1 Introduction
Subsurface migration of low-soluble radionuclides, e.g. Eu(III), tetravalent actinides and Tc(IV), could be effected by the presence of different colloid particles [1–5]. Colloids are submicron particles having high free surface to mass ratio and could effectively sorb cations from groundwater. At high ionic strengths colloids are coagulated and may precipitate on rocks; however, they are stable in diluted solutions.
Colloids that could be found in aquatic environment are mineral particles, hydrolyzed precipitates of metal ions, high-molecular-weight organic substances, microorganisms, etc. In case contaminants are bound to these colloids, such particles are referred to as “pseudo-colloids” with respect to contaminants. Intrinsic or eigencolloids are another type of colloid particles that tetravalent actinides [6] and Tc(IV) [7] could form. Intrinsic colloids are formed through the oxo- and hydroxo bridge formation between actinide atoms [8,9]. Rothe et al. [10] used laser-induced breakdown detection and extended X-ray absorption fine structure (EXAFS) to detect and probe intrinsic colloids that were present in equilibrium with Pu(OH)4 even at relatively low pH values. The formation of intrinsic colloids is possible in the near-field conditions of the nuclear repository site, where the concentration of actinides could be high.
This paper deals with the examination of Pu and Np redox speciation in colloidal suspension typical for conditions of PA “Mayak”. Earlier the migration of actinides including Pu in far-field conditions of PA “Mayak” was studied by the authors of this paper [5]. Colloids were separated by ultrafiltration and characterized by transmission electron microscopy (TEM) with energy dispersed X-ray microanalysis (EDX) and selected area electron diffraction (SAED). It was established that the main colloidal phase is amorphous hydrous ferric oxide (HFO), which was present as spherical particles with sizes from a few nanometers to about a micrometer. Other identified phases were hematite, clays, calcite, rutile, barite, zeolites, monazite, rancieite, plagioclase and other phases. The nano-secondary ion mass spectrometry was used to study preferential binding of Pu and U onto colloidal matter. Elemental distribution of U, Pu and some major colloid-borne elements (Fe, Mn, Al, Si, Ca) was obtained, which reveals that HFO, MnO2 and hematite were responsible for actinide binding [5]. In this paper we continue our studies of actinide redox speciation in far-field conditions by running laboratory simulants.
2 Experimental
The groundwater sample was collected about 500 m away from Karachay Lake. The values of pH, Eh, conductivity and NO3− were measured on-site by an in-situ geochemical probe. The groundwater collected by an in-situ pump was filtered immediately through 200-nm, 50-nm, and 15-nm nucleopore filters (JINR, Dubna) and through 10-kDa and 3-kDa membranes (Millipore).
The analysis of colloids separated on-site by sequential micro- and ultrafiltration included determination of major elements and actinides, scanning electron microscopy with energy-dispersed X-ray microanalysis (SEM–EDX) and nano-SIMS.
The major elements were determined using ICP-MS after washing the filter with 1:1 HNO3 which avoids dissolution of the filter material but enable dissolution of colloids.
For the SEM–EDX (Hitachi S-3200 N) the 1 × 1 cm pieces of each filter were taken, dried and then mounted with carbon to avoid charging effects. For nano-SIMS (Cameca, nano-SIMS-50), the pieces of filters were taken and ultrasonicated in deionized water for a few minutes to desorb colloids from the filters. The aliquot of suspension with colloidal particles was deposited on silicon chips and air-dried. All analyses were performed using 16 keV O− primary ions, and detecting positive secondary ions. The spatial distribution of major elements (Fe, Mn, Ti, Ca, Al) and actinides was analyzed.
During the sampling separate samples of about 1 L each were collected for further laboratory experiments. In order to prevent changes in pH and Eh values they were sealed hermetically in N2 atmosphere. One of these samples was used to determine colloid concentration and size distribution. To determine the total concentration of colloids the known volume of sample was filtered through pre-weighed ultrafilter (3 kDa). After filtration the ultrafilter was dried in an execator and weighed again. The concentration of colloids (in mg/L) was determined by the mass difference after and before filtration. The colloid size distribution was studied by photon correlation spectroscopy (PCS). The aliquot taken from this sample was also used to determine major cations and anions by ICP-MS, AAS and ion chromatography.
