1 Introduction
The basic mechanism of TiO2 photodegradation of organic compounds is widely accepted as photo excitation generating electrons–holes transferring to the TiO2 surface where an oxidation and reduction reactions occur. It is well known that OH radicals, which have a very high oxidation reduction potential (ORP) and oxidation reactivity, are generated as a product of such photoexcited reactions. Research has concentrated on the oxidation reactions between the OH radicals and variable substrates [1–5], but little attention has been given to the origin of the OH radicals or the transient species associated with the oxidation.
Flash photolysis is one of the most powerful means to study transient absorption. It may provide information on the intermediate product of the primary reaction that would only exist for a very short time. This information is important in determining the photoinduced reaction mechanism [6–10].
Pyrogallol, also called pyrogallic acid or 1,2,3-trihydroxybenzene, is a white crystalline powder and powerful reducing agent belonging to the phenol family. It is used as a photographic film developer, in the dyeing of hair and suturing materials, and for oxygen absorption in gas analysis. It also has antiseptic properties. Pyrogallol is not likely to be used as a modern hair dye due to the suspected toxicity of the compound.
In this study, transmission laser flash photolysis is employed to study the primary TiO2 photooxidation of pyrogallol. The initial oxidation dynamic of pyrogallol photooxidation was determined from the transient absorption spectra and the spectral change caused by pyrogallol concentration and dissolved oxygen.
2 Experimental
2.1 Instrumentation and materials
Time-resolved transient transmission measurements were performed using the third harmonic (355 nm, 5 ns full width at half-maximum) from a Q-switched Nd:YAG laser (Quanta-Ray, GCR-150). The transient signals were recorded with LKS 60 laser photolysis equipment. Steady-state UV–vis absorption was measured with a UV–vis spectrophotometer (Shimadzu, UV-1700). TiO2 powder suspension was sonicated in an ultrasonic cleanser (Branson, 3510).
TiO2 powder (P25, Degussa), pyrogallol (C6H3(OH)3, HPLC grade) and high-purity nitrogen were used during the experiments.
2.2 Sample preparation
TiO2 powder was suspended in 500 mL deionized water by sonicating for 10 min. The pH value of this suspension was 5.5. Through steady-state absorption spectra scanning, a TiO2 concentration with an ideal extinction value at 355 nm was selected as the subsequent experimental TiO2 concentration.
Concentrated pyrogallol solution was added to the TiO2 suspension and stirred to form a uniform mixture. The desired volume of the mixture and concentrations of pyrogallol and TiO2 were realized by adding suitable quantities of deionized water. The mixture was poured into a quartz cuvet, which was transparent on all sides, and the cuvet was placed inside the flash photolysis apparatus to produce a transmission system for the subsequent experiment. All procedures for the sample preparation were performed with shielding from UV light.
3 Results and discussion
3.1 Steady-state absorption spectra
Fig. 1 shows the UV–vis scanning spectra of 10, 20 and 25 mg/L TiO2 suspension. It was found that the absorbance over wavelengths of 200–600 nm had a very good linear correlation with the TiO2 concentration. For example, at a wavelength of 355 nm, the linear regression equation for the absorbance and concentration of TiO2 suspension was: A = 0.0327C + 0.0376 (R2 = 0.9981). The TiO2 concentration of 20 mg/L was selected as the subsequent experimental TiO2 concentration as it had the most ideal extinction value at 355 nm, namely the absorbance equaled 0.680, which was neither very high nor very low. After the suspension was left for 1 h, the absorption spectra of TiO2 suspension was measured again and showed scarcely any difference compared to the original spectra, which indicated that the TiO2 suspension was homogeneous and stable.
