrometallurgy. In order to develop predictive models of the water–solvent equilibrium of ions, the explicit distribution of ions in the first nanometre near the monolayer of extractant present at the water–oil interface needs to be measured and taken into account. By analogy with kinetics of chemical reactions, it is clear that the transient excited limiting step is the ion adsorption on the macroscopic monolayer. These ions present in the “excited transient state” are in kinetic equilibrium with ions in water as well as in reverse micelles. The extraction yield depends on the free energy difference between water and reverse micelles, while the kinetics of transfer depends on the activation energy, which is the difference of the energy between the aqueous bulk and the excited state of ions. The interaction between the transient fluctuations reminiscent of liquid crystals and the macroscopic water–solvent interface must be characterized and their microstructure as well as dynamics needs to be elucidated. Modeling kinetics at the liquid-liquid interface is crucial to predict extraction yields for a given chemical engineering strategy including continuous emulsification of microemulsions. This needs to be understood in terms of a balance three possible states of the ions: hydrated, adsorbed at the macroscopic interface and embedded in small micelles. Similar situation occurs in the corrosion process of surface layer; it has to be quenched for waste-conditioning material or enhanced for efficient solubilisation of solids: development of predictive models of reactants' distributions (mainly ions and charged species) in the last nanometre and in the diffuse cloud of reactive species present at the interface is needed. These models should provide a description beyond the DLVO theory, i.e. they should take into account the Hofmeister effect, surface charge regulation, dispersion forces and molecular protrusion [6]. This will be the central paradigm of the interfacial chemistry under reactive conditions (team 3).
Synergeic effects often attributed to self-assembly at supramolecular scale as well as colloidal scale require better understanding of the extractant self-assembly, at the origin of extracting power. Moreover, the “solvent” used by chemical engineers often contains solvo-surfactants, hydrotropes which induce long-range order in the solvent, as well as co-surfactants, with deep implication in the cohesion density and liquid–liquid surface tension. Most effective and selective processes able to perform reversible selective adsorption/desorption of specific ions in a mixture involve synergic effects in the interaction between recognized “target” ions and a self-assembled nano-object, contained in either a droplet or a solid nanoparticle; therefore peptides containing extractant groups as well as surfactants with grafted complexing groups will be used as model systems. The methods of combinatory chemistry using self-assembled peptides or modified peptides will be used to compare self-assembly and selective ion recognition capacity. Modeling at supramolecular level to develop predictive models required for process development will be an important task. These topics will constitute the central paradigm of the team dealing with ion separation using bio-inspired supramolecular self-assembled colloids (team 2) working in close cooperation with the team devoted to ions at interfaces.
Using chemistry induced mechanically by sound requires combining physical chemistry of liquid surfaces (as in teams 2 and 3 just described) and sonochemistry and could potentially cross-fertilize recent advances in both fields – using the physical chemistry of colloids such as controlling intermolecular forces by the presence of salt, solvo-surfactants or charged surface active crystalline nanoparticles by possibilities offered by sonochemistry. We will characterize and model phase transitions such as nucleation and coalescence of bubbles from gases dissolved in liquids as well as chemical reactivity under high temperature, high pressures and ultrasonic speed of reactant molecules during the implosion of the micron-sized bubbles coming from gas dissolved in the liquid.
Surface of bubbles appearing in liquids under ultrasonic excitation are by definition absolutely clean, and the density of ions and solutes at the liquid–vapor interface can be controlled and finely tuned by formulation of the fluid (chaotropic versus cosmotropic salts, hydrotropes and surface active partially hydrophobic colloids). Chemical study requires time-resolved (nano- to microsecond range) spectrophotometry of the light emitted, as well as the electrical field radiated by ions associated to the interface.
The initial direction of research is to measure and develop predictive models of the light emitted by the bubble during implosion, depending on the solutes present, and use this knowledge to develop new separative physical processes such as sound-induced flotation or new “green chemistry” processes, such as selective destruction, oxidation of toxic species, or peptization of flocculates containing heavy ions or even actinides.
Sonochemistry, i.e. chemical reactions triggered by thermal and pressure ultra-short transient peaks of temperature, is potentially the source of a vast emerging “green chemistry” technology based on nanometric to micrometric bubbles which can be generated via ultrasound. Under hydrostatic oscillating pressure, dissolved gas nucleates in the form of bubbles. The coalescence of bubbles is controlled by salt-specific effects, surface dilation modulus and by adsorption of colloidal semi-hydrophobic species. However, the relative importance of these three mechanisms is not known. The “test tube” of the sonochemist is a sub-micronic bubble and the reagents are the molecules present at the interface, between the compressed vapor bubbles till the implosion of the bubble [7]. No predictive model exists yet about the most direct manifestation of sonochemistry: sonoluminescence produced during bubble implosion, and the effect of solutes present in the liquid submitted to ultrasonic excitation.