The separate 1-L samples were used for actinide redox speciation. It was shown in the earlier study [5] that actinide concentration was too low for redox speciation by spectroscopic methods, e.g. X-ray absorption near edge structure (XANES) or X-ray photoelectron spectroscopy (XPS). In order to use XANES, 242Pu and 237Np were added to the sample to obtain the total Pu and Np concentrations of about 1 × 10−5 M. Both actinides were added in pentavalent form to avoid precipitation of insoluble Pu(IV) or Np(IV) hydroxides at neutral pH values. On another hand, in near-field conditions of Karachay Lake, high redox potential (Eh ≈ +500 to +550 mV) favors Pu and Np stabilization in higher oxidation states. The presence of Pu(V) was earlier demonstrated by membrane extraction procedure [5]. Preparation of Pu(V) from Pu(IV) involves its oxidation to Pu(VI) and then reduction to Pu(V) as described by Nesmeyanov [13]. Freshly prepared Pu(V) solution was used for the experiment and the absence of other valence states was confirmed spectrophotometrically. The stock solution of 237Np already contains Np in pentavalent state, which was also confirmed by spectrophotometry. Upon the addition of radionuclides to the colloid suspension it was continuously shaken and aliquots of solutions were taken periodically to determine sorption of radionuclides. After the steady-state equilibrium was reached for both Pu and Np, they were separated by ultrafiltration (3 kDa) and dried for further XANES and XPS examination.
XPS was done with an electrostatic spectrometer HP 5950A Hewlett–Packard using monochromatised Al Kα (hν = 1486.6 eV) in a vacuum of 1.3 × 10−7 Pa at room temperature. The resolution measured as full-width at half-maximum (FWHM) of the Au 4f7/2 line on the standard rectangular golden plate was 0.8 eV. The binding energies Eb (eV) were measured relatively to the binding energy of the C 1s electrons from hydrocarbons absorbed on the sample surface upon vacuum drying of the samples and taken equal to 285.0 eV. The FWHM was measured relatively to the width of the C 1s line of hydrocarbons accepted to be equal to 1.3 eV. The error in determination of electron binding energies and the line widths did not exceed 0.1 eV and that of the relative line intensities was less than 10%.
The XANES spectra of the Np–L3 and Pu–L3 edges of the air-dried samples were obtained in fluorescent mode at the EXAFS Station of the Siberian Synchrotron Radiation Center. The storage ring VEPP-3 with electron beam energy of 2 GeV and an average stored current of 80 mA has been used as the source of radiation. The X-ray energy was monitored with a channel cut Si(111) monochromator. For the XANES spectra, the energy step was ∼0.4 eV.
3 Results and discussion
3.1 Brief description of the site
The production association “Mayak” is the nuclear-waste reprocessing plant that is located in the South Urals region of Russia. Since the middle of the 20th century it is responsible for the treatment of spent nuclear fuel as well as Pu and U separation for fabrication of new fuel. For several decades low-level nuclear wastes were discharged to the open pond called Karachay Lake. These waste solutions were NaNO3 brines with salt concentration of up to 150 g/L. According to the earlier geochemical examination of the site, the groundwater in near-field conditions is present as oxic (Eh ≈ +500 mV) slightly acidic (pH ≈ 5.5) solutions with Na+, Ca2+ and Mg2+ as the main cations and NO3−, CO32−, SO42− as the main anions [5,11,12].
The geological conditions of the site are characterized by fractured Silurian and Devonian metavolcanic rocks with andesitic and basaltic compositions [11]. High porosity of rocks favors contaminated groundwater migration from Karachay Lake preferably to the south direction. With distance, the dilution of contaminated waste solutions by groundwater occurs, which results in the decrease of Eh values from highly oxic for near-field conditions to about +50 mV for groundwater collected at a distance of more than 4 km. The pH is increased to about 8 simultaneously. More detailed description of the site and its geochemical conditions was given earlier in several references [11,12] as well as in our previous study [5].
