1 Introduction
The oligomerization of ethylene as a major industrial process provides linear α-olefins, which are extensively used in the preparation of detergents, plasticizers, and fine chemicals and as comonomers in the production of linear low-density polyethylene (LLDPE). Linear α-olefins were originally manufactured by the Ziegler (Alfen) process [1] and the well-known Shell Higher Olefin Process (SHOP), which was developed with nickel complexes as catalysts [2]. Over the past decades, increasing interest has been focused on both modification of original ligands and exploration of new ligands for nickel catalysts. The recent review articles have covered the progress of nickel complexes as catalysts for ethylene reactivity [3]; in general, the nickel complexes perform catalytic activities in the assistance of combined multi-chelating ligands, such as N–N [4,5], P–N [6], N–O [7], N–N–O [8], N–P–N, P–N–P, P–O–P [9], P–N–N [9b,10] and N–N–N [11]. Approximately simultaneously with the above cationic catalysts, a class of neutral nickel catalysts based on salicylaldimine ligands [12] was reported independently by Johnson et al. [13] and Grubbs and coworkers [7a,7b]. Like SHOP-type catalyst containing P–O-based ligands, the monoanionic bidentate N–O-ligands and their nickel complexes deserve further investigation. In addition to designing catalysts for ethylene oligomerization and polymerization, it remains important to synthesize new compounds and find their application potential.
In this work, a series of 2-(1H-benzimidazol-2-yl)-phenols and their nickel complexes have been synthesized and characterized. These nickel complexes have been evaluated in the oligomerization of ethylene upon treatment with Et2AlCl. We report the synthesis and characterization of bis(2-(1H-benzimidazol-2-yl)-phenoxy) nickel and the investigation of their catalytic behavior for ethylene oligomerization. The influence of the ligand environment and of various reaction conditions, such as different cocatalysts, Al/Ni molar ratio, reaction temperature, reaction time as well the addition of auxiliary ligand PPh3, on the catalytic properties of the title nickel complexes will be discussed.
2 Results and discussion
2.1 Synthesis and characterization
The 2-(1H-benzimidazol-2-yl)-phenol derivatives (L1–3) were synthesized according to the previous reports [14], while the derivatives (L4–6) were prepared by the literature methods [15]. All these compounds were readily purified by recrystallization from their ethanol solutions and characterized by FT-IR, NMR and elemental analysis. Their nickel complexes were obtained by mixing the methanol solution of Ni(Ac)2·4H2O (Ac = MeCO2) and the corresponding ligands with KOH at room temperature (Scheme 1). The solution color was immediately changed from green to yellow with formation of a precipitate, which indicated that the coordination of 2-(1H-benzimidazol-2-yl)-phenol to nickel is fast. The resulting nickel complexes precipitated and were separated by filtration, washed with diethyl ether and dried in vacuum. All compositions of nickel complexes were consistent with their elemental analyses. Considering their IR spectra, the typical stretching frequencies of N–H (3058 cm−1) and CN (1622–1635 cm−1) bonds in C1–3 shifted to lower wave numbers, in comparison with their corresponding free ligands L1–3 (ν(N–H): 3236–3326 cm−1; ν(CN): 1632–1638 cm−1). In contrast, the stretching frequencies of CN bonds in C4–6 experienced little change; however, the peak intensity was greatly reduced. To clarify their molecular structures, ligand L4 and complex C5 were further analyzed by single-crystal X-ray diffraction.

Synthesis of nickel complexes C1–6.
Single crystals of the organic compound (L4) suitable for X-ray diffraction analysis were obtained by slow evaporation of its methanol solution, while single crystals of complex C5 were obtained by slow diffusion of diethyl ether into its methanol solution. Their molecular structures are shown in Figs. 1 and 3 and selected bond lengths and angles are listed in Table 1.

Crystal structure of ligand L4. Thermal ellipsoids are shown at 30% probability; hydrogen atoms and solvent have been omitted for clarity.
