1 Introduction
NMR has been widely used over the past two decades to investigate the metabolism of living systems from 13C-labelling experiments [1,2]. NMR provides direct information on the carbon position(s) of metabolites that receive the label, from which metabolic pathways can be identified. Moreover, the approach is quantitative and the amount of label incorporated in the various carbon positions of metabolites can be measured. In combination with appropriate mathematical models of metabolism, the NMR data can be exploited for the determination of metabolic fluxes, i.e. the actual rates of biochemical reactions in living systems [3,4].
The amount of label incorporated in a specific carbon position (also termed specific 13C-enrichment) of a metabolite can be measured by 13C NMR, but 1H NMR is usually preferred because of the higher sensitivity of the latter nucleus. Due to the heteronuclear 13C–1H coupling, the NMR signal of protons attached to a 13C nucleus consists of two satellite lines; meanwhile, the signal from 1H attached to a 12C remains central. The amount of 13C incorporated in a protonated carbon can be measured from the intensity of the satellite lines relative to the total intensity of the corresponding 1H resonance. This approach can be conveniently applied to pure compounds, but the narrow spectral width of the 1H nucleus hampers seriously its application to complex mixtures of metabolites such as cell extracts or biomass hydrolysates, which are commonly considered in metabolic studies. To address this problem, different options have been proposed. A first option consists in separating the compounds of interest in order to record resolved spectra. But not all compounds can be always separated properly, and the process can be tedious and time-consuming. Furthermore, this is of no help where the overlapping signals are coming from the same molecule. A second option is the application of quantitative 2D NMR methods where the various signals are separated along the two spectral dimensions [5]. This allows 13C-enrichments to be determined for a large number of metabolites from a single analysis, but the overlapping problems still remain for signals that are in close vicinity. A third option is the application of difference spin-echo methods to discriminate overlapping signals from labelled compounds [6]. The method is based on the selective inversion of the satellite lines, which can be further added or subtracted to the normal spectrum in order to extract the signals from the protons attached to a 12C or a 13C, respectively.
In this paper, we have evaluated the reliability of selective excitation experiments using either DPFGSE [7,8] or DANTE-Z [9,10] modules to provide an accurate measurement of 13C-enrichments. This was achieved by applying the different experiments to a solution of lysine – a compound giving overlapping 1H NMR signals – with known 13C-enrichment. Further, a sequence containing a DANTE-Z module combined to a Zero-Quantum Filtered TOCSY was built-up to generate a method allowing the specific 13C-enrichment to be determined from proton signals that are normally embedded into a crowded region of the 1H NMR spectrum. This method was applied to the determination of the split ratio between the two lysine biosynthetic pathways occurring in the bacterium Corynebacterium glutamicum.
2 Experimental
2.1 Lysine sample preparation
A standard sample of lysine was prepared by mixing 10.9 mg of unlabelled – i.e. 13C at natural abundance – l-lysine-monohydro-chloride (Sigma) to 15.5 mg of [U-13C6]-l-lysine-dihydro-chloride (98% 13C-label, Eurisotop, France). Labile protons were exchanged with deuterium by lyophilizing twice in 3 ml D2O 99.9% (Eurisotop), and the final sample was suspended in 600 μl of 100 mM DCl (Eurisotop). The pH was adjusted to 7 with NaOH before the first lyophilization. Because the sample is prepared from a mixture of [U-13C6]-lysine and unlabelled lysine, all carbon positions of lysine in the final sample have the same specific 13C-enrichment. The 13C-enrichment of the Cɛ carbon measured from the Hɛ signal in the 1D 1H NMR spectrum recorded on the lysine sample was 53.8% (Fig. 1(1)).