3.2 Groundwater composition
The groundwater sample that was collected for analysis had pH = 5.8 and Eh = 510 mV. The major complexing anions were carbonate, hydroxide and nitrate as presented in Table 1. Oxic Eh values could be responsible for stabilization of actinides, including Pu in higher oxidation states. The concentration of 239Pu was 2.9 × 10−12 M, which is below the solubility limit of Pu(IV) hydroxide, taking Ksp(Pu(OH)4(hyd.,aged)) = −58 ± 1 from Lemire et al. [14]. However, the reported concentration of Pu for Karachay Lake and near-field conditions exceeds this value. In case Pu(IV) is the dominant redox state of this element in near-field conditions, the formation of intrinsic colloids is possible. However, no information is available concerning the possible fate of these colloids upon migration to the far-field and dilution of contaminated groundwater.
The chemical and radionuclide composition of the groundwater taken for this study
Component | Concentration, ppm | Component | Concentration, ppm or Bq/L |
Na | 750 | HCO32−/CO32− | 266 |
K | 9.5 | Cl− | 470 |
Ca | 2300 | SO42− | 220 |
Mg | 180 | PO43− | 0.5 |
Mn | 8.7 | NO3− | 6900 |
Al | 3.2 | ||
Fe | 15.3 | U | 38 |
239Pu | 1.6 | ||
PH | 5.8 | 241Am | 0.29 |
Eh, mVa | +510 | 237Np | 10.4 |
a As measured in situ by geochemical probe vs. standard hydrogen electrode.
The obtained fractionation of actinides with colloids of different sizes is presented in Fig. 1. In the oxic conditions of the collected groundwater sample, U is presented in rather soluble hexavalent form. The fraction of about 90% of U was found in filtrate and the rest 10% was distributed through colloids. In general neptunium distribution was the same as U except that its higher concentration was found in colloidal fraction. In contrast to U and Np that were presumably found in high valence states, plutonium, americium and curium were preferentially bound to nanocolloids. This indirectly indicates that Pu(IV) was the predominant valence state of these element under these conditions despite rather oxic Eh values.
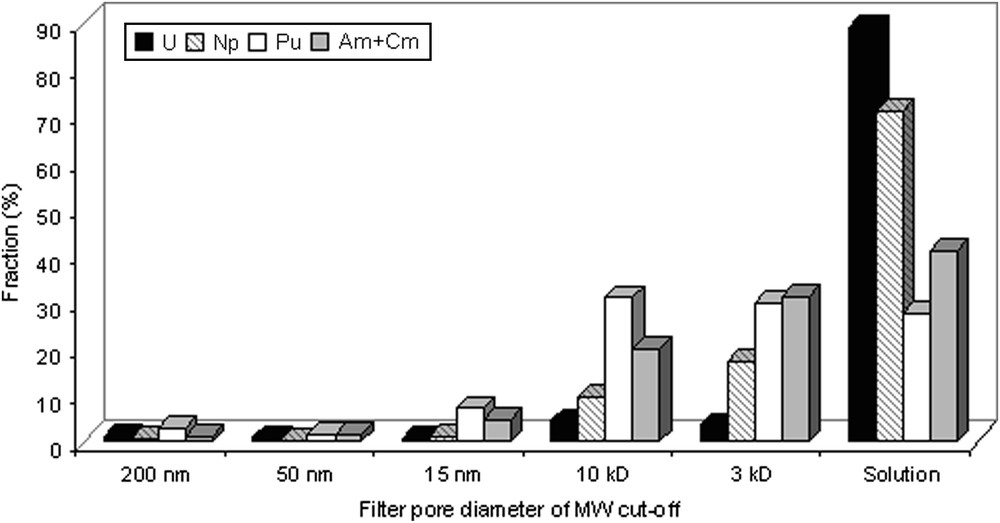
Fractionation of U, Np, Pu and Am together with Cm with colloidal particles of different sizes.
The composition of colloidal matter separated by filtration with different filter pore sizes was studied by SEM–EDX. The typical SEM images are presented in Fig. 2 as well as EDX spectrum. The size, morphology, mineral and elemental compositions of colloids were almost the same as in our previous study of colloids in sample collected about 2.5 km away from Karachay Lake [5]. Most of the colloidal particles were of spherical shape with sizes from a few tens of nanometers to hundreds of nanometers with Fe as the main element. Calcite, clays and silica particles were detected by SEM–EDX but their concentrations were significantly lower than that of spherical Fe-containing particles. The total concentration of colloids was as high as 0.31 g/L as determined by the mass difference of the filter before and after filtration. The colloid size distribution studied by PCS is presented in Fig. 3. The average colloid size was 350 nm, which corresponds to the SEM (see Fig. 2A).