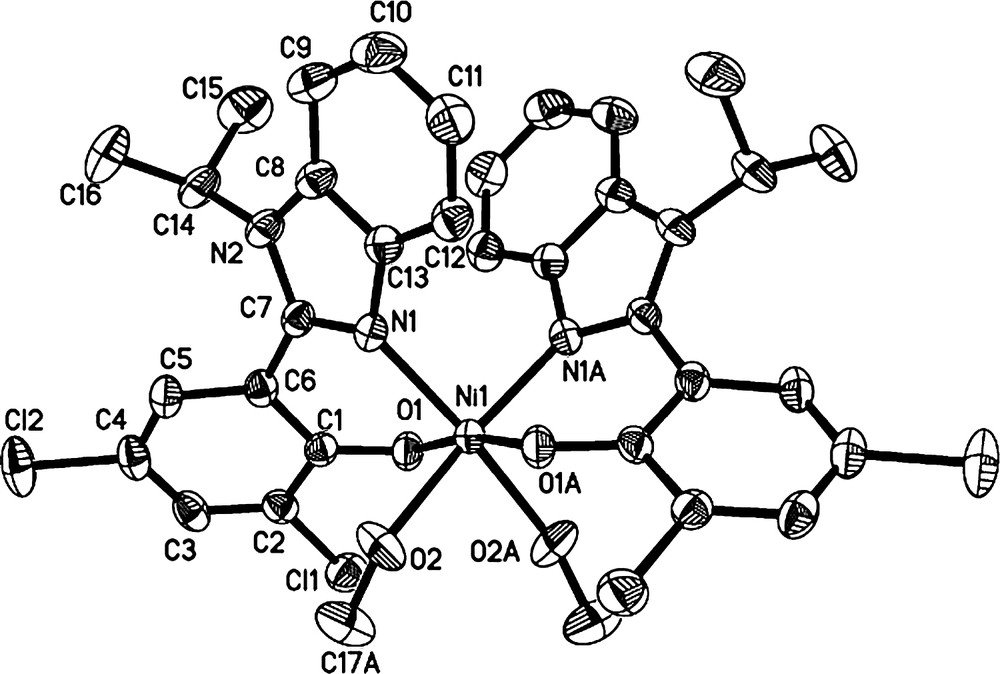
Molecular structure of C5. Thermal ellipsoids are shown at 30% probability; hydrogen atoms and solvent have been omitted for clarity.
Selected bond lengths (Å) and angles (°) for L4 and C5
L4 | C5 | ||
Bond lengths | |||
O(1)–C(1) | 1.353(3) | Ni(1)–O(1) | 2.0309(2) |
O(1)–H(1) | 0.8200 | Ni(1)–O(1A) | 2.0310(2) |
N(1)–C(7) | 1.359(3) | Ni(1)–N(1A) | 2.0569(2) |
N(1)–C(13) | 1.388(3) | Ni(1)–N(1) | 2.0569(2) |
N(1)–C(14) | 1.475(3) | Ni(1)–O(2) | 2.126(2) |
N(2)–C(7) | 1.322(3) | Ni(1)–O(2A) | 2.126(2) |
N(2)–C(8) | 1.397(3) | N(1)–C(7) | 1.331(3) |
Bond angles | |||
C(1)–O(1)–H(1) | 109.5 | N(1)–Ni(1)–O(2A) | 169.46(8) |
C(7)–N(1)–C(13) | 106.47(2) | O(1)–Ni(1)–N(1A) | 98.89(7) |
C(7)–N(1)–C(14) | 126.2(2) | O(1)–Ni(1)–N(1) | 88.27(7) |
C(13)–N(1)–C(14) | 127.2(2) | N(1A)–Ni(1)–N(1) | 90.76(1) |
C(7)–N(2)–C(8) | 104.58(2) | O(1)–Ni(1)–O(2) | 91.47(8) |
N(2)–C(7)–N(1) | 113.4(2) | O(2)–Ni(1)–O(2A) | 88.05(1) |
O(1)–Ni(1)–O(1A) | 169.85(1) |
In the solid state of L4, the benzimidazole plane and the phenol plane make a dihedral angle of 61.8o. Furthermore, hydrogen bonding interactions exist between ligand molecules and methanol molecules and form a 1-D infinite zigzag chain (Fig. 2). The O-atom O(2) from methanol forms hydrogen bond with H(1) (H(1)⋯O(2) = 1.834 Å) and the N atom N(2) from benzimidazole forms hydrogen bond to H(2) (H(2)⋯N(2) = 1.881 Å). The data of these hydrogen bond interactions are listed in Table 2.
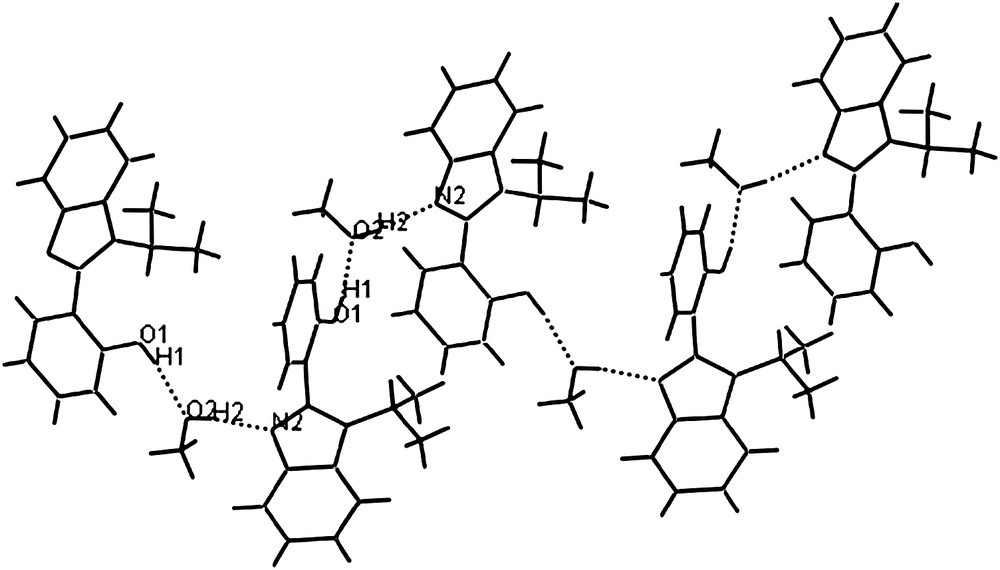
The 1-D chain formed by intermolecular hydrogen bond interactions in L4.
