1 Introduction
The Knoevenagel reaction [1] represents an important route to unsaturated α,β-dicarbonyl compounds [2]. The course of the reaction depends essentially on reactants and catalysts. Aldehydes and malonic esters undergo Knoevenagel condensation in the presence of secondary amines or their salts [3]. The reaction involving ketones requires Lewis acids such as titanium tetrachloride [4], zinc chloride [5–7] or zinc acetate [8].
In the last years solid supports were increasingly used with success. Under such mild conditions the synthesis of various organic compounds can be achieved with high selectivity through oxidation [11], reduction [12], alkylation [13], condensation [14,15], and acylation [16] reactions. The Knoevenagel reaction is usually catalyzed by weak bases [1] or by suitable combinations of amines and carboxylic [17] or Lewis [18] acids under homogeneous conditions. The Knoevenagel condensation can also be carried out on clays such as montmorillonite KSF [19,20], K10 [21], K10-ZnCl2 [22], bentonite [23] or other solid supports [24–27] such as alumina [28,29], silica gel [30,31], xonotlite [32,33], AlPO4–Al2O3 [29].
The present study aims at measuring the effect of water in the Knoevenagel reaction and using the results for a synthesis of coumarines.
2 Results and discussion
2.1 Knoevenagel reaction
In the presence of solid supports the reaction between active methylene compounds and carbonyl compounds affords the Knoevenagel product (Table 1). Reactions involving ketones are weakly promoted. With aldehydes, however, the reactivity is enhanced. This is ascribed to steric and inductive (+I) effects, which in the case of aldehydes decrease the electrophilicity of the carbon atom in the CHO group and, therefore, disfavours the attack of the intermediate carbanion formed in the first step (Scheme 1). As an illustration, benzaldehyde is more reactive than p-chlorobenzaldehyde as the Cl atom (mesomeric effect (+M)) weakens this electrophilicity. Indeed, the presence of chloride group causes increase of the barrier for the nucleophilic attack on the carbonyl group [34].
Effect of the types of solid supports on the yield of the Knoevenagel condensation
R1 | R2 | X | Y | Yield % (product) | ||||
A | B | C | D | E | ||||
C6H5 | H | CN | CN | 97 (1a) | 92 (1a) | 97 (1a) | 88 (1a) | 91 (1a) |
CN | CO2Me | 98 (2a) | 59 (2a) | 70 (2a) | 55 (2b) | 63 (2b) | ||
CO2Et | CO2Et | 97 (3a) | 45 (3a) | 56 (3a) | 26 (3c) | 36 (3c) | ||
p-ClC6H4 | H | CN | CN | 87 (4a) | 84 (4a) | 81 (4a) | 73 (4a) | 72 (4a) |
CN | CO2Me | 85 (5a) | 57 (5a) | 66 (5a) | 53 (5b) | 60 (5b) | ||
CO2Et | CO2Et | 86 (6a) | 44 (6a) | 55 (6a) | 28 (6c) | 33 (6c) | ||
H | CN | CN | 95 (7a) | 90 (7a) | 93 (7a) | 90 (7a) | 91 (7a) | |
CN | CO2Me | 98 (8a) | 63 (8a) | 75 (8a) | 61 (8b) | 69 (8b) | ||
CO2Et | CO2Et | 94 (9a) | 52 (9a) | 64 (9a) | 33 (9c) | 44 (9c) | ||
CH3 | H | CN | CN | 75 (10a) | 70 (10a) | 71 (10a) | 73 (10a) | 68 (10a) |
CN | CO2Me | 55 (11a) | 48 (11a) | 56 (11a) | – | – | ||
CO2Et | CO2Et | 59 (12a) | 47 (12a) | 58 (12a) | – | – | ||
n-C3H7 | H | CN | CN | 66 (13a) | 65 (13a) | 67 (13a) | – | – |
CN | CO2Me | 52 (14a) | 40 (14a) | 48 (14a) | – | – | ||
CO2Me | CO2Me | 51 (15a) | 30 (15a) | 37 (15a) | – | – | ||
CO2Et | CO2Et | 50 (16a) | 26 (16a) | 33 (16a) | – | – | ||