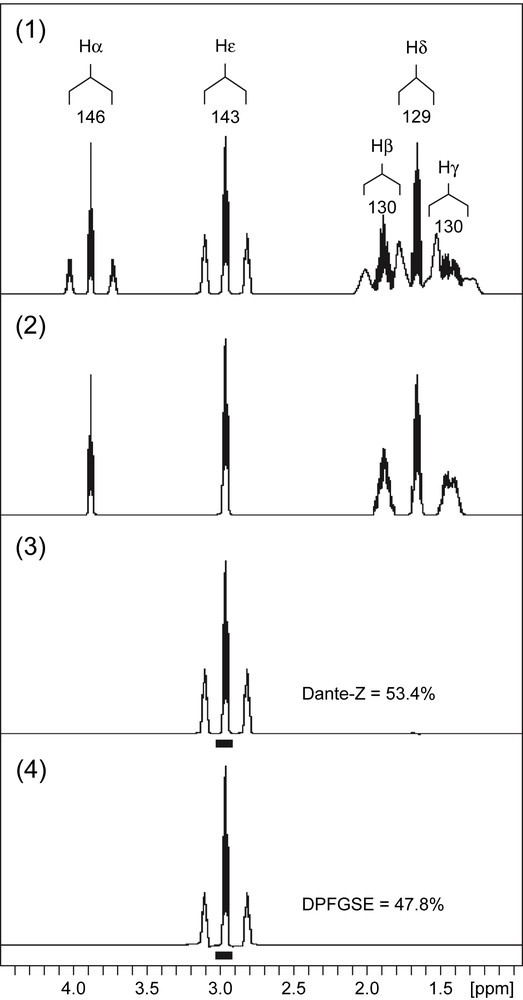
Proton NMR spectra of 13C-labelled lysine. (1) 1D 1H quantitative spectrum. The assignments of the various resonances in the spectrum are provided as annotations above the peaks. Each resonance appears as a central line and two satellite lines separated by the value of the coupling constant – indicated for each resonance below the peak annotation. The spectrum shows that the resonances from the Hβ, Hγ, Hδ protons overlap at the level of the satellite lines, and, therefore, the different contributions cannot be integrated properly. (2) 1D 13C-decoupled 1H spectrum. In this spectrum, all the signals appear as central lines only because the 13C–1H heteronuclear coupling has been suppressed. (3) 1D 13C-decoupled DANTE-Z spectrum. The Hɛ protons were decoupled from 13C nuclei and selectively excited by a DANTE-Z pulse scheme. Because the decoupling fuses all signals into the central lines, the selective excitation can be applied to a narrow 60-Hz bandwidth (black box below the spectrum). The spectra were recorded with no 13C-decoupling during the acquisition period. (4) 1D 13C-decoupled DPFGSE spectrum. Same experiment as in Fig. 1(3), except that DPFGSE pulse scheme was used instead of the DANTE-Z module for selective excitation (black box below the spectrum).
2.2 Corynebacterium glutamicum sample preparation
C. glutamicum was grown on a medium containing [6-13C]-glucose (99% 13C-label) as sole carbon source. At the end of the culture, the supernatant was separated from the cells by centrifugation and dried under nitrogen flux. Labile protons were exchanged with deuterium as described above for the lysine standard sample.
2.3 NMR spectroscopy
All 1D and 2D NMR spectra were obtained on an Avance 500 MHz spectrometer (Bruker) equipped with a 5 mm z-gradient BBI probe. The data were acquired and processed using TOPSPIN® 1.3 software. The temperature was 298 K.
The 1D proton spectra were recorded by using quantitative conditions. To have full signal recovery, the relaxation delay between scans was 30 s and the pulse angle was 30°. The sweep width was 5000 Hz and the acquisition time was 1.6 s. A total of 16 scans were accumulated.
Selective excitations were performed with shaped pulses. The excitation range was 60 Hz except for the γ protons, for which it was 100 Hz because of the larger multiplet width. The 1D 13C-decoupled DANTE-Z sequence consisted of eight consecutive scans of on-resonance Inversion BURP (I-BURP) pulses followed by eight consecutive scans of off-resonance I-BURP pulses [11]. This cycle was repeated four times. A 0.7 G/mm purge gradient was applied after the selective excitation. The 1D 13C-decoupled DPFGSE sequence consisted of two REfocusing BURP (RE-BURP) pulses. The gradient powers were set at 0.7 and 0.3 G/mm, respectively. The decoupling of 13C nuclei during the selective pulses was performed using a GARP4 sequence at a power of 3.8 kHz.