Hydrogen-bond interactions in L4
D–H⋯A | D⋯H/Å | H⋯A/Å | D⋯A/Å | ∠DHA/° |
C(1)–H(1)⋯O(2) | 0.820 | 1.834 | 2.642 | 168.71 |
O(2)–H(2)⋯N(2) | 0.820 | 1.881 | 2.693 | 170.24 |
Complex C5 shows a six-coordinated nickel core due to the coordination of two solvent molecules, in which the nickel center is surrounded by two ligands in a greatly distorted octahedral environment. The two oxygen atoms from two ligands lie in trans-positions, whereas the two nitrogen atoms are in cis-positions. The Ni–O bond (Ni(1)–O(2) = 2.126 Å) formed by methanol molecule is about 0.09 Å longer than that formed with the ligand (Ni(1)–O(1) = 2.031 Å). The length of the CN double bond (N(1)–C(7) = 1.331 Å) is slightly longer than that in ligand L4 (N(2)–C(7) = 1.322 Å). The bond angles around the nickel center are in the range of 88.05(1)° (O(1)–Ni(1)–N(1))–98.89(7)° (O(2)–Ni(1)–O(2A)).
2.2 Ethylene oligomerization
The catalytic activities of C1–6 for ethylene oligomerization have been carried out in the presence of diethylaluminum chloride (Et2AlCl). In all cases, these catalysts generate butenes and hexenes as main oligomeric products.
2.2.1 Ethylene oligomerization at ambient pressure
These nickel complexes were initially studied for their catalytic activities in ethylene oligomerization at ambient pressure. The effects of various cocatalysts, such as MAO, MMAO and Et2AlCl were studied in detail with C5 for the ethylene reactivity. The results indicated that different organoaluminums greatly influenced their catalytic behavior. The active Ni species was initially formed with alkylation by cocatalyst, and different cocatalysts showed characteristic features in activating nickel catalyst. The catalytic system with Et2AlCl showed the highest activity; therefore, our further studies were carried out with Et2AlCl as cocatalyst. The results at ambient pressure are collected in Table 3.
Results of ethylene oligomerization with C1–6 at ambient pressurea
Entry | Cat. | Cocat. | Al/Ni | Tb (°C) | tc (min) | Oligomer distributiond (%) | Activitye | ||
C4/∑C | C6/∑C | α-olefin (%) | |||||||
1 | C5 | MAO | 1000 | 20 | 30 | 87.3 | 12.7 | >21 | 0.2 |
2 | C5 | MMAO | 1000 | 20 | 30 | 97.0 | 3.0 | >25 | 1.19 |
3 | C5 | Et2AlCl | 100 | 20 | 30 | 91.6 | 8.4 | >24 | 2.19 |
4 | C5 | Et2AlCl | 200 | 20 | 30 | 92.2 | 7.8 | >26 | 2.51 |
5 | C5 | Et2AlCl | 500 | 20 | 30 | 93.7 | 6.3 | >28 | 1.42 |
6 | C5 | Et2AlCl | 700 | 20 | 30 | 95.1 | 4.9 | >31 | 1.03 |
7 | C5 | Et2AlCl | 200 | 0 | 30 | 95.3 | 4.7 | >25 | 1.96 |
8 | C5 | Et2AlCl | 200 | 40 | 30 | 96.8 | 3.2 | >58 | 0.058 |
9 | C5 | Et2AlCl | 200 | 60 | 30 | 100 | --- | >62 | 0.056 |
10 | C5 | Et2AlCl | 200 | 20 | 5 | 95.4 | 4.6 | >39 | 3.36 |
11 | C5 | Et2AlCl | 200 | 20 | 15 | 93.6 | 6.4 | >33 | 2.87 |
12 | C5 | Et2AlCl | 200 | 20 | 45 | 90.5 | 9.5 | >31 | 1.64 |
13 | C5 | Et2AlCl | 200 | 20 | 60 | 90.4 | 9.6 | >31 | 1.55 |
14 | C1 | Et2AlCl | 200 | 20 | 30 | 92.4 | 7.6 | >15 | 3.01 |
15 | C2 | Et2AlCl | 200 | 20 | 30 | 93.4 | 6.6 | >23 | 2.28 |
16 | C3 | Et2AlCl | 200 | 20 | 30 | 92.8 | 7.2 | >22 | 2.00 |
17 | C4 | Et2AlCl | 200 | 20 | 30 | 93.1 | 6.9 | >17 | 2.37 |
18 | C6 | Et2AlCl | 200 | 20 | 30 | 91.6 | 8.4 | >17 | 3.39 |
a Conditions: 5 μmol of catalysts; solvent: toluene (30 ml).
b Reaction temperature.
c Reaction time.
d Determined by GC.
e Oligomerization activity: 105 g mol−1 (Ni) h−1.