C6H5 | CH3 | CN | CN | 50 (17a) | 30 (17a) | 28 (17a) | 32 (17a) | 32 (17a) |
CN | CO2Et | 40 (18a) | 31 (18a) | 27 (18a) | 30 (18b) | 32 (18b) | ||
CO2Me | CO2Me | 38 (19a) | 27 (19a) | 25 (19a) | 33 (19c) | 31 (19c) | ||
CO2Et | CO2Et | 38 (20a) | 27 (20a) | – | – | – | ||
C2H5 | C2H5 | CN | CN | 52 (21a) | 33 (21a) | 36 (21a) | 35 (21a) | 38 (21a) |
CN | CO2Et | 44 (22a) | 34 (22a) | 31 (22a) | 38 (22b) | 32 (22b) | ||
CO2Et | CO2Et | 40 (23a) | 27 (23a) | – | – | – |
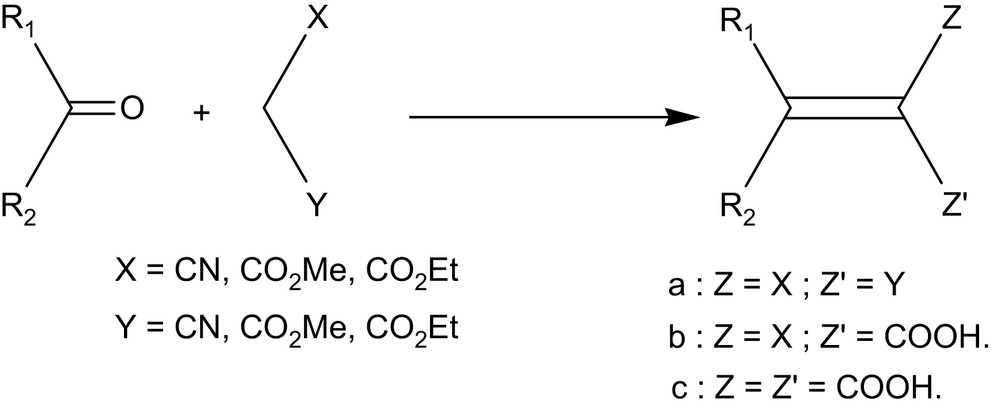
The results are also informative about the amphoteric character of clays. KSF and K10 montmorillonites have simultaneously basic and acid sites able to capture the proton of the labile hydrogen in the methylene compound. The basic sites ascribed to negative charges dispersed on the oxygen atoms [35] activate the Knoevenagel condensation. The acid sites which are due to the presence of water favour transesterification products when the active methylene compound bears an ester group, as shown by Ponde et al. [36].
As a consequence, the Knoevenagel condensation is promoted in the presence of water; however, transesterification takes place. The results may be interpreted in the sense that with water the active methylene groups come closer to the negative charges in the solid catalyst, favouring, thereby, the formation of carbanions. The Lewis acid centers of the clay activate the ester groups by coordination between the aluminum atom of the clay and the oxygen of the carbonyl group. Under such conditions the nucleophilic attack by water is favoured, leading to the formation of the corresponding acids (Scheme 2).

The results indicate that alumina is an excellent catalyst for the Knoevenagel condensation. The reaction is nearly quantitative in less than 5 min or so. The solid–liquid phase transfer process can be schematized as shown in Scheme 3.

Mechanism of the Knoevenagel condensation in the presence of alumina.
2.2 Synthesis of coumarines
Coumarines and their derivatives are natural products which are widely used for cosmetic, agricultural, pharmaceutical purposes [37]. Several synthetic ways have been proposed [19,24,38]. Two methods emerge: the Pechmann condensation [39] and the Knoevenagel reaction between salicylaldehyde and its derivatives with functionalized ethyl acetates (Scheme 4).

The 3-carboxylic coumarines are obtained in aqueous media while without solvent there is formation of the corresponding esters (Table 2). In agreement with Bigi's papers [19], our results show that coumarines can be synthesized in high yields in the presence of KSF montmorillonite. In the presence of water, however, hydrolysis of the ester group takes place.
Effect of the types of solid supports on the yield of coumarines and their derivatives
R1 | R2 | X | Yield % (product) | ||
F | G | H | |||
H | H | CN | 78 (24a) | 54 (24a) | 55 (24a) |
CO2Et | 95 (25a) | 67 (25a) | 72 (25b) | ||
H | OMe | CN | 86 (26a) | 78 (26a) | 66 (26a) |
CO2Et | 93 (27a) | 65 (27a) | 71 (27b) | ||
Cl | H | CN | 84 (28a) | 54 (28a) | 61 (28a) |
CO2Et | 96 (29a) | 67 (29a) | 73 (29b) | ||
OMe | H | CN | 78 (30a) | 51 (30a) | 56 (30a) |
CO2Et | 93 (31a) | 63 (31a) | 66 (31b) |
In this study, the preferred method uses alumina as the catalyst since under such conditions the reactivity is better than with KSF montmorillonite.