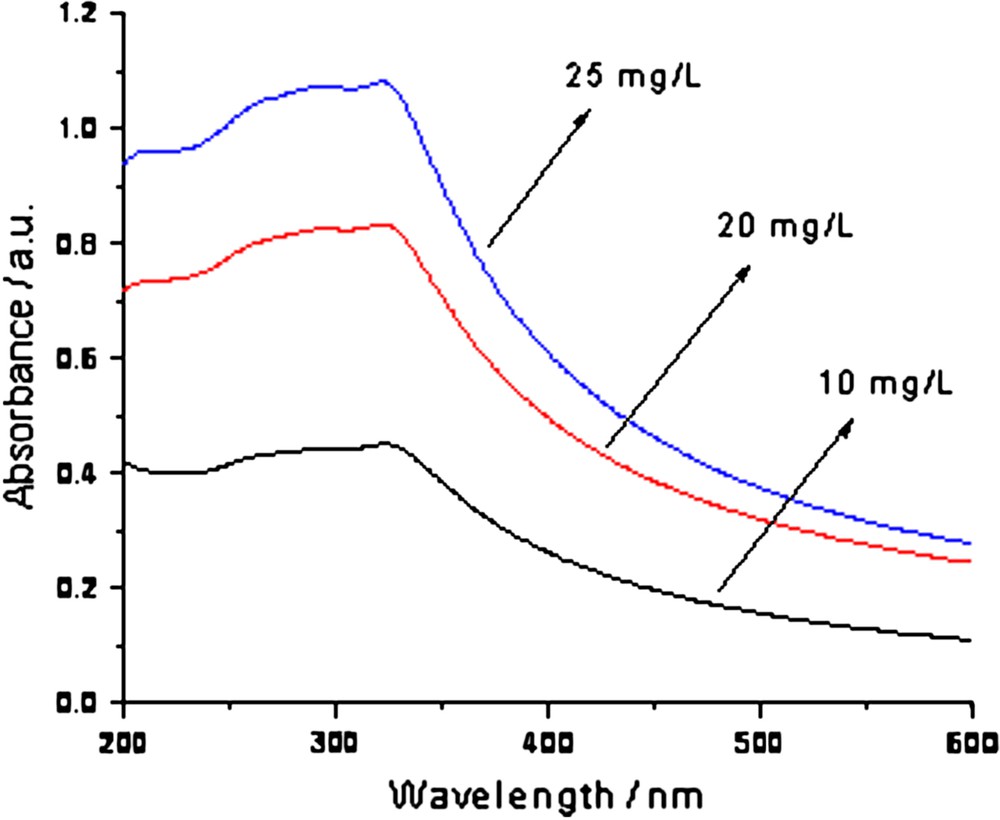
Absorbance spectra of TiO2 suspension.
Different amounts of pyrogallol concentrated solution were added to 20 mg/L TiO2 suspension to give different concentrations of pyrogallol and TiO2 mixed solutions. An absorption spectra measurement (Fig. 2) showed no influence on the absorbance at 355 nm compared to that of pure TiO2 suspension. That is, pyrogallol had no absorbance at 355 nm and it had no direct photolysis at this wavelength. This guaranteed that the function of TiO2 would be recognized if there was any photolysis of pyrogallol during the following experiment.

Absorbance spectra of TiO2 + pyrogallol mixture compared to pure TiO2 suspension.
3.2 Electron–hole generation and recombination
Fig. 3 depicts the transient absorption spectra observed for the 50 mg/L pyrogallol + 20 mg/L TiO2 mixture after 80 ns, 240 ns and 15 μs of laser excitation. The spectra recorded immediately after laser excitation (Δt = 80 ns) shows peak absorptions around 325 nm and 600 nm. Both the electron and the hole absorb at λ = 600 nm [11]. Electrons and holes then recombined rapidly and the peak absorbance dropped to zero after 15 μs of excitation. The peak absorption at 325 nm may indicate some new transient species formed, which could exist for a relatively longer time than the electrons and holes. To determine the characteristic of this transient species, 310 nm was selected as the subsequent detecting wavelength because the absorbance at 310 nm is more stable than that at 325 nm in the spectral range of the same peak.

Time-resolved absorption spectra recorded after 355-nm laser pulse excitation of a 50 mg/L pyrogallol + 20 mg/L TiO2 mixed solution.
3.3 Transient absorption with and without pyrogallol
The transient absorption of the 50 mg/L pyrogallol + 20 mg/L TiO2 mixture and 20 mg/L TiO2 pure solution after excitation detected at 310 nm is presented in Fig. 4. The recombination reaction of holes and electrons finished within 800 ns if no pyrogallol was added to the TiO2 suspension. However, when pyrogallol was added, the transient absorbance did not drop to zero after hole and electron recombination finished, but a low obvious absorbance lasted for a relatively long period of time. This must be attributable to some newly generated transient species with an absorption at 310 nm.