Reacting species are the liquid vapor, the gases initially dissolved in the fluid and also all species specifically adsorbed at the interface such as salts including a structure-breaking (“chaotropic”) ion or hydrotropes able to rearrange within milliseconds at the liquid–vapor interface. During formation of the bubble, surface active materials as well as any partially hydrophobic colloids adsorb on the bubble and concentrate reagents in the bubbles. The timescales involved in temperature and pressure spikes are comparable with self-assembled structures in soft matter and complex fluids. Also, bubbles produced via ultrasound can nucleate easier in the presence of rough powders, which are degraded in corrosive conditions at a much larger rate in the presence of cavitations induced by vaporization/degassing provoked by ultrasound.
Bubbles induced by ultrasounds may be stabilized by surface active molecules and polymers, and hence may be the core of a whole technology transposing the flotation process – the biggest colloidal process by tonnage on mankind – from macroscopic bubbles to microscopic bubbles produced by ultrasound in water solutions saturated by gas. Since the bubbles produced by ultrasound are smaller than those used in standard technology via a “frit”, their Laplace pressure is much larger. Thus more possibilities are offered by ultra-sound produced bubbles flotation than classically, as obtained via blowing gas into a solution through a porous solid. New separation processes could come from the control of sonochemical processes, treated by analogy with detonics of fast reactions (Fig. 2).
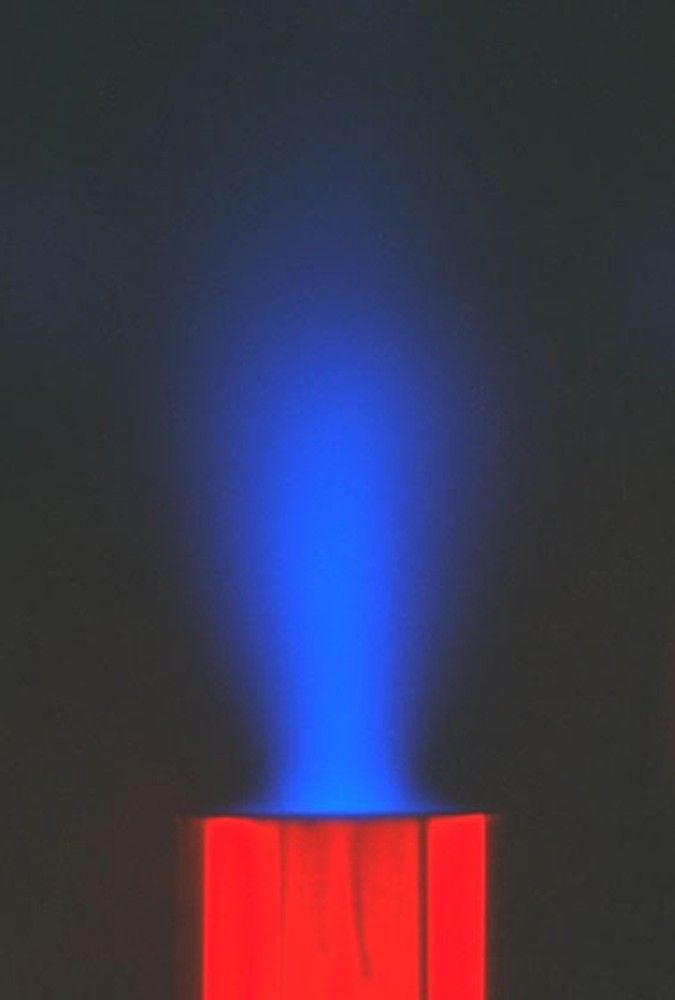
Sonoluminescence produced in a liquid under ultrasound excitation.
The micron-sized bubble resulting from nucleation of initially molecularly dissolved gas and vapor is indeed an ultra-high temperature and pressure transient chemical reactor, with high power for disrupting and finally new ways of solubilizing cohesive nanohybrids, as required by the fuel cycle. The reactants in interaction are molecules mainly located at the solid–liquid interface. This type of chemistry minimizes secondary waste for different reasons, including the absence of a permanent “container” where high T–P takes place. Progresses in the comprehension of the simplest cases (sonoluminescence or sonication of dispersed solids) require conjunction of competence in physical chemistry of multiphase systems, acoustics in droplets and also chemistry of interfaces. This furtive chemistry, studying sonochemical processes in complex fluids will be the scientific domain of expertise of one team at ICSM (team 4).