A 13C-decoupled DANTE-Z, Zero-Quantum Filtered TOCSY (DANTE-Z ZQF-TOCSY) sequence (Fig. 2) was generated by inserting a 13C-decoupled DANTE-Z pulse module into the previously described ZQF-TOCSY sequence [12]. The 13C-decoupled DANTE-Z pulse was applied with the same parameters as above. The TOCSY transfer was carried out using a DIPSI-2 mixing sequence applied during 20 ms with 10 kHz field width. At the end of the mixing period, in-plane magnetization was suppressed by a square 2.6 G/mm purge gradient. A Zero-Quantum Filter [13] consisting of a 180° CHIRP adiabatic pulse with 10% smoothing, 20 kHz sweep width, and 30 ms duration at 330 Hz maximal RF power, was applied before acquisition. A square 0.2 G/mm gradient pulse was applied during the adiabatic pulse.
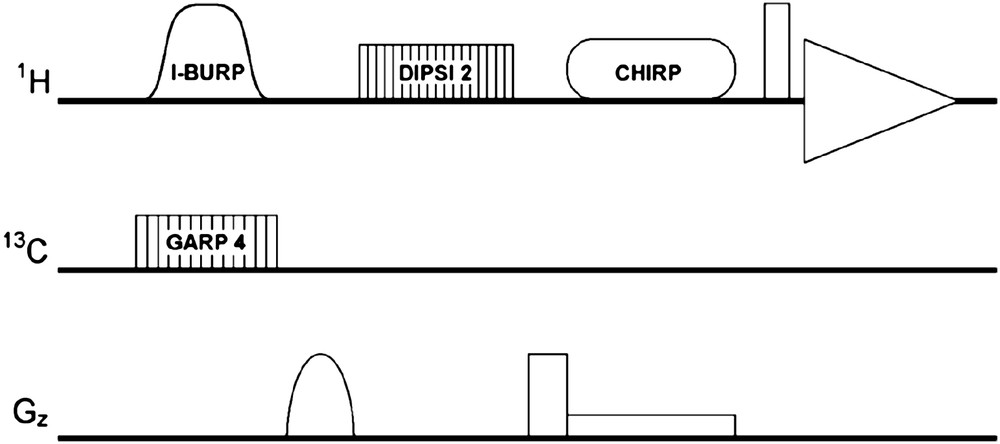
DANTE-Z ZQF-TOCSY pulse sequence. See text for details.
The pulse sequence (with phase cycles) and the AU program for Topspin® (Bruker spectrometers) of the DANTE-Z ZQF-TOCSY can be obtained freely upon request to Jean-Charles Portais (email: stephane.massou@insa-toulouse.fr).
3 Results and discussion
3.1 Selective excitation using DPFGSE and DANTE-Z
Fig. 1(1) shows the 1D 1H NMR spectrum of a 13C-labelled lysine sample, which contains the resonances from the five protonated carbons (α,β,γ,δ,ɛ) of this amino acid. Three resonances (β,γ,δ) are very close one to each other, with clear overlaps of the 1H–13C satellite lines that prevent the accurate integration of almost all signals in this region. This overlap still remains in 2D 1H,1H spectra (data not shown), which means that the specific 13C-enrichments of Lys β,γ, and δ carbons cannot be measured from classical 1D 1H or 2D 1H,1H NMR spectroscopy. The spectrum can be simplified by means of a selective excitation, but for the determination of 13C-enrichments, the two satellite lines as well as the central one have to be recovered. If the selective excitation is applied over the spectral region containing the three lines, then the overlapping signals will be excited as well, which is not desired. This problem can be solved by decoupling the 13C atoms during the selective excitation, so that the central and satellite lines fuse into only one signal that can be selectively excited (Fig. 1(2)). The signal acquisition itself is performed without 13C-decoupling so that the actual spectrum contains the heteronuclear couplings.