2.2.2 Effects of reaction parameters
The catalytic system of C5/Et2AlCl was typically investigated with varying reaction conditions, such as the molar ratio of Al/Ni and reaction temperature. The amount of Et2AlCl played an important role on the catalytic properties of the system. When the Al/Ni molar ratio was changed in the range of 100–700 (entries 3–6 in Table 3), the highest activity was observed at the Al/Ni ratio of 200 (2.51 × 105 g mol−1 (Ni) h−1, entry 4 in Table 3), while a higher molar ratio led to lower activity. A possible reason could be that a threshold amount of Et2AlCl as cocatalyst was necessary to efficiently activate the catalytic precursor; however, larger amounts of Et2AlCl might over-reduce the nickel species and cause their deactivation. This phenomenon was commonly observed in catalytic systems using late-transition metals; the direct evidence (intermediate) might be difficult to obtain due to excessive amounts of cocatalyst and the sensitivity of the intermediate formed.
The reaction temperature also had an important effect on the catalytic properties of the system, which remarkably affected the oligomerization activity, and the optimum activity was observed at the temperature of 20 °C (entry 4 in Table 3). The catalyst C5 showed higher oligomerization activity at lower temperature, and a further increase of the reaction temperature to 40 °C and 60 °C led to the sharp decrease of oligomerization activities (entries 8 and 9 in Table 3), which might be ascribed to the decomposition of the active catalytic sites and lower ethylene solubility at higher temperature.
The catalyst lifetime is one significant factor in industrial considerations. The effect of reaction time on catalytic activity was also studied using the C5/Et2AlCl system with Al/Ni molar ratio of 200 at 20 °C (entries 4 and 10–13 in Table 3). The oligomerization activity progressively decreased when the reaction was prolonged from 5 to 60 min, which indicated that the catalyst lifetime was relatively short. At the same time, the amount of higher carbon-number oligomers slightly increased with prolonged reaction time.
2.2.3 Effects of ligand environment
It can be observed that the ligand environment has considerable effects on the catalyst behavior, such as activity and distribution of products. Under similar conditions, the activity decreased in the order C3 < C2 < C1, which indicated that the more substituted the phenyl group, the lower the ethylene reactivity (entries 14–16 in Table 3). This can probably be attributed to the increasing nucleophilicity of the metal center with the higher number of methyl groups on the phenyl ring, which weakens the interaction between the nickel atom and the π-electrons of ethylene monomer and decreases the rate of ethylene insertion in the chain-growth steps.
On the other hand, the incorporation of an isopropyl group on the N atom of imidazole into the complexes led to a decrease in ethylene reactivity. As shown in Table 3, complex C4 showed lower activity than C1. Complexes C1–C3, containing N–H groups, showed relatively higher catalytic activities compared with other analogues. This could be possibly caused by their deprotonation to give anionic amide ligands when activated by Et2AlCl. The anionic amide ligands could be free or form N–Al species to increase their catalytic activity [14a].
It should be pointed out that the selectivity for linear α-olefins of oligomers was varied with the reaction parameters and ligand environments. However, no unambiguous trend could be identified. In general, the selectivity for α-olefins was relatively low. The low selectivity for linear α-olefins with the nickel complexes can be attributed to their ability to reversibly eliminate β-H after ethylene insertion and reinsert butene after chain transfer or to lead to isomerization of 1-butene by a re-uptake mechanism [6e]. Moreover, the ability of Ni(II) complexes to isomerize α-olefins was also observed in previous studies [5i,6b].
2.2.4 Effects of ethylene pressure
Ethylene oligomerization with complex C5 was also conducted under different ethylene pressures. The results are shown in Table 4. And at 30 atm, in the presence of 200 equiv of Et2AlCl, complex C5 showed an activity of 8.9 × 106 g mol−1(Ni) h−1 for ethylene oligomerization. It could be seen that the catalytic activities apparently increased under higher pressure, which could be attributed to the higher monomer concentration.