Two mainstreams for waste materials are well understood, as a result of intense fundamental research since 20 years: the family of borosilicate glasses including engineered modifiers with its star “R7T7” and microcrystalline ceramic material with a large amount of intergranular amorphous material known as SYNROC inspired by geochemical observations. Whatever be the microstructure, no solid material can be engineered in order to reduce to undetectable values the release of complex fractal-like colloids created by energy release in the material. The key property of glass is the local reformation of the glassy network. These inorganic complex “polymers” are released at the macroscopic interface of the confinement material, and are one vehicle for transport of cations. However, the complex fractal colloids could be considered as the precursor of a secondary material, for example by ion exchange within an ionic solid. The mechanical or leaching performance of the ionic solid could be improved after solid-state reaction with radiolytically produced species (Fig. 3).

An “active” molecule adsorbed at an interface, compared to the counter-ion cloud (thanks to Sylvain Prévot).
Using modern progresses of hybrid multi-scale nanomaterials, there is a large class of possibilities of multi-scale nanomaterials. For instance, colloidal silica could be engineered for initial storage of active species, but these could be embedded at the micron-scale in an ionic solid. The latter would be reactive towards the species released at the interface of this hybrid nanomaterial in order to form a slowly forming “secondary phase” with better usage properties than the initial material. Application of this strategy is not limited to the nuclear industry. For example developing paints that self-heal slowly under the influence of atmospheric carbon dioxide would also be possible by concentrating in the same institute competence in synthesis, characterization of evolution and modeling of this type of nanomaterials. The knowledge about nanomaterials, their synthesis, the chemical reactivity and modeling of long-term usage properties will be the driving force for the team Self-repairing of hybrid nanomaterials with hierarchical structure (team 5). Of course, if one of the nanomaterials designed is of interest for specific storage application after separation of species, this team will be working with teams already active in Marcoule outside the Institute of Separation Chemistry involved in development of these materials and that involved in program-oriented projects.
Long-term usage with a high level of confidence and known safety margins of materials at high temperature, in the presence of corrosive fluids such as molten salts or concentrated ionic solutions requires also better experimental knowledge of the “interface”. Characteristics of the latter to be determined are thickness, own internal structure as well as speciation of atoms involved. Each of the atoms or molecules near the interface under stress (high temperature, oxidative stress) can be characterized by a coefficient of diffusion. From there, they are two types of “extreme cases”. Corrosion of the interface is dominated by the “disappearance” of atoms or molecules near the interface and hence displacement of the interface towards the bulk material, with instability of shapes associated to defects or local re-crystallisation. The opposite extreme is the case where the diffusion of species is faster than the speed of interface displacement. The first case is the case of ceramics in mild conditions. The second case is typical for fluids in boiling conditions. Complex gels or nanomaterials close from the melting transition lie probably in the intermediate case, when neither diffusion of atoms nor the speed of dissolution can be neglected. Acquisition of experimental data on selected model systems requires an effort in measurement of solid-state diffusion, characterization of interface and evaluation of surface reactivity in extreme conditions. The selected model systems will be preferably relevant to generation IV of nuclear plants, because fuel will be used at the highest possible “safe” temperature, but could also be chosen as confinement materials under very aggressive chemical stress, and the problems related linked to re-dissolution of materials, since dissolution of materials or uncontrolled solubilisation is the main “enemy” of efficient separation chemistry.
Successful predictive modeling of evolution of a solid–fluid interface will require a subtle dosage between approach involving diffusion of atoms, diffusion of defects as well as evolution of the interface defined by a distribution of curvatures. Evolution of surface structure in real cases and the kinetics associated have to be described along several mechanisms, since the corresponding morphology of the surface is present in several forms, ranging from simple uniform ablation to formation of dendrite cracks or even autogenic build-up of fractal structures. Associating surface and bulk characterization methods on model systems selected with our colleagues from applied research with sufficient precision to allow successful predictive modeling is the initial direction of research of the team Interface of materials under rapid evolution (team 6).
Most of the scientific challenges in separation chemistry as well as in sonochemistry described up to this point may involve actinides as frequently as possible; natural uranium and thorium as well as depleted uranium will be handled in the institute within the French regulation. All experiments involving other actinides, for which security and safety requirements need the usage of chemical and physical instrumentations in “zones surveillées” will be done at the adequate installation “Atalante” in cooperation with teams managing this large experimental facility. Actinides will therefore be studied as ions in fluids, up to ions embedded in micelles and liquid crystals. For the teams working on porous solids, interfaces and nanomaterials, actinides will be chemically bound to solids, such as oxides, nitrides or carbides.