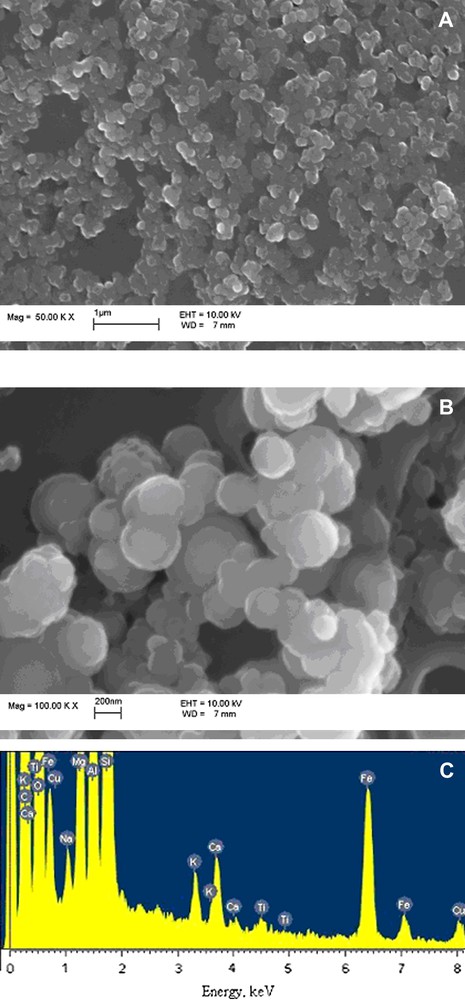
Typical SEM images of colloids isolated from groundwater: (A) general view of filter with 50-nm pores; (B) SEM taken with higher magnification of the same filter; (C) typical EDX taken from colloids.
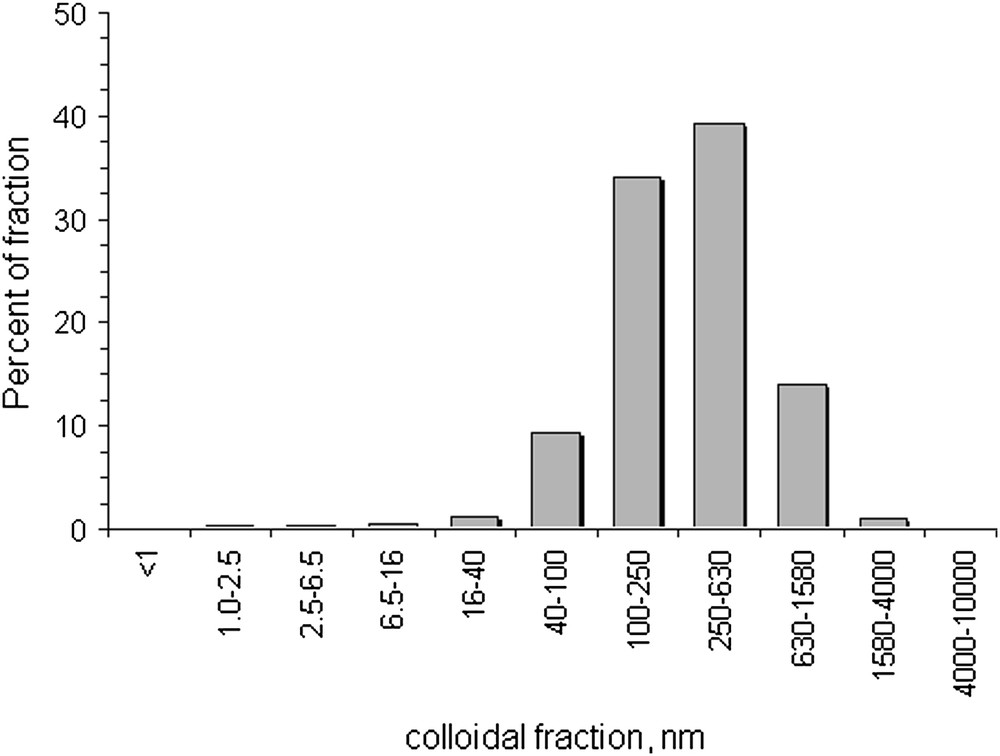
The distribution of colloids according to their size as determined by PCS.