To evaluate the effectiveness of DANTE-Z and DPFGSE pulses to achieve the selective excitation of 13C-decoupled signals in a quantitative manner, they were applied first to a signal in a clear region of the spectrum (Hɛ). The two pulses were applied with the same excitation width (60 Hz), using I-BURP (D-DANTE-Z) and RE-BURP (DPFGSE) sequences. GARP4 13C-decoupling was applied during the selective excitation period. After the selective excitation, the 1H NMR spectra were acquired without 13C-decoupling. The results are displayed in Fig. 1(3) (DANTE-Z excitation) and 1.4 (DPFGSE excitation). In both cases, the satellites and the central lines of Hɛ multiplet have been well excited and the signal was roughly similar to that observed in the standard 1D 1H spectrum (Fig. 1(1)). A close examination of the spectra revealed that the relative intensity of the satellite lines in the DANTE-Z spectrum was almost identical to that observed in the standard 1H spectrum. As a result, the 13C-enrichment measured from the DANTE-Z spectrum (53.4%) was in close agreement with the value determined from the standard 1H spectrum (53.8%). In the DPFGSE spectrum, the relative intensity of the satellite lines was lower compared to the two other spectra, and the 13C-enrichment measured from the former spectrum was only 47.8%. Such discrepancy between the measured value and the expected one is likely to be due to relaxation effects. Protons attached to a 13C nucleus have shorter – transverse and longitudinal – relaxation times compared to the same protons attached to a 12C nucleus. The duration of the DPFGSE module is too long and leads to the partial relaxation of all protons, so that a fraction of the signal is lost prior to the acquisition. Because the relaxation is faster with 13C-coupled protons, the fraction of lost signal is wider for protons attached to 13C nuclei compared to protons attached to 12C nuclei. Such explanation is consistent with the underestimation of the 13C-enrichment calculated from the DPFGSE spectrum. The duration of the DANTE-Z pulse scheme is shorter than that of DPFGSE, and the former sequence is expected to be less sensitive to relaxation effects [9,10], as emphasized by the 13C-enrichment calculated from the spectrum displayed in Fig. 1(3), in close agreement with the expected one.
3.2 Specific 13C-enrichment of lysine Cβ and Cδ
The 13C-decoupled DANTE-Z sequence described above was applied for the determination of the 13C-enrichments of carbons β, δ and γ of lysine from their 1H signals, which tightly overlap in the 1H spectrum (Fig. 1(1)). The corresponding spectra of the Hβ, Hδ and Hγ are shown in Fig. 3(1–3), respectively. To evaluate the quality of these experiments, the three signals were summed using appropriate scaling factors, and the reconstructed spectrum so-obtained (filled line in Fig. 3(4)) was compared to the standard 1H-spectrum. The two spectra were closely similar, as shown from their difference (dotted line in Fig. 3(4)). This result indicated that the proposed method was reliable for providing selective excitations of the three lysine protons in a quantitative manner.
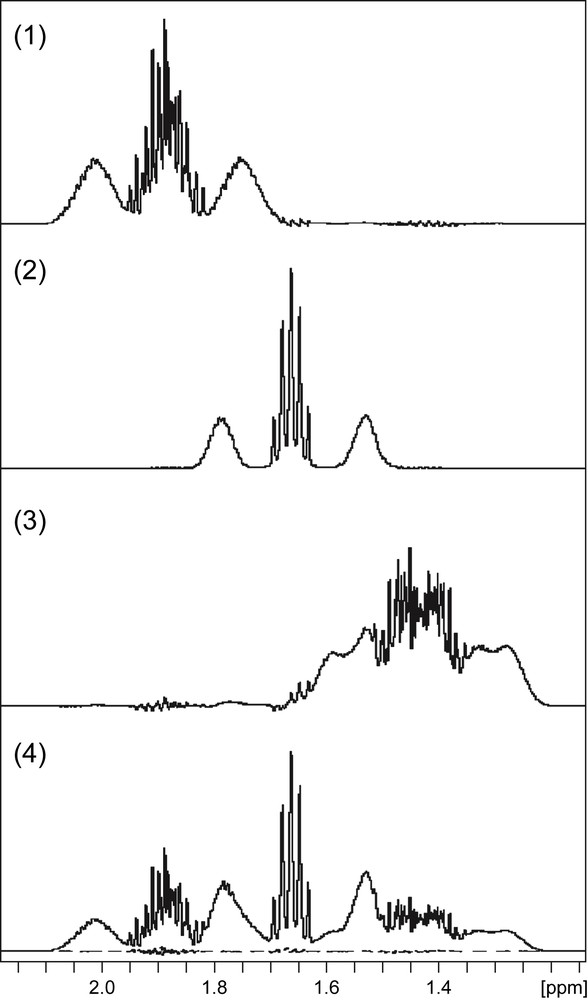
Spectrum simplification using 13C-decoupled DANTE-Z selective excitation. The spectrum obtained from the 13C-decoupled DANTE-Z selective excitation of Hβ (1), Hδ (2) and Hγ (3) protons of lysine. The spectra were recorded with no 13C-decoupling during the acquisition period. The satellite lines appear in each spectrum but do not overlap with other signals due to the selective excitation. (4) Scaled sum (filled line) of spectra shown in Fig. 3(1–3). The dotted lines below the spectrum shows the difference between the reconstructed spectrum and the 1D 1H spectrum of Fig. 1(1).