Results of ethylene oligomerization with C5 at different ethylene pressurea
Entry | Cat. | P (atm) | Oligomer distributionb (%) | Activityc | |
C4/∑C | C6/∑C | ||||
1 | C5 | 10 | 95.3 | 4.7 | 0.72 |
2 | C5 | 20 | 91.8 | 8.2 | 3.70 |
3 | C5 | 30 | 93.6 | 6.4 | 8.90 |
a Conditions: 5 μmol of catalysts; cocat: Et2AlCl, Al/Ni = 200; solvent: toluene (100 ml); reaction time: 30 min; reaction temperature: 20 °C.
b Determined by GC.
c Oligomerization activity: 106 g mol−1 (Ni) h−1.
2.2.5 Effects of auxiliary ligand (PPh3)
Our previous studies on nickel catalysts have demonstrated that the incorporation of PPh3 into the catalytic system can lead to higher activity and longer lifetime of the catalyst [5i,7g,11d]. Accordingly, complexes C1 and C5 were selectively studied with the effect of PPh3, and their results are summarized in Table 5. In the presence of 10 equiv PPh3 at ambient pressure, the catalytic activities of complexes C1 and C5 were clearly improved; while increasing ethylene pressure to 30 atm, their catalytic activities were greatly improved, with activities of 6.52 × 106 g mol−1 (Ni) h−1 (for C1) and 1.6 × 107 g mol−1 (Ni) h−1 (for C5), respectively (entries 3 and 4 in Table 5). Previously, an active species containing PPh3 was isolated [7g]; however, different catalytic systems involve different active species. The plausible effect of auxiliary ligand PPh3 is to associate and dissociate from the nickel center, and this influences the subsequent activation and protection of the active sites.
Oligomerization of ethylene with C1, C5/Et2AlCl/PPh3 systema
Entry | Cat. | P (atm) | Oligomer distributionb (%) | Activityc | |
C4/∑C | C6/∑C | ||||
1 | C1 | 1 | 93.3 | 6.7 | 1.10 |
2 | C5 | 1 | 93.0 | 7.0 | 1.44 |
3 | C1 | 30 | 91.1 | 9.1 | 6.52 |
4 | C5 | 30 | 93.0 | 7.0 | 16.0 |
a General conditions: 5 μmol complex; 30 ml toluene (1 atm), 100 ml toluene (30 atm); reaction time: 30 min; reaction temperature: 20 °C; cocat: Et2AlCl, Al/Ni = 200; 10 equiv of PPh3.
b Determined by GC.
c Oligomerization activity: 106 g mol−1 (Ni) h−1.
3 Conclusion
A series of nickel complexes containing 2-(1H-benzimidazol-2-yl)-phenoxy ligands were synthesized. X-ray determination revealed that the complexes adopt a six-coordinated distorted octahedral geometry. Activated by Et2AlCl, all nickel complexes exhibited considerably high catalytic activities for ethylene oligomerization with C4–C6 olefins as the main products. The ethylene oligomerization activity was found to be affected by the substituents in the ligand's framework and reaction parameters. The addition of PPh3 as an auxiliary ligand led to increased catalytic activity.
4 Experimental
4.1 General procedures
All manipulations of air- or moisture-sensitive compounds were carried out under atmosphere of argon using standard Schlenk techniques. IR spectra were recorded on PerkinElmer FT-IR 2000 spectrometer by using KBr disc in the range 4000–400 cm−1; 1H NMR and 13 C NMR spectra were recorded on a Bruker DMX-300 instrument with TMS as the internal standard. Elemental analysis was performed on a Flash EA1112 microanalyzer. GC analysis was performed with a VARIAN CP-3800 gas chromatograph equipped with a flame ionization detector and a 30-m (0.2-mm i.d., 0.25-μm film thickness) CP-Sil 5 CB column.
Solvents were dried by the appropriate drying reagents and distilled under nitrogen prior to use. Methylaluminoxane (MAO) was purchased from Albemarle as a 1.46 M solution in toluene. Modified methylaluminoxane (MMAO, 1.9 M in heptane, 3A) was purchased from Akzo Corp. Diethylaluminum chloride (Et2AlCl, 2 M in hexane) was purchased from Acros Chemicals. All other chemicals were obtained commercially and used without further purification unless otherwise stated.
4.2 Synthesis of ligands
4.2.1 2-(1H-Benzimidazol-2-yl)-phenol (L1)
Typical procedure is as follows: to a solution of salicylaldehyde (1.83 g, 15.0 mmol) in EtOH (50 ml), sodium metabisulfite (1.60 g, 8.5 mmol) in water was added in portions. The reaction mixture was stirred vigorously and more EtOH was added. The mixture was kept in a refrigerator for several hours. The precipitate was filtered and dried. The mixture of these salts and 1,2-phenylenediamine (0.43 g, 4.0 mmol) in DMF (10 ml) were heated at 130 °C for 4 h. The reaction mixture was cooled, poured into water, and the solid was filtered. After recrystallization from EtOH, the ligand was obtained. Yield: 80.9%. 1H NMR (CDCl3, 400 MHz): δ 6.96 (t, 1H, J = 7.08 Hz), 7.13 (d, 1H, J = 7.96 Hz), 7.31–7.39 (m, 2H), 7.58 (d, 2H, J = 7.32 Hz), 7.75 (s, 1H), 9.41 (s, 1H, N–H), 13.09 (s, 1H, O–H). 13C NMR (CDCl3, 75 MHz): δ 111.7, 116.1, 117.8, 121.6, 124.7, 130.3, 150.8, 157.3. IR (KBr disc, cm−1): 3326, 3057, 1632, 1590, 1492, 1419,1395, 1261, 1133, 840, 754, 727. Anal. Calc. for C13H10N2O: C, 74.27; H, 4.79; N, 13.33. Found: C, 74.42; H, 4.99; N, 13.14.