The nano-SIMS was used to determine the preferential sorption of actinides onto different colloidal particles. This method enables to study element distribution with high sensitivity (ppb level) with local resolution of 30 nm. Earlier this method was used to study groundwater colloids from well located about 2 km away from Karachay Lake. In the current study we obtained about 30 elemental maps taken from different filters. The typical elemental map is presented in Fig. 4. The concentration of Np and Pu was too small for nano-SIMS measurements; however, U distribution could be detected despite only a small fraction of these elements is bound to colloids. We assume that sorption of Pu and Np onto different colloids under experimental conditions is the same as for U. This was already demonstrated in our previous paper [5]. As demonstrated in Fig. 4 uranium distribution fits the distribution of Fe. The concentration of Ca is about 1–3% of the Fe concentration. Under groundwater conditions no intrinsic colloids were detected by nano-SIMS.
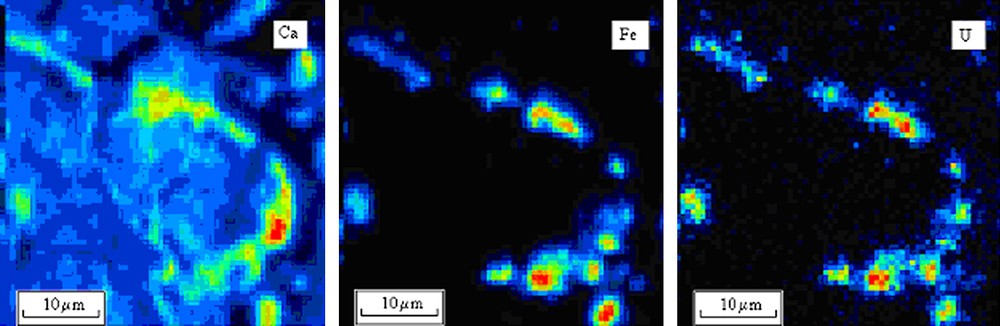
The elemental maps of Ca, Fe and U on colloids separated from groundwater.
3.3 Interaction of Pu(V) and Np(V) with colloidal suspension and its speciation using XPS and XANES
The kinetics of Pu(V) and Np(V) sorption onto colloids separated from groundwater is presented in Fig. 5. Despite both radionuclides were taken in pentavalent form, two major differences in their sorption could be established. First, the sorption of Pu reached the value of 85% in steady-state conditions and only about 60% for Np. Second, the kinetics of Pu sorption was faster than that of Np sorption. These indirectly indicate either different mechanism of Pu and Np sorption or redox state transformations that occur upon interaction with colloid suspension. The valence states of Pu and Np in soluble fraction (not bound to colloids) were determined using solvent extraction technique as described by Choppin et al. [15]. According to these tests both Np and Pu stayed in pentavalent form in solution. To determine redox state of Pu and Np bound to colloids the XPS and XANES were used.