3 Conclusion
We have demonstrated a very simple and highly efficient method for the condensation of aldehydes with various active methylene compounds to give Knoevenagel products in excellent yields. We have found that coumarin-3-carboxylic esters could be synthesized in high yields by the Knoevenagel condensation in the presence of clay KSF and alumina. The reaction time is reduced to only a few minutes by using alumina as catalyst. We suggest that the present method may displace all other methods that use various organic solvents and catalysts.
4 Experimental section
4.1 General procedures
4.1.1 Procedure A
To a stirred solution of 10 mmol of aldehyde in 10 mmol of the methylene compound was added 3 g of alumina. After few minutes, the product was extracted with dichloromethane (3 × 20 mL). After removing the solvent under vacuum, the product is obtained with a good purity. The product was purified if necessary by crystallization or by flash chromatography using a mixture of hexane–ether as eluent.
4.1.2 Procedure B
Aldehyde (10 mmol), methylene compound (15 mmol) and KSF (1 g) were heated under stirring at 160 °C for 24 h. After cooling to room temperature, methanol (50 mL) was added and heated for 5 min. The catalyst was removed by Büchner filtration and the organic phase, concentrated under vacuum, was chromatographed on silica gel using a mixture of hexane–ether as eluent.
4.1.3 Procedure C
The same protocol that in procedure B, but with K10 instead of KSF.
4.1.4 Procedure D
The aldehyde (10 mmol), methylene compound (15 mmol) and KSF (1 g) in water (3.5 mL) were heated at reflux (100 °C) for 24 h. After cooling to room temperature, the solid is collected by Büchner filtration and solid was heated in methanol (50 mL) for 5 min. The catalyst was removed by Büchner filtration and washed with methanol. The solvent was removed in vacuo. The product was purified by flash chromatography using a mixture of hexane–ether as eluent.
4.1.5 Procedure E
The same protocol that in procedure D, but with K10 instead of KSF.
4.1.6 Procedure F
A mixture of a hydroxyaldehyde derivative (10 mmol), ethyl acetate derivative (15 mmol) and 3 g of alumina was stirred at room temperature. At the end, the reaction mixture was extracted with an appropriate solvent (3 × 20 mL) than recrystallized or purified by flash chromatography to effort the corresponding coumarine.
4.1.7 Procedure G
A mixture of a hydroxyaldehyde derivative (10 mmol), ethyl acetate derivative (15 mmol) and 1 g of KSF was heated under stirring at 160 °C for 24 h. After cooling to room temperature, methanol (50 mL) was added and heated for 5 min. The catalyst was removed by Büchner filtration and the organic phase, concentrated under vacuum, was chromatographed on silica gel using a mixture of hexane–ether as eluent.
4.1.8 Procedure H
A mixture of a hydroxyaldehyde derivative (10 mmol), ethyl acetate derivative (15 mmol), water (3.5 mL) and 1 g of KSF was heated at reflux (100 °C) for 24 h. After cooling to room temperature, the solid is collected by Büchner filtration and heated in methanol (50 mL) for 5 min. The catalyst was removed by Büchner filtration and washed with methanol. The solvent removed in vacuo. The product was purified by flash chromatography using a mixture of hexane–ether as eluent.
4.2 Characterization of compounds
1H NMR (300 MHz) and 13C NMR (75 MHz) spectra were recorded on a Bruker AC300 spectrometer using tetramethylsilane as internal reference. IR spectra were recorded with a JASCO FT/IR-420 spectrophotometer. Melting points were taken on a Reichert-Heizbank apparatus.
4.2.1 2-(Phenylmethylene)malononitrile 1a
Mp = 83–84 °C. IR: νCC = 1590 cm−1, νCN = 2224 cm−1. 1H NMR (300 MHz, CDCl3): δ 7.90 (d, J = 7.5 Hz, 2H), 7.79 (s, 1H), 7.64 (t, J = 7.2 Hz, 1H), 7.54 (dd, J = 7.2, 7.5 Hz, 2H). 13C NMR (75 MHz, CDCl3): δ 160.08, 134.75, 130.82, 129.81, 129.71, 113.79, 112.63, 82.85.