4.2.2 2-(6-Methyl-1H-benzimidazol-2-yl)-phenol (L2)
L2 was prepared by using the same procedure as the synthesis of L1 except that 4-methyl-o-phenylenediamine was used instead of 1,2-phenylenediamine. Yield: 75.0%. 1H NMR (CDCl3, 300 MHz): δ 2.50 (s, 3H, CH3), 6.96 (t, 1H, J = 7.56 Hz), 7.08–7.14 (m, 2H), 7.32–7.46 (m, 2H), 7.51–7.60 (m, 2H), 9.21 (s, 1H, N–H), 12.98 (s, 1H, O–H). 13C NMR (CDCl3, 75 MHz): δ 20.0, 111.7, 113.2, 116.0, 117.7, 123.0, 124.4, 128.2, 130.0, 131.1, 150.4, 157.1. IR (KBr disc, cm−1): 3236, 3082, 3058, 2913, 2858, 1732, 1697, 1638, 1599, 1487, 1420, 1390, 1322, 1235, 1161, 847, 819, 802. Anal. Calc. for C14H12N2O: C, 74.98; H, 5.39; N, 12.49. Found: C, 74.74; H, 5.45; N, 12.23.
4.2.3 2-(5,6-Dimethyl-1H-benzimidazol-2-yl)-phenol (L3)
L3 was prepared by using the same procedure as the synthesis of L1 except that 4,5-dimethylbenzene-1,2-diamine was used instead of 1,2-phenylenediamine. Yield: 60.2%. 1H NMR (CDCl3, 300 MHz): δ 2.38 (d, 6H, CH3), 6.95 (t, 1H, J = 6.85 Hz), 7.15 (t, 1H, J = 8.60 Hz), 7.33–7.41 (m, 3H), 7.58 (t, 1H, J = 7.24 Hz), 9.75 (s, 1H, N–H), 13.98 (s, 1H, O–H). IR (KBr disc, cm−1): 3259, 3051, 2977, 2923, 1638, 1583, 1531, 1490, 1466, 1438, 1416, 1386, 1287, 1240, 1167, 1038, 852. Anal. Calc. for C15H14N2O: C, 75.61; H, 5.90; N, 11.76. Found: C, 75.38; H, 6.01; N, 11.36.
4.2.4 2-(1-Isopropyl-1H-benzimidazole-2-yl)-phenol (L4)
L4 was prepared by using the same procedure as the synthesis of L1 except that N-isopropyl-benzene-1,2-diamine was used instead of 1.2-phenylenediamine. Yield: 77.3%. 1H NMR (CDCl3, 400 MHz): δ 1.74 (d, 6H, J = 6.88 Hz, CH3), 5.16–5.23 (m, 1H, C–H), 7.00 (t, 1H, J = 7.55 Hz), 7.17 (d, 1H, J = 8.26 Hz), 7.27–7.34 (m, 2H), 7.38 (t, 1H, J = 7.78 Hz), 7.46 (d, 1H, J = 7.83 Hz), 7.67 (d, 1H, J = 7.81 Hz), 7.78 (t, 1H, J = 5.68 Hz). 13C NMR (CDCl3, 75 MHz): δ 21.3, 49.32, 112.7, 113.7, 117.9, 118.8, 119.3, 122.4, 122.5, 141.8, 151.0, 158.0. IR (KBr disc, cm−1): 2979, 2935, 1544. 1934, 1894, 1778, 1608, 1523, 1457, 1389, 1288, 1230, 1180, 1132, 1102, 1043, 971, 914, 849, 747, 694, 667, 630. Anal. Calc. for C16H16N2O: C, 76.16; H, 6.39; N, 11.10. Found: C, 76.35; H, 6.35; N, 10.89.