The first target here is to improve knowledge about thin layers of actinides, i.e. their reactivity and dissolution from the metallic or oxide form in the presence of oxygen peroxide. This simple way of introducing highly reactive species in a known concentration will be studied in detail. The knowledge of the behavior of actinides in the presence of radical produced by an ionizing particle along a track needs to be largely improved. For example the relative importance of the covalence or the ionicity of the bond between atoms in a solid, as well as in a salt, molten salt or highly concentrated solution is not known. Moreover, it is known qualitatively that metallic or oxide-type layers synthesized through different routes have extremely different behaviors. Two known routes of synthesis of well-defined thin films are either assembling “secondary building units” or small nanoparticles usually containing 1–10 actinide atoms, or using vapor phase deposition process which produces the deposit layer by layer from the gas phase. Therefore, in a joint research program with teams at the ITU-Joint Research Centre, Karlsruhe, dissolution kinetics and morphological evolution will be studied on “thin” layers – thin meaning here 1 nm to 1 μm – in the presence of oxygen peroxide, and later, in the presence of minor actinides so as to study quantitatively the effect of self-irradiation.
These scientific targets will be addressed by the team Chemical and physical properties of massive actinides (team 1). Especially strong scientific links with teams 1 and 2 will lead to common publications about classifying actinide-based anions or cations in the Hofmeister chaotropic/cosmotropic series. Implication of the interaction of actinides with red cell membranes or model membranes made of polyelectrolyte or actin filaments adsorbed on bilayers will be studied in collaboration with the other fundamental research laboratory in Marcoule: the “Service de biologie et de toxicologie nucléaire” and proposals will be submitted to the ACTINET network. Properties related to oxido-reduction, as well as solubility and corrosion will be studied in conjunction with team 6. Interaction with the sonochemists will involve separation by flotation of actinide-based colloids. Nanomaterials prepared by team 5 will of course include actinides, while the experimental methods developed for characterization of surface by team 6 will of course be used by the scientists dealing with actinides.
Finally, there is also a strong need for in situ isotropic imagig in materials containing active species. Looking back at what has been achieved after 20 years of effort in imaging ice cores extracted in polar regions, one realizes that characterization of materials which have been for long period in high radiation environment possibly reveals interstitial hydrogen or other isotopes which could be studied using localized nuclear magnetic resonance. Their 3D cartography using new solid-state imaging techniques would be probably an invaluable information for models of long-term usage – long term meaning here 10–50 years – of materials in an active environment. Even in cases where 3D imaging is not possible, the simple chemical characterization such as via the shift of silicium according to coordinence with oxygen atoms, is already important, if possible in an active environment containing paramagnetic species, and with ‘‘imaging” coming from polarization transfer at a fluid-solid interface. The recent giant leap of possibility offered by ex situ NMR techniques [8], much easier to nuclearise than conventional high field NMR machines limited to low levels of activity (typically less than 1 MBq per sample), will be used by the team devoted to isotopic chemistry and NMR imaging. This team will work very closely with a team in project-oriented research already present in Marcoule.
Availability of large quantities of the appropriate isotopes would allow the studies of solids and liquids by NMR: the labeling of an essential or a toxic element in a sample containing enzymes or active membrane-based biochemical systems would provide useful information on fate and species of a given element in living systems.
This type of applications is possible only by conjunction of two factors: availability of isotopically pure samples, liquid- and solid-state NMR in controlled environment. These goals can therefore only be tackled by association of a production line of pure isotopes in CEA, associated with teams in basic research of materials and fluids, and biochemistry and biology.
As a more ambitious long-term target, it is important to share instrumental and methodological developments of “analytical” instrumentation, either spectroscopic or based on scattering or cartography of local properties, as a generalization of imaging with non-radioactive instrumental developers. This has been done in the field of mass spectrometry. A national effort has been taken in the frame of the GDR “NOMADE” as well as in European networks to start feasibility experiment of solid-state NMR including magic angle spinning of samples containing several percent per mass of actinides. These experimental techniques based on NMR adapted to high levels of activity would be relevant also in applied programs aimed at designing new conditioning of nuclear waste, substrate for incineration of minor actinides as well as for nuclear fuels. All these experiments could be done also at high temperature, if the design of a sample cell can be certified.
There are even more attractive objectives in physical chemistry of isotopically pure materials, that could only be developed within CEA. Considering again the case of PuCoGa5, it appears that the transition temperature towards the superconductive state depends on the isotope used to prepare the sample. If these could be precisely measured, the relevant data would be the most crucial knowledge, as this isotopic effect challenges description of superconductivity. Even more “simpler” effects, such as thermal conductivity, can depend by factors up to 50% on the isotopic composition. Coherent phonon propagation, perturbed by minor isotopes seen as a mechanical defect in the lattice, is dominant in the thermal conductivity measured. This is the case with samples containing yttrium, barium and cobalt. More understanding of the underlying phenomena is needed before evaluation of the improvement of performance such as that of semi-conductor chip using isotopically pure 29Si, making profit of its low calorific capacity, in order to produce chips working in extreme environment, a possible contribution of the isotopic chemistry – both knowledge and controlled in-house production lines – to the electronic industry.