4.2.2 2-Cyano-3-phenyl-acrylic acid methyl ester 2a
Mp = 87–89 °C. 1H NMR (300 MHz, CDCl3): δ 8.27 (s, 1H), 8.00 (d, J = 7.2 Hz, 2H), 7.49–7.60 (m, 3H), 3.94 (s, 3H). 13C NMR (75 MHz, CDCl3): δ 166.20, 155.42, 133.49, 131.18, 130.14, 129.36, 117.37, 105.48, 53.47.
4.2.3 2-Cyano-3-phenyl-acrylic acid 2b
1H NMR (300 MHz, CDCl3): δ 10.86 (b, 1H), 8.26 (s, 1H), 7.67 (d, J = 8.1 Hz, 2H), 7.24–7.42 (m, 3H). 13C NMR (75 MHz, CDCl3): δ 169.76, 160.51, 134.19, 129.04, 127.87, 126.32, 118.72, 101.89.
4.2.4 2-Benzylidene-malonic acid diethyl ester 3a
1H NMR (300 MHz, CDCl3): δ 8.10 (s, 1H), 7.17–7.32 (m, 5H), 4.05 (q, J = 7.2 Hz, 4H), 1.13 (t, J = 7.2 Hz, 6H). 13C NMR (75 MHz, CDCl3): δ 167.95, 142.89, 134.09, 128.98, 128.60, 127.70, 127.31, 60.85, 14.45.
4.2.5 2-Benzylidene-malonic acid 3c
1H NMR (300 MHz, CDCl3): δ 10.86 (b, 2H), 7.98 (s, 1H), 7.69 (d, J = 8.1 Hz, 2H), 7.25–7.42 (m, 3H). 13C NMR (75 MHz, CDCl3): δ 170.00, 154.50, 134.39, 129.24, 127.80, 126.42, 122.05.
4.2.6 2-[(4-Chlorophenyl)methylene]malononitrile 4a
Mp = 162–164 °C. IR: νCC = 1585 cm−1, νCN = 2226 cm−1. 1H NMR (300 MHz, CDCl3): δ 7.84 (s, 1H), 7.69 (d, J = 8.4 Hz, 2H), 7.43 (d, J = 8.4 Hz, 2H). 13C NMR (75 MHz, CDCl3): δ 158.32, 141.22, 131.82, 130.16, 129.37, 113.45, 112.30, 83.45.
4.2.7 3-(4-Chloro-phenyl)-2-cyano-acrylic acid methyl ester 5a
1H NMR (300 MHz, CDCl3): δ 7.79 (s, 1H), 7.68 (d, J = 8.1 Hz, 2H), 7.33 (d, J = 8.1 Hz, 2H), 3.92 (s, 3H). 13C NMR (75 MHz, CDCl3): δ 167.02, 157.43, 140.40, 131.92, 130.26, 129.38, 117.60, 105.80, 53.62.
4.2.8 3-(4-Chloro-phenyl)-2-cyano-acrylic acid 5b
1H NMR (300 MHz, CDCl3): δ 10.85 (b, 1H), 7.73 (s, 1H), 7.58 (d, J = 8.4 Hz, 2H), 7.29 (d, J = 8.4 Hz, 2H). 13C NMR (75 MHz, CDCl3): δ 170.10, 158.94, 133.31, 133.02, 129.08, 127.86, 117.62, 102.05.
4.2.9 2-[(4-Chlorophenyl)methylene]malonic acid diethyl ester 6a
1H NMR (300 MHz, CDCl3): δ 8.09 (s, 1H), 7.64 (d, J = 8.4 Hz, 2H), 7.38 (d, J = 8.4 Hz, 2H), 4.13 (q, J = 7.2 Hz, 4H), 1.16 (t, J = 7.2 Hz, 6H). 13C NMR (75 MHz, CDCl3): δ 166.95, 148.90, 135.03, 134.12, 128.82, 127.72, 126.22, 60.28, 14.43, 14.46.
4.2.10 2-(4-Chloro-benzylidene)-malonic acid 6c
1H NMR (300 MHz, CDCl3): δ 10.86 (b, 2H), 7.94 (s, 1H), 7.34 (d, J = 8.1 Hz, 2H), 7.26 (d, J = 8.1 Hz, 2H). 13C NMR (75 MHz, CDCl3): δ 170.00, 155.14, 133.52, 133.41, 129.10, 127.92, 122.05.