4.2.5 2,4-Dichloro-6-(1-isopropyl-1H-benzimidazol-2-yl)-phenol (L5)
L5 was prepared by using the same procedure as the synthesis of L4 except that 3,5-dichloro-salicylaldehyde was used instead of salicylaldehyde. Yield: 87.4%, 1H NMR (CDCl3, 300 MHz): 1.69 (d, 6H, J = 6.93 Hz, CH3), 4.88–4.97 (m, 1H, C–H), 7.20 (t, 2H, J = 6.06 Hz), 7.25 (d, 1H, J = 2.20 Hz), 7.40 (d, 1H, J = 2.39 Hz), 7.56–7.60 (m, 2H). 13C NMR (CDCl3, 75 MHz): δ 21.3, 49.6, 112.6, 116.8, 119.1, 122.8, 123.1, 123.6, 124.5, 126.2, 131.1, 132.4, 141.0, 149.2, 152.6. IR (KBr disc, cm−1): 2046, 2987, 2939, 2389, 1755, 1611, 1588, 1500, 1455, 1423, 1369, 1291, 1269, 1198, 1134, 1057, 1014, 866, 747, 692, 640, 572. Anal. Calc. for C16H14Cl2N2O: C, 59.83; H, 4.39; N, 8.72. Found: C, 59.52; H, 4.40; N, 8.38.
4.2.6 2-(1-Isopropyl-1H-benzimidazol-2-yl)-6-methoxy-phenol (L6)
L6 was prepared by using the same procedure as the synthesis of L4 except that 5-methoxy-salicylaldehyde was used instead of salicylaldehyde. Yield: 82.0%. 1H NMR (CDCl3, 300 MHz): 1.64 (d, 6H, J = 6.93 Hz, CH3), 3.76 (s, 1H, CH3O), 4.87–4.96 (m, 1H, C–H), 6.76–6.80 (m, 1H), 6.90 (d, 1H, J = 2.97 Hz), 7.08 (d, 1H, J = 9.00 Hz), 7.29–7.36 (m, 2H), 7.61–7.66 (m, 1H), 7.82–7.85 (m, 1H). 13C NMR (CDCl3, 75 MHz): δ 21.3, 49.3, 55.8, 112.6, 113.1, 114.0, 116.9, 118.5, 119.4, 122.4, 122.6, 132.9, 135.1, 142.0, IR (KBr disc, cm−1): 2941, 2541, 1613, 1512, 1494, 1431, 1371, 1270, 1220, 1107, 1040, 821, 751. Anal. Calc. for C17H18N2O2 C, 72.32; H, 6.43; N, 9.92. Found: C, 72.38; H, 6.23; N, 9.72.
4.3 Synthesis of complexes C1–6
4.3.1 Bis[2-(1H-Benzimidazol-2-yl)-phenoxy] nickel (C1)
To a solution of L1 (0.106 g, 0.5 mmol) and KOH (0.028 g, 0.5 mmol) in methanol was added a solution of Ni(Ac)2·4H2O (0.062 g, 0.25 mmol) in methanol. The color changed immediately. And the reaction mixture was stirred for 10 h at room temperature. The resulting precipitate was filtered and dried in vacuum to yield C1 as a yellow powder in 81% yield. IR (KBr disc, cm−1): 3098, 3054, 2919, 2866, 2769, 1622, 1605, 1565, 1541, 1480, 1448, 1387, 1262, 913, 865, 765, 755, 728, 702, 550. Anal. Calc. for C26H18N4NiO2·CH3OH: C, 63.69; H, 4.35; N, 11.00. Found: C, 63.66; H, 3.95; N, 11.31.
4.3.2 Bis[2-(6-Methyl-1H-benzimidazol-2-yl)-phenoxy] nickel (C2)
In a manner similar to that described for C1, C2 was prepared as a yellow solid in 75% yield. IR (KBr disc, cm−1): 3058, 2972, 2919, 1622, 1606, 1565, 1540, 1481, 1458, 1448, 1328, 1309, 1263, 1141, 865, 765, 756, 727, 703, 549. Anal. Calc. for C28H22NiN4O2·CH3OH: C, 64.83; H, 4.88; N, 10.43. Found: C, 64.59; H, 4.48; N, 10.48.
4.3.3 Bis[2-(5,6-Dimethyl-1H-benzimidazol-2-yl)-phenoxy] nickel (C3)
In a manner similar to that described for C1, C3 was prepared as a yellow solid in 82% yield. IR (KBr disc, cm−1): 3062, 2966, 2933, 2357, 1635, 1606, 1556, 1535, 1481, 1448, 1378, 1320, 1263, 1138, 1038, 1017, 880, 843, 753, 697, 569. Anal. Calc. for C30H26N4NiO2·CH3OH: C, 65.87; H, 5.35; N, 9.91. Found: C, 65.64; H, 5.12; N, 10.17.
4.3.4 Bis[2-(1-Isopropyl-1H-benzimidazole-2-yl)-phenoxy] nickel (C4)
In a manner similar to that described for C1, C4 was prepared as a yellow solid in 75% yield. IR (KBr disc, cm−1): 2981, 2935, 1609, 1599, 1506, 1551, 1473, 1426, 1384, 1329, 1284, 1265, 1145, 1105, 1045, 1022, 861, 812, 741, 697, 647. Anal. Calc. for C32H30N4NiO2·2CH3OH: C, 65.30; H, 6.12; N, 8.96. Found: C, 65.03; H, 5.89; N, 8.67.