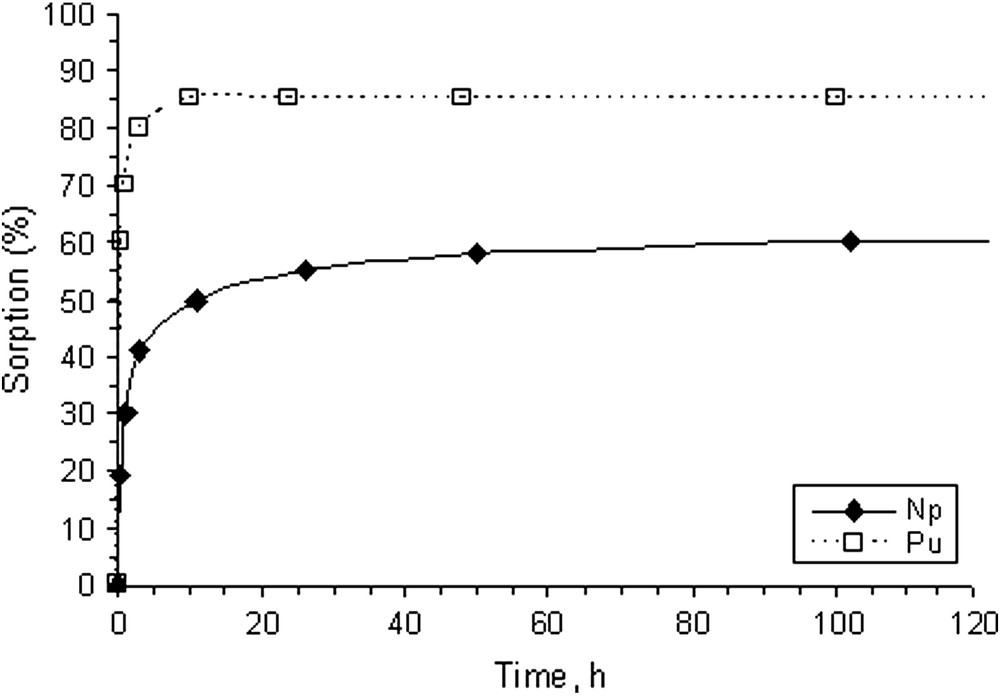
The kinetics of Pu(V) and Np(V) sorption onto colloids isolated from groundwater.
3.4 Actinide redox speciation by XPS and XANES
The spectroscopic methods like XPS and XANES could be used to get undisturbed information on chemical state of actinides on solid substrates including colloids [16,17]. Earlier Teterin et al. successfully used actinide 4f XPS to determine redox state of Pu and Np sorbed onto goethite and hematite colloids under well-defined laboratory conditions. The chemical shift in 4f spectra enables the redox state analysis as well as the presence of shake-up satellites for tetravalent species. The 4f electrons' XPS spectra of Np and Pu bound to colloids separated from groundwater are presented in Fig. 6. According to the presented spectra, Pu(IV) bound to groundwater colloids is in tetravalent state. This is evidenced from shake-up satellites above main peaks whose chemical shift is expected for Pu(OH)4 or PuO2. In contrast to Pu, Np is not changing its valence state and stays as Np(V).
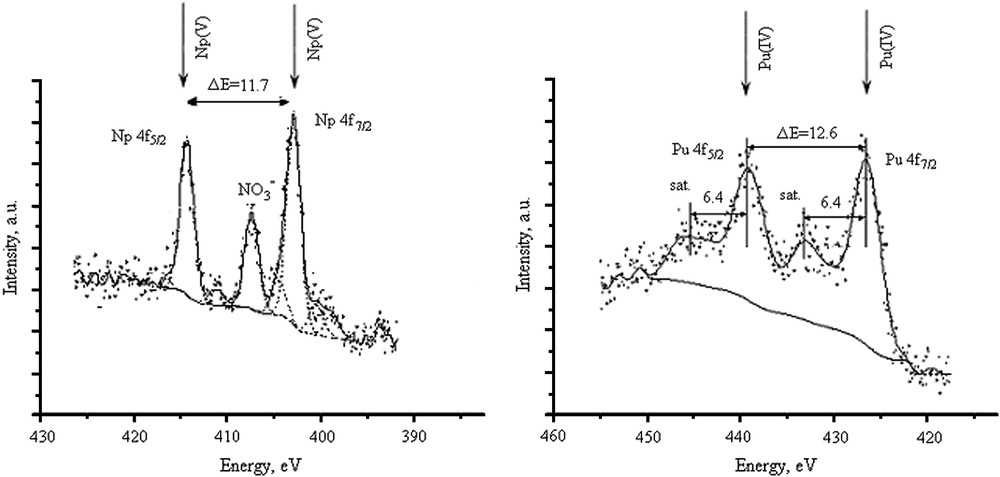
The 4f electrons' XPS spectra of Pu and Np bound to colloids separated from groundwater.