4.2.11 2-Furan-2-ylmethylene-malononitrile 7a
1H NMR (300 MHz, CDCl3): δ 7.81 (s, 1H), 7.41 (d, J = 1.8 Hz, 1H), 6.27–6.35 (m, 2H). 13C NMR (75 MHz, CDCl3): δ 165.20, 156.10, 146.21, 114.42, 113.92, 112.95, 111.45, 83.42.
4.2.12 2-Cyano-3-furan-2-ylacrylic acid methyl ester 8a
1H NMR (300 MHz, CDCl3): δ 7.81–7.86 (m, 2H), 7.02 (d, J = 3.3 Hz, 1H), 6.66 (dd, J = 1.8, 3.3 Hz, 1H), 3.78 (s, 3H). 13C NMR (75 MHz, CDCl3): δ 166.03, 155.82, 155.43, 146.10, 117.22, 113.27, 112.08, 109.30, 52.61.
4.2.13 2-Cyano-3-furan-2-ylacrylic acid 8b
1H NMR (300 MHz, CDCl3): δ 10.85 (b, 1H), 7.80–7.86 (m, 2H), 7.00 (d, J = 3.3 Hz, 1H), 6.68 (dd, J = 1.8, 3.3 Hz, 1H).
4.2.14 2-Furan-2-ylmethylene-malonic acid diethyl ester 9a
1H NMR (300 MHz, CDCl3): δ 8.13 (s, 1H), 7.81 (d, J = 1.8 Hz, 1H), 7.05 (d, J = 3.3 Hz, 1H), 6.66 (dd, J = 1.8, 3.3 Hz, 1H), 4.17–4.25 (m, 4H), 1.27–1.32 (m, 6H). 13C NMR (75 MHz, CDCl3): δ 167.02, 156.40, 150.10, 145.91, 129.09, 113.07, 112.01, 61.42, 13.94.
4.2.15 2-Furan-2-ylmethylene-malonic acid 9c
1H NMR (300 MHz, CDCl3): δ 10.86 (b, 2H), 8.10 (s, 1H), 7.80 (d, J = 1.8 Hz, 1H), 7.05 (d, J = 3.3 Hz, 1H), 6.68 (dd, J = 1.8, 3.3 Hz, 1H).
4.2.16 2-Ethylidene-malononitrile 10a
1H NMR (300 MHz, CDCl3): δ 6.94 (q, J = 6.9 Hz, 1H), 1.83 (d, J = 6.9 Hz, 3H). 13C NMR (75 MHz, CDCl3): δ 159.61, 118.12, 83.27, 12.30.
4.2.17 2-Cyano-but-2-enoic acid methyl ester 11a
1H NMR (300 MHz, CDCl3): δ 6.95 (q, J = 6.9 Hz, 1H), 3.88 (s, 3H), 1.82 (d, J = 6.9 Hz, 3H). 13C NMR (75 MHz, CDCl3): δ 165.93, 153.24, 118.02, 106.50, 51.23, 13.19.
4.2.18 2-Ethylidene-malonic acid diethyl ester 12a
1H NMR (300 MHz, CDCl3): δ 7.19 (q, J = 6.9 Hz, 1H), 4.22 (q, J = 7.2 Hz, 4H), 1.78 (d, J = 6.9 Hz, 3H), 1.34 (t, J = 7.2 Hz, 6H). 13C NMR (75 MHz, CDCl3): δ 166.01, 147.07, 127.25, 62.06, 16.15, 14.37, 9.30.
4.2.19 2-Butylidene-malononitrile 13a
IR: νCC = 1607 cm−1, νCN = 2238 cm−1. 1H NMR (300 MHz, CDCl3): δ 7.33 (t, J = 7.5 Hz, 1H), 2.57 (q, J = 7.5 Hz, 3H), 1.58–1.70 (m, 2H), 1.02 (t, J = 7.5 Hz, 3H). 13C NMR (75 MHz, CDCl3): δ 168.42, 112.17, 90.28, 30.62, 21.31, 13.50.
4.2.20 2-Cyano-hex-2-enoic acid methyl ester 14a
1H NMR (300 MHz, CDCl3): δ 7.27 (t, J = 6.9 Hz, 1H), 3.83 (s, 3H), 2.05–2.11 (m, 2H), 1.34–1.42 (m, 2H), 1.12 (t, J = 7.2 Hz, 3H). 13C NMR (75 MHz, CDCl3): δ 166.15, 156.17, 117.12, 105.40, 53.20, 27.56, 23.21, 15.14.