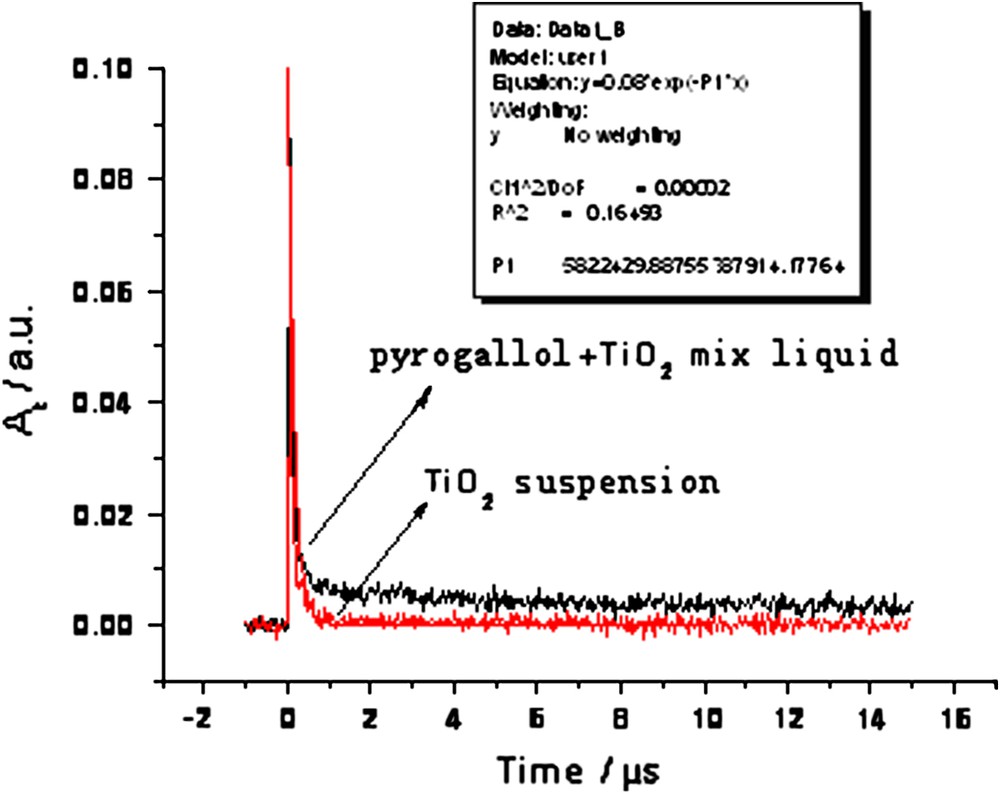
Transient absorption spectra obtained at 310 nm after flash photolysis, in 50 mg/L pyrogallol + 20 mg/L TiO2 mixed solution and 20 mg/L TiO2 pure solution.
From the time-resolved absorption curves in Fig. 4, it is also found that the recombination of holes and electrons finished in about 800 ns for pure TiO2. Some literature has reported that the average lifetime of holes and electrons is only a few tens of picoseconds [12]. This does not conflict with our observation because the recombination competes with the trapping of free charges by coordination defects at the surface and by lattice defects in the nanoparticle bulk. After photoexcitation, some of the electron–hole pairs recombine rapidly and some migrate to the TiO2 surface. Among the carriers on the surface of TiO2, 45% of the carriers descend into surface traps and react with substances adsorbed on the surface slowly thereafter (after about 200 ns), and 10% of the carriers enter deep inside the TiO2 nanoparticles and do not react at all [11,13,14]. The variation of transient absorption observed in this experiment, from its peak to zero, was due to the complicated generation and recombination of electrons and holes.
3.4 Transient absorption for different concentrations of pyrogallol
Fig. 5 shows the transient absorption results at 310 nm obtained as a function of pyrogallol concentration in the TiO2 solution. The absorbance signal increased with increasing pyrogallol concentration. This is due to more transient species being generated when the amount of pyrogallol absorbed on the surface of TiO2 was higher. There are two possibilities for the transient species. One is pyrogallol cations oxidized by holes and the other is the OH adducts formed from OH radicals.