The redox speciation of Pu and Np by XANES gave the same results as by XPS. The obtained L3 XANES spectra of Pu and Np bound to groundwater colloids and reference spectra of Pu(IV) and Pu(V) solutions are presented in Fig. 7. For actinide dioxocations, i.e. An(V)O2+ and An(VI)O22+ the white line (WL) is much weaker than for simple cations, i.e. An(III) and An(IV). In the latter case the WL is followed by deep oscillations while for dioxocations flat transition to EXAFS region is typically observed [18]. The feature of WL of Np XANES spectra is typical for penta- and hexavalent actinides while for Pu it is typical for Pu(IV). Despite the feature of XANES spectra for Pu sample corresponding to the Pu(IV), its normalized intensity is lower than for Pu(IV) aqueous solution. Earlier [19] it was demonstrated that normalized intensity of WL for Pu(IV) in the form of intrinsic colloids is less than that for Pu(IV) aqueous solution. The same observations were made for ZrO2 nano-particles including the effect of particle size [20].
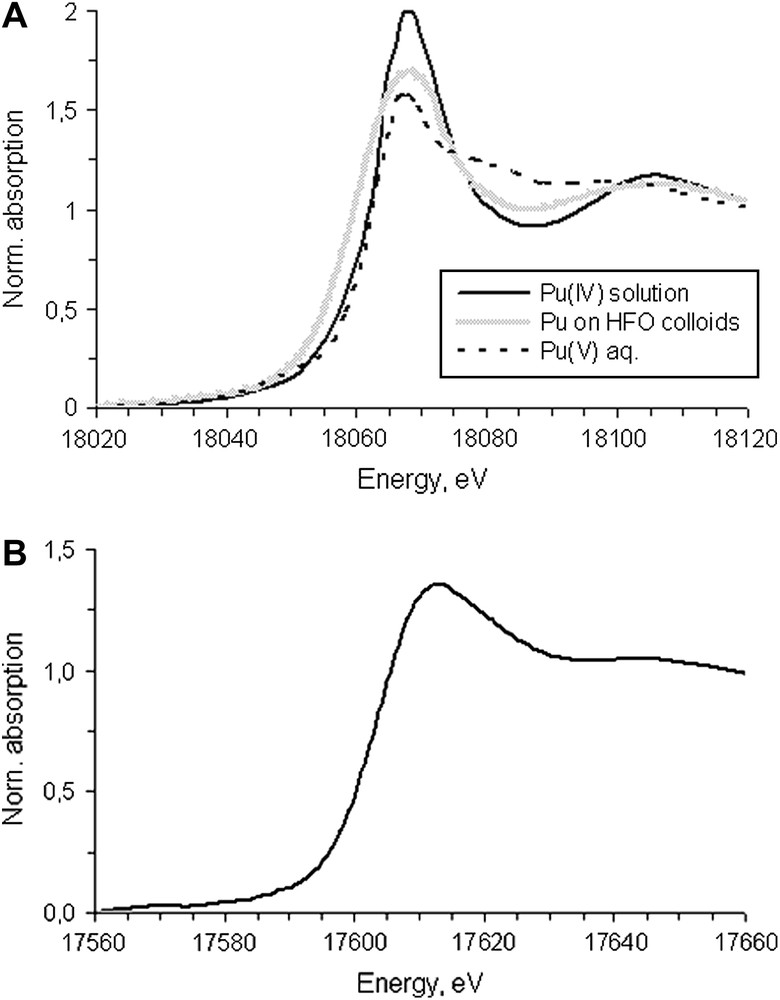
An L3 XANES spectra: (A) Pu(IV) and Pu(V) solution reference spectra and Pu spectra bound to groundwater colloids; (B) Np(V) spectra bound to groundwater colloids.
Following these experimental observations we could conclude that the mechanism of Pu binding to HFO colloids separated from groundwater occur through its reduction to Pu(IV) upon sorption to HFO colloids with subsequent formation of PuO2 × nH2O intrinsic colloids. The reduction of tracer quantities of Pu(V) upon interaction with different Fe(III)-containing colloidal particles was established earlier [21–23]; however, its mechanism remains unclear. Taking into consideration that Pu(IV) has much stronger sorption affinity towards different surfaces than Pu(V), the presence of sorbent will shift the Pu(V)/Pu(IV) equilibrium and increase the stability field of Pu(IV) on pH–Eh diagram. It is also possible that reduction is caused by the increase of local concentration of Pu near the surface (in electric double layer) of colloidal particle. The stability field of Pu(IV) is increased with increase in its total concentration as demonstrated in Fig. 8 for the case of the studied groundwater.