4.2.21 2-Butylidene-malonic acid dimethyl ester 15a
1H NMR (300 MHz, CDCl3): δ 7.11 (t, J = 6.9 Hz, 1H), 3.82 (s, 6H), 2.05–2.11 (m, 2H), 1.35–1.42 (m, 2H), 1.07 (t, J = 7.2 Hz, 3H). 13C NMR (75 MHz, CDCl3): δ 165.21, 149.65, 128.87, 54.51, 34.01, 22.06, 15.12.
4.2.22 2-Butylidene-malonic acid diethyl ester 16a
1H NMR (300 MHz, CDCl3): δ 7.12 (t, J = 6.9 Hz, 1H), 4.20 (q, J = 7.2 Hz, 4H), 2.04–2.10 (m, 2H), 1.32–1.44 (m, 8H), 1.08 (t, J = 7.2 Hz, 3H). 13C NMR (75 MHz, CDCl3): δ 165.22, 152.56, 126.15, 60.69, 28.21, 23.01, 15.61, 14.17.
4.2.23 2-(1-Phenylethylidene)malononitrile 17a
Mp = 93–95 °C. IR: νCC = 1586 cm−1, νCN = 2228 cm−1. 1H NMR (300 MHz, CDCl3): δ 7.46–7.58 (m, 5H), 2.64 (s, 3H). 13C NMR (75 MHz, CDCl3): δ 175.41, 135.86, 132.26, 129.15, 127.32, 112.70, 84.67, 24.20.
4.2.24 2-Cyano-3-phenyl-but-2-enoic acid ethyl ester 18a
1H NMR (300 MHz, CDCl3): δ 7.47–7.60 (m, 5H), 4.24 (q, J = 7.2 Hz, 2H), 2.19 (s, 3H), 1.67 (t, J = 7.2 Hz, 3H). 13C NMR (75 MHz, CDCl3): δ 168.14, 165.91, 134.29, 128.94, 128.02, 126.90, 117.92, 94.76, 61.59, 24.13, 14.87.
4.2.25 2-Cyano-3-phenyl-but-2-enoic acid 18b
1H NMR (300 MHz, CDCl3): δ 10.86 (b, 1H), 7.38–7.58 (m, 5H), 2.14 (s, 3H). 13C NMR (75 MHz, CDCl3): δ 169.77, 168.74, 135.04, 129.14, 128.07, 126.86, 117.82, 94.36, 23.17.
4.2.26 2-(1-Phenyl-ethylidene)-malonic acid dimethyl ester 19a
1H NMR (300 MHz, CDCl3): δ 7.27–7.40 (m, 5H), 3.96 (s, 6H), 2.26 (s, 3H). 13C NMR (75 MHz, CDCl3): δ 166.50, 158.62, 133.94, 128.84, 127.97, 127.21, 120.21, 56.20, 19.70.
4.2.27 2-(1-Phenyl-ethylidene)-malonic acid 19c
1H NMR (300 MHz, CDCl3): δ 10.85 (b, 2H), 7.37–7.56 (m, 5H), 2.20 (s, 3H). 13C NMR (75 MHz, CDCl3): δ 169.86, 163.84, 135.02, 129.23, 128.05, 126.73, 114.14, 20.07.
4.2.28 2-(1-Phenyl-ethylidene)-malonic acid diethyl ester 20a
1H NMR (300 MHz, CDCl3): δ 7.17–7.39 (m, 5H), 4.22 (q, J = 7.2 Hz, 4H), 2.16 (s, 3H), 1.41 (t, J = 7.2 Hz, 6H). 13C NMR (75 MHz, CDCl3): δ 166.51, 158.62, 135.41, 129.14, 128.07, 127.12, 118.52, 62.13, 25.30, 14.37.
4.2.29 2-(1-Ethyl-propylidene)-malononitrile 21a
1H NMR (300 MHz, CDCl3): δ 2.41 (q, J = 7.5 Hz, 4H), 1.26 (t, J = 7.5 Hz, 6H). 13C NMR (75 MHz, CDCl3): δ 180.62, 118.21, 78.27, 24.13, 13.05.
