1 Introduction
First interest for lanthanide ions in molecular magnetism resulted from seminal work by Gatteschi and Kahn who showed that ferromagnetic interactions take place between GdIII and CuII when assembled by Schiff base ligands [1–3]. Such a ferromagnetic behavior was also found between GdIII and aminoxyl radical units [4–6]. This has opened the way for a more systematic investigation [7–9] of the magnetic exchange coupling taking place between the paramagnetic Ln ions and either 3d ions [10–17] or organic radicals [18–23]. In recent years, the lanthanide ions have attracted a renewed interest in molecular magnetism since it has been found that those Ln ions with orbital momentum can lead to slow relaxation of the magnetization when involved in low dimensional compounds [24–27].
Compartmental ligands derived from Schiff bases have played an important role in the development of heteronuclear {3d–4f} species since they permit a controlled preparation of bi- and trimetallic compounds. Considering that such binuclear compounds can be assembled in larger species by the means of a bridging unit [28], we have envisaged the assemblage of {3d–4f} units with a complementary building unit that also ensures for efficient magnetic interactions among the magnetic centers. We disclose here two compounds obtained by assembling {LMe2NiLn}3+ (see Scheme 1) and {W(CN)8}3− units with Ln = Y (1) and La (2). The diamagnetic Ln ions Y and La have been considered in order to confirm the anticipated ferromagnetic {Ni–W} interaction and to evaluate the strength of the coupling. Rather unexpectedly, the two compounds have been found to exhibit different supramolecular organizations. Their crystal structures and magnetic properties are reported.

Binuclear {LMe2NiLn} unit.
2 Results and discussion
Synthesis and structural features. The reaction of {LMe2NiLn(NO3)3} (Ln = Y for 1 and La for 2, LMe2 see Scheme 1) with Cs3{W(CN)8} in H2O resulted in the formation of the heterotrinuclear compounds [{LMe2NiY(H2O)4}{W(CN)8}]∞·9.5H2O, 1, and [{LMe2NiLa(H2O)4.5}2{W(CN)8}2]·9H2O, 2. Slow inter-diffusion of the reagent solutions yielded well-shaped crystals and precipitate, only the crystals have been used for the forthcoming investigation. The IR spectra for compounds 1 and 2 are very similar except for the signature of the CN ligands. Compound 1 exhibits two bands with νCN = 2167 and 2147 cm−1 whereas 2 is characterized by a broad pattern with several overlapping bands at νCN = 2149, 2160, and 2176 cm−1, suggesting a different coordination scheme for the cyanometalate units.
Compound 1 crystallizes in the Pca21 space group, and there are two independent [{LMe2NiY(H2O)4}{W(CN)8}] fragments in the asymmetric unit (Fig. 1). The structure of 1 consists of a 1D polymeric structure based on the alternation of {LMe2NiY(H2O)4} and {W(CN)8} units (Fig. 2). Each {W(CN)8} moiety is linked to two {LMe2NiY(H2O)4} units through WCN → Ni linkages and each {LMe2NiY(H2O)4} accommodates two W modules in trans position, thus developing the coordination polymer. The Ni–N(NC) distances lie between 2.04(1) and 2.13(2) Å. The Ni–N–C angles span from 160(2) to 164(2)° and the W–C–N angles are found between 171(2) and 178(2)° (see Fig. 1 caption). The coordination sphere of the Y ion consists of eight oxygen atoms, four are from the LMe2 ligand and four from H2O molecules. The crystal lattice is completed by 9.5·H2O molecules leading for compound 1 to the formula [{LMe2NiY(H2O)4}{W(CN)8}]∞·9.5H2O. Several of the lattice H2O molecules are engaged into H-bonds with the cyanide ligands of the W units. The two independent chains developed from the asymmetric unit are bridged by a H2O (O20) that forms H-bonds with two {W(CN)8} units, realizing a ladder-type organization (Fig. 2). The existence of an intramolecular H-bond between a CN and one-water molecule coordinated to Y can be noticed.
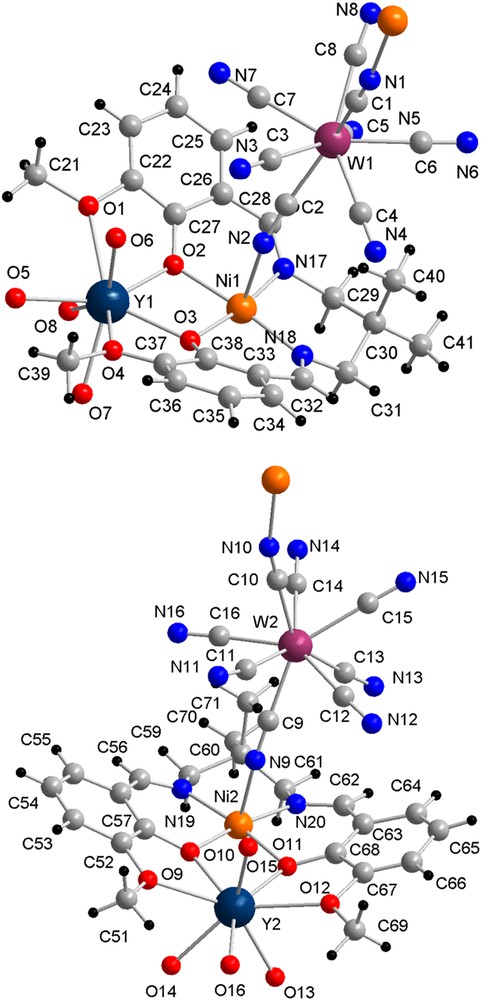
View of the two asymmetric fragments of 1 with their numbering scheme. Selected distances (Å) and angles (°): Ni1–N1, 2.06(2); Ni1–N2, 2.13(2); Ni1–N17, 2.04(1); Ni1–N18, 1.99(2); Ni1–O2, 2.02(2), Ni1–O3, 1.99(2); Ni2–N9, 2.12(2); Ni2–N10, 2.9(2); Ni2–N19, 1.99(2); Ni2–N20, 1.99(2), Ni2–O10, 2.02(1); Ni2–O11, 2.05(1); Y1⋯Ni1, 3.367(3); Y2⋯Ni2, 3.373(3); Y1–O1, 2.53(2); Y1–O2, 2.53(1); Y1–O3, 2.28(1); Y1–O4, 2.58(1); Y1–O(H2O), 2.29(1)–2.36(1); Y2–O9, 2.56(1); Y2–O10, 2.20(1); Y2–O11, 2.22(1); Y2–O12, 2.56(1), Y2–O(H2O), 2.34(1)–2.37(1); Ni1–N1–C1, 163(2); Ni1–N2–C2, 160(1); Ni2–N9–C9, 164(2); Ni2–N10–C10, 163(2).