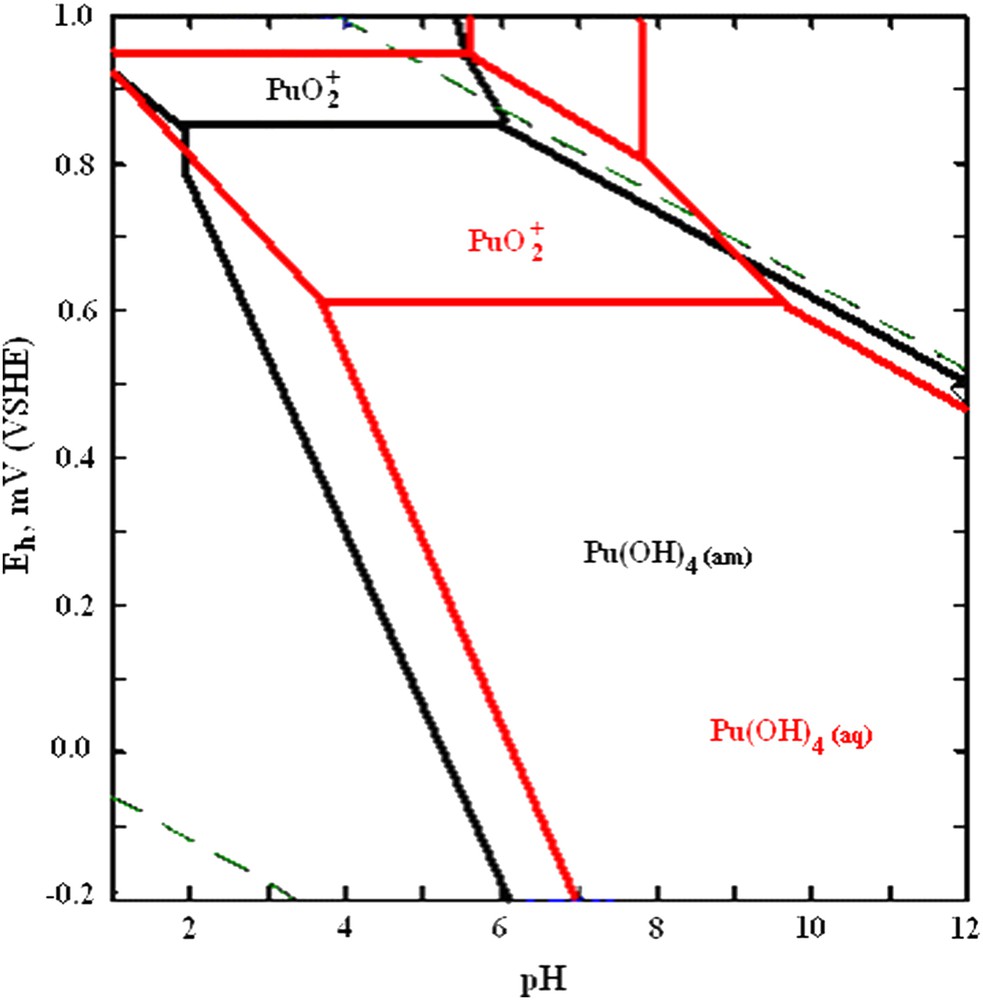
The pH–Eh diagram for Pu. Red – total concentration of Pu is 1.0 × 10−5 M; black – total concentration of Pu is 2.9 × 10−12 M. (For interpretation of the references to colour in this figure legend, the reader is referred to the web version of this article.)
4 Conclusions
The speciation of Pu and Np in groundwater colloid suspension of HFO was studied by a variety of methods. It was established that even at relatively high Eh values Pu(V) is reducted upon interaction with HFO with subsequent formation of intrinsic colloids. This scenario could be realized in the near-field conditions of nuclear waste repository sites. It was established by nano-SIMS that upon migration to the far-field intrinsic colloids are destroyed and pseudo-colloids are formed by Pu(IV) sorption onto HFO.
Acknowledgement
The work was supported by ISTC (Grant 3290).