4.2.30 2-Cyano-3-ethyl-pent-2-enoic acid ethyl ester 22a
1H NMR (300 MHz, CDCl3): δ 4.27 (q, J = 7.5 Hz, 2H), 2.40 (q, J = 7.5 Hz, 4H), 1.37 (t, J = 7.5 Hz, 3H), 1.28 (t, J = 7.5 Hz, 6H). 13C NMR (75 MHz, CDCl3): δ 174.52, 168.17, 117.29, 96.51, 62.19, 29.16, 17.61, 16.17, 14.10.
4.2.31 2-Cyano-3-ethyl-pent-2-enoic acid 22b
1H NMR (300 MHz, CDCl3): δ 10.85 (b, 1H), 2.28 (q, J = 7.5 Hz, 4H), 1.19 (t, J = 7.5 Hz, 6H). 13C NMR (75 MHz, CDCl3): δ 175.92, 171.53, 117.20, 96.44, 24.10, 13.57.
4.2.32 2-(1-Ethyl-propylidene)-malonic acid diethyl ester 23a
1H NMR (300 MHz, CDCl3): δ 4.24 (q, J = 7.5 Hz, 4H), 2.38 (q, J = 7.5 Hz, 4H), 1.67 (t, J = 7.5 Hz, 6H), 1.18 (t, J = 7.5 Hz, 6H). 13C NMR (75 MHz, CDCl3): δ 172.10, 166.35, 116.75, 62.96, 24.31, 15.37, 14.50.
4.2.33 3-Cyanocoumarin 24a
Mp = 183–185 °C. IR: νCO = 1730 cm−1, νCN = 2230 cm−1, νCC = 1600 cm−1. 1H NMR (300 MHz, CDCl3): δ 8.38 (s, 1H), 7.71 (dd, J = 9, 3 Hz, 1H), 7.60 (td, J = 8.4, 3 Hz, 1H), 7.37–7.45 (m, 2H). 13C NMR (75 MHz, CDCl3): δ 160.12, 156.50, 148.53, 130.41, 126.60, 120.50, 120.30, 118.90, 116.21, 102.82.
4.2.34 Ethyl-3-coumarincarboxylate 25a
Mp = 93–95 °C. IR: νCO = 1754 cm−1, νCC = 1610 cm−1. 1H NMR (300 MHz, CDCl3): δ 8.67 (s, 1H), 7.64 (dd, J = 9, 3 Hz, 1H), 7.51 (ddd, J = 9, 8.4, 2.7 Hz, 1H), 7.31–7.42 (m, 2H), 4.21 (q, J = 7.2 Hz, 2H), 1.38 (t, J = 7.2 Hz, 3H). 13C NMR (75 MHz, CDCl3): δ 164.42, 161.76, 155.16, 148.24, 132.24, 130.10, 129.40, 124.80, 120.75, 119.97, 64.70, 16.72.
4.2.35 Coumarin-3-carboxylic acid 25b
Mp = 190–192 °C. 1H NMR (300 MHz, CDCl3): δ 10.86 (b, 1H), 8.47 (s, 1H), 7.59–7.65 (m, 2H), 7.30–7.40 (m, 2H). 13C NMR (75 MHz, CDCl3): δ 169.12, 161.41, 155.10, 148.08, 131.21, 129.14, 124.18, 120.70, 119.82, 118.94.
4.2.36 8-Methoxy-2-oxo-2H-chromene-3-carbonitrile 26a
1H NMR (300 MHz, CDCl3): δ 8.51 (s, 1H), 7.24 (dd, J = 8.7, 2.7 Hz, 1H), 7.17 (t, J = 8.7 Hz, 1H), 7.01 (dd, J = 8.7, 2.7 Hz, 1H), 3.93 (s, 3H). 13C NMR (75 MHz, CDCl3): δ 161.26, 160.02, 155.65, 137.46, 129.14, 127.04, 119.01, 118.12, 114.03, 101.21, 61.11.
4.2.37 8-Methoxy-2-oxo-2H-chromene-3-carboxylic acid ethyl ester 27a
1H NMR (300 MHz, CDCl3): δ 8.78 (s, 1H), 7.26 (dd, J = 8.7, 3 Hz, 1H), 7.14 (dd, J = 8.7, 8.1 Hz, 1H), 7.00 (dd, J = 8.1, 3 Hz, 1H), 4.22 (q, J = 7.2 Hz, 2H), 3.92 (s, 3H), 1.35 (t, J = 7.2 Hz, 3H). 13C NMR (75 MHz, CDCl3): δ 164.80, 159.72, 155.10, 152.51, 135.21, 129.01, 126.13, 123.19, 118.72, 117.94, 63.92, 60.40, 15.67.