Because the lysine sample considered in these experiments consisted of a mixture of [U-13C6]- and unlabelled lysine, all carbons in the final sample have the same specific 13C-enrichment. The 13C specific enrichment measured from DANTE-Z spectra for the carbons β and δ of lysine were 52.6% and 53.9%, respectively. These values were in close agreement with the value obtained for the Cɛ carbon from the Hɛ signal (53.8%). The enrichment of the Cγ carbon could not be measured because the different satellite and central lines of this resonance are so large that they overlap in the actual spectrum and therefore they cannot be integrated properly.
3.3 Selective ZQF-TOCSY
The 13C-decoupled DANTE-Z experiment described above does not allow the measurement of 13C-enrichments from protons that are still overlapped by other signals in the 13C-decoupled 1H spectrum, because it is not possible to selectively excite the resonance of interest without exciting the overlapping signal. In that case, a different strategy can be applied if the resonance of interest can be excited via magnetization transfer. To test the efficiency of such approach, a pulse sequence combining 13C-decoupled DANTE-Z excitation and magnetization transfer using Zero-Quantum Filtered (ZQF) TOCSY was generated. The advantage of ZQF-TOCSY with respect to the determination of 13C-enrichments has been described elsewhere [5].
The DANTE-Z ZQF-TOCSY experiment was applied to transfer the magnetization from the Hɛ to the Hδ of lysine. The Hɛ protons were selectively excited using the 13C-decoupled DANTE-Z module, and then the Hɛ magnetization was transferred to the Hδ protons by means of the TOCSY isotropic mixing. The TOCSY transfer was restricted to the nearest proton by applying an isotropic mixing time (20 ms) short enough to avoid further transfer to the Hγ and Hβ protons. The resulting spectrum is shown in Fig. 4, where only the two signals corresponding to the Hɛ and the Hδ were observed. The 13C-enrichment of the Cδ carbon measured from the Hδ signal was 53.7%, a value which is in good agreement with the expected one.

Selective ZQF-TOCSY spectrum. The lysine Hɛ protons were selectively excited by a 13C-decoupled DANTE-Z pulse scheme. The Hɛ magnetization was subsequently transferred to the Hδ protons by a TOCSY mixing period of 20 ms. Zero-quantum coherences were suppressed by application of Zero-Quantum Filters.
The above results indicate that the combination of DANTE-Z and ZQF-TOCSY is a reliable tool for the determination of 13C-enrichment from proton signals embedded into a crowded region of the 1H NMR spectrum.
3.4 Ratio measurement between the two variants diaminopimelate pathway
In the bacterium C. glutamicum, lysine can be synthesized via two different processes: the dehydrogenase variant and the succinylase variant of the diaminopimelate pathway [14]. The two variants can be discriminated by means of 13C-labelling experiments [15]. When bacteria are cultured with [6-13C]-glucose as a carbon source, the flux ratio between the two metabolic pathways can be evaluated from the following formula [15]:
We have applied the above-described selective pulse schemes to the determination of the 13C-enrichments of the lysine and acetate produced by the bacteria, i.e. the lysine and acetate found in the culture supernatant. The 13C-enrichments of lysine Cδ and Cβ were found to be 49.9 and 46.4%, respectively, and that of acetate Cβ was 52.4%. From these values, the split ratio was calculated to be 41.1%.
4 Conclusion
The above results show that 13C-decoupled DANTE-Z pulse schemes can be profitably applied for the measurement of 13C-enrichments from 1H NMR spectra in situations where the signals of interest are embedded in a crowded region of the spectrum. Furthermore, such selective excitation can be combined to TOCSY experiment to transfer the magnetization to desired but not – directly or easily – accessible signals, which extends the range of NMR technique valuable for the measurement of 13C-enrichment in complex mixtures of metabolites.
Acknowledgements
This work was partially supported by grant No. JC8099 from the French Ministry for Education and Research. The NMR equipment used in the work was financed from grants by the Région Midi-Pyrénées and the European Regional Development Fund (ERDF).