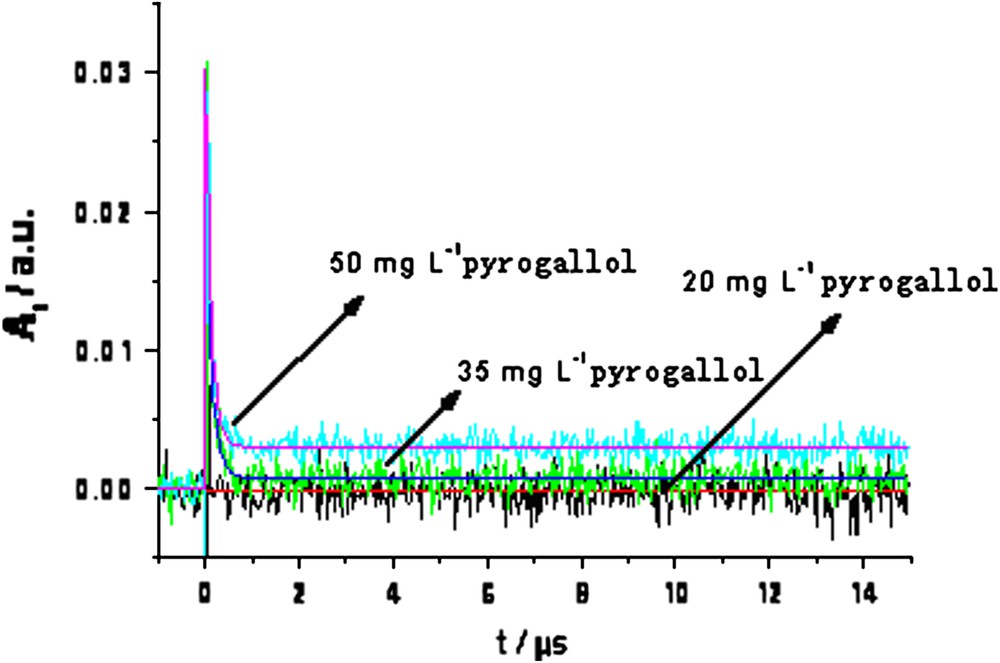
Transient absorption spectra obtained at 310 nm after flash photolysis, in different concentrations of pyrogallol + 20 mg/L TiO2 mixed solution.
3.5 Transient species determination
In order to determine the transient species present after laser photolysis of pyrogallol + TiO2 solution, the influence of dissolved oxygen was studied. A mixture of 50 mg/L pyrogallol + 20 mg/L TiO2 in a quartz cuvet was purged with high-purity nitrogen for 20 min to drive away dissolved oxygen. A flash photolysis experiment was conducted immediately after deoxygenation. The transient absorption spectra of both deoxy and air-equilibrated samples were similar, which suggested that the presence of oxygen had little effect on the transient absorption. If the transient species was an OH adduct, it should be easily oxidized by oxygen and the absorption signal of the oxygen-containing sample should attenuate obviously compared to the N2-saturated sample. This lack of sensitivity to oxygen suggests that the transient species was not any OH adduct, but pyrogallol cations that could not be oxidized by oxygen.
3.6 Primary mechanism of the photooxidation of pyrogallol by titanium dioxide
Based on the previous results, it is deduced that the initial TiO2 photocatalytic reaction mechanism for pyrogallol is the generation of photoexcited electrons–holes followed by the holes, instead of OH radicals, oxidizing pyrogallol into pyrogallol cations. The reactions are described as
TiO2 + hν → h+ + e− | (1) |
h+ + e− → heat | (2) |
h+ + pyrogallol → pyrogallol+ | (3) |
4 Conclusions
TiO2 photocatalytic oxidation reactions of pyrogallol were observed by time-resolved transmission flash photolysis. Compared with pure TiO2 suspension, transient absorption spectra of the pyrogallol and TiO2 mixture clearly indicated that some transient species besides electrons–holes were generated after laser-flash excitation. The higher the concentration of pyrogallol in the mixture, the stronger the transient absorption signal detected. The transient species was confirmed by a deoxygenation experiment to be pyrogallol cations oxidized by photo-generated holes, instead of pyrogallol OH adducts oxidized by OH radicals. Based on the above results, the primary oxidation mechanism of pyrogallol by TiO2 was deduced as the generation of electrons–holes immediately after photo excitation, followed by the holes transferring to the surface of the TiO2 and oxidizing pyrogallol into its cations.
Acknowledgements
We thank Dr. Bin Ouyang and Wenbo Dong for their generous help with the data analysis and valuable discussions. This work was supported by the National High-Tech R & D Program of China (2002AA601250).