4.2.38 8-Methoxy-2-oxo-2H-chromene-3-carboxylic acid 27b
1H NMR (300 MHz, CDCl3): δ 10.84 (b, 1H), 8.67 (s, 1H), 7.56 (dd, J = 9, 2.7 Hz, 1H), 7.27 (dd, J = 9, 8.4 Hz, 1H), 7.03 (dd, J = 8.4, 2.7 Hz, 1H), 3.91 (s, 3H). 13C NMR (75 MHz, CDCl3): δ 171.20, 160.42, 155.54, 154.48, 134.39, 129.08, 127.10, 122.32, 118.70, 114.97, 60.03.
4.2.39 7-Chloro-2-oxo-2H-chromene-3-carbonitrile 28a
1H NMR (300 MHz, CDCl3): δ 8.48 (s, 1H), 7.59 (d, J = 8.7 Hz, 1H), 7.26 (dd, J = 8.7, 2.4 Hz, 1H), 7.22 (d, J = 2.4 Hz, 1H). 13C NMR (75 MHz, CDCl3): δ 163.12, 159.96, 154.62, 134.19, 128.98, 126.89, 126.19, 122.76, 117.82, 102.18.
4.2.40 7-Chloro-2-oxo-2H-chromene-3-carboxylic acid ethyl ester 29a
1H NMR (300 MHz, CDCl3): δ 8.79 (s, 1H), 7.61 (d, J = 8.7 Hz, 1H), 7.29 (dd, J = 8.7, 2.1 Hz, 1H), 7.23 (d, J = 2.1 Hz, 1H), 4.23 (q, J = 7.5 Hz, 2H), 1.67 (t, J = 7.5 Hz, 3H). 13C NMR (75 MHz, CDCl3): δ 167.15, 163.10, 153.06, 152.69, 134.54, 128.91, 127.07, 126.36, 123.13, 121.97, 61.06, 15.17.
4.2.41 7-Chloro-2-oxo-2H-chromene-3-carboxylic acid 29b
1H NMR (300 MHz, CDCl3): δ 10.85 (b, 1H), 8.69 (s, 1H), 7.59 (d, J = 8.7 Hz, 1H), 7.30 (dd, J = 8.7, 2.1 Hz, 1H), 7.22 (d, J = 2.1 Hz, 1H). 13C NMR (75 MHz, CDCl3): δ 170.31, 162.43, 156.04, 152.52, 134.34, 129.02, 126.12, 125.96, 122.07, 121.95.
4.2.42 7-Methoxy-2-oxo-2H-chromene-3-carbonitrile 30a
1H NMR (300 MHz, CDCl3): δ 8.46 (s, 1H), 7.53 (d, J = 8.7 Hz, 1H), 6.74 (dd, J = 8.7, 2.1 Hz, 1H), 6.69 (d, J = 2.1 Hz, 1H), 3.87 (s, 3H). 13C NMR (75 MHz, CDCl3): δ 162.16, 160.61, 158.98, 152.81, 127.96, 121.04, 117.32, 111.42, 108.19, 100.98, 57.61.
4.2.43 7-Methoxy-2-oxo-2H-chromene-3-carboxylic acid ethyl ester 31a
1H NMR (300 MHz, CDCl3): δ 8.78 (s, 1H), 7.51 (d, J = 8.7 Hz, 1H), 6.74 (d, J = 8.7 Hz, 1H), 6.70 (s, 1H), 4.23 (q, J = 7.5 Hz, 2H), 3.81 (s, 3H), 1.63 (t, J = 7.5 Hz, 3H). 13C NMR (75 MHz, CDCl3): δ 166.31, 163.02, 161.16, 153.62, 150.86, 128.06, 123.12, 121.21, 112.81, 107.36, 62.09, 57.61, 15.37.
4.2.44 7-Methoxy-2-oxo-2H-chromene-3-carboxylic acid 31b
1H NMR (300 MHz, CDCl3): δ 10.87 (b, 1H), 8.77 (s, 1H), 7.52 (d, J = 8.7 Hz, 1H), 6.74 (dd, J = 8.7, 2.1 Hz, 1H), 6.72 (d, J = 2.1 Hz, 1H), 3.78 (s, 3H). 13C NMR (75 MHz, CDCl3): δ 171.02, 162.00, 160.86, 154.54, 150.58, 127.96, 122.05, 121.00, 111.18, 107.69, 57.02.