4.3.5 Bis[2,4-Dichloro-6-(1-isopropyl-1H-benzimidazol-2-yl)-phenoxy] nickel (C5)
In a manner similar to that described for C1, C5 was prepared as a yellow solid in 75% yield. IR (KBr disc, cm−1): 3080, 2977, 2880, 2938, 1612, 1588, 1498, 1459, 1448, 1385, 1300, 1248, 1155, 1135, 1107, 1052, 1017, 858. Anal. Calc. for C32H26Cl4N4NiO2·2CH3OH: C, 53.51; H, 4.49; N, 7.34. Found: C, 53.91; H, 4.42; N, 7.44.
4.3.6 Bis[2-(1-Isopropyl-1H-benzimidazol-2-yl)-6-methoxy-phenoxy] nickel (C6)
In a manner similar to that described for C1, C6 was prepared as a yellow solid in 77% yield. IR (KBr disc, cm−1): 3056, 2974, 2937, 2830, 1615, 1549, 1505, 1484, 1390, 1306, 1267, 1215, 1173, 1137, 1112, 1044, 931, 869, 822. Anal. Calc. for C34H34N4NiO4·2CH3OH: C, 63.08; H, 6.18; N, 8.17. Found: C, 62.78; H, 6.39; N, 7.90.
4.4 General procedure for ethylene oligomerization
4.4.1 Ethylene oligomerization at 1 atm of ethylene pressure
The catalytic precursor was dissolved in toluene in a Schlenk tube and the reaction solution was stirred under 1 atm ethylene at the controlled reaction temperature. The reaction was initiated by adding the desired amount of cocatalysts such as diethylaluminum chloride (Et2AlCl), methylaluminoxane (MAO) or MMAO. After the desired period of time, a small amount of the resulting solution was collected with syringe and quenched with 5% aqueous hydrogen chloride. An analysis by gas chromatography (GC) was carried out to determine the distribution of oligomers obtained.
4.4.2 Ethylene oligomerization at higher ethylene pressure
Ethylene oligomerization at elevated ethylene pressure was carried out in a 250-ml autoclave stainless steel reactor equipped with a mechanical stirrer and a temperature controller. Briefly, toluene, the desired amount of cocatalyst and toluene solution of catalytic precursor (the total volume was 100 ml) was added to the reactor in this order under an ethylene atmosphere. Reaching the desired reaction temperature, ethylene with the desired pressure was introduced to start the reaction, and the ethylene pressure was kept by constant feeding of ethylene. After 30 min, the reaction was stopped. A small amount of the reaction solution was collected, terminated by the addition of 5% aqueous hydrogen chloride and then analyzed by gas chromatography (GC) for determining the distribution of oligomers obtained.
4.5 X-ray crystal structure determination of L4 and C5
Single-crystal X-ray diffraction studies for ligand L4 and complex C5 were carried out on a Rigaku RAXIS Rapid IP diffractometer with graphite-monochromated Mo Kα radiation (λ = 0.71073 Å). Unit cell dimensions were obtained with least-squares refinements. Intensities were corrected for Lorentz and polarization effects and empirical absorption. The structures were solved by direct methods, and refined by full-matrix least-square on F2. Each hydrogen atom was placed in a calculated position, and refined using a riding model. All non-hydrogen atoms were refined anisotropically. Structure solution and refinement were performed using SHELXL-97 package [16]. Crystal date and processing parameters are summarized in Table 6. The data of L4 and C5 have been deposited with the Cambridge Crystallographic Data Center under CCDC 639668 and 639669, respectively.
Crystallographic data and refinement for L4 and C5
L4 | C5 | |
Formula | C17H20N2O2 | C35H38Cl4N4NiO5 |
Formula weight | 284.35 | 795.20 |
Crystal system | Orthorhombic | Orthorhombic |
Space group | P2(1)2(1)2(1) | Pccn |
a (Å) | 10.430(2) | 20.579(4) |
b (Å) | 12.033(2) | 8.8868(2) |
c (Å) | 12.468(3) | 20.278(4) |
V (Å3) | 1564.8(5) | 3708.5(1) |
Z | 4 | 4 |
Dcalc (g/cm−3) | 1.207 | 1.424 |
F(000) | 608 | 1648 |
θ range (°) | 2.35–27.40 | 2.69–27.35 |
Reflections collected | 2036 | 31735 |
Parameters | 192 | 238 |
Goodness-of-fit | 0.764 | 0.860 |
Final R indices [I > 2σ(I)] | R1 = 0.0376 | R1 = 0.0439 |
wR2 = 0.0628 | wR2 = 0.0996 | |
R indices (all data) | R1 = 0.0753 | R1 = 0.0812 |
wR2 = 0.0683 | wR2 = 0.1079 |
Acknowledgements
This project was supported by NSFC no 20473099.