View of the supramolecular organization of the trinulear {3d–4f–5d} units for compound 1 with the H2O molecules bridging the two chains; a selection of terminal H2O molecules H-bonded to the CN ligands is also depicted. Selected distances (Å): O20⋯N8, 2.91(3); O20⋯N12, 2.88(3); N3⋯O7, 2.85(2); N11⋯O14, 2.88(2).
The molecular structure of compound 2 is shown in Fig. 3. Interestingly, changing the Ln ion from Y to La leads to a different assembling scheme for the molecular building units. For 2 it consists in a hexanuclear aggregate involving two cyanometalate and two {LMe2NiLa} units. One of the {W(CN)8} units bridges the two {LMe2NiLa} moieties by coordination to the Ni ions in the same manner as for 1, and the second W unit is linked to one of the La ions by means of a CN ligand. The Ni ions are in an octahedral surrounding that comprises two N and two O atoms from LMe2 located in equatorial positions, whereas the axial positions are occupied by the N atom of the bridging cyanide and a H2O molecule. The two La ions display nine atoms in their coordination spheres with four O from the LMe2 ligand and five H2O for La2 whereas for La1 one of the H2O is replaced by the cyanide linkage with a W unit. All of the CN–Ni/La angles are close to linearity (170–173°). The crystal lattice also contains nine H2O molecules for each cluster leading to the formula [{LMe2NiLa(H2O)4.5}2{W(CN)8}2]·9H2O for 2. Several hydrogen bonds are established between H2O molecules and cyanide units (Fig. 3). The most relevant with respect to the subsequent magnetic studies are the H2O that bridge two W centers of adjacent aggregates (O20 and O7).

View of (top) the hexanuclear aggregate 2, (bottom) the hydrogen bonds established between 2 and H2O molecules. Selected interatomic distances (Å) and angles (°): Ni1–N1, 2.059(3); Ni1–O9, 2.191(3), Ni2–N2, 2.084(3); Ni2–O19, 2.015(4); La1–N9, 2.642(4); Ni1⋯La1, 5.5670(6); Ni2–La2, 3.6043(6); W1–C–N, 175.1(4)–179.5(5); W2–C–N, 172.9(4)–179.7(6); Ni1–N1–C1, 170.1(3); Ni2–N2–C2, 170.0(3); La1–N9–C9, 173.2(3); O7⋯N11, 2.684(8); O7⋯N8, 2.848(1); O16⋯N13, 2.832(5); O17⋯N14, 2.732(7); O20⋯N15, 2.769(7); O20⋯N4, 2.848(7); O21⋯N6, 2.873(7); O28⋯N5, 2.794(7); O31⋯N12, 2.761(6).
Stricto sensus, Y(III) is not a lanthanide ion, however, it is usually compared to La because these ions have the electronic configuration of the noble gases [Kr] and [Xe], respectively. Moreover, the chemical behavior of Y(III) ion is known to be similar to that of the Ln(III) ions and numerous examples of isostructural compounds have been reported [5,29,30]. For the two compounds reported here this isostructurality does not apply, at least as far as the supramolecular organization is concerned. Both 1 and 2 are made up by the assemblage of one binuclear {3d–4f}3+ cation and one {W(CN)8}3− anion, but, whereas the Y derivative consists in a chain organization, the La compound is an hexanuclear {3d–5d–4f} aggregate. A rational for this dissimilar association scheme might be found in the slightly larger ionic radii for La3+ (1.06 Å) compared to Y3+ (0.90 Å) that leads to a coordination sphere of the Ln ion for 2 and 1 of nine and eight, respectively. This may have an incidence onto the crystal packing and drive the supramolecular assembling scheme for the compounds.
The polyhedral shape around the W centers was ascertained by a continuous shape measure (CShM) analyses [31,32] carried out with SHAPE [33]. The knowledge of the shape of the paramagnetic {M(CN)8} units is of importance regarding the magnetic behavior because the actual geometry determines the spin density distribution and consequently the strength of the exchange interaction mediated by the bridging cyanide [34]. Analysis of the coordination polyhedra of the cyanometalate cores in compounds 1 and 2 shows that the geometry around all the W centers is close to square antiprism. The results of the SHAPE analysis have been tabulated in Table 1.
Results of the SHAPE analysis for the {W(CN)8} cores in compounds 1 and 2
[M(CN)8] | (SAPR-8)a | (DD-8)a | (JBTP-8)a | (SBTP-8)a |
W1(1) | 0.45124 | 1.82875 | 1.89915 | 23.99432 |
W2(1) | 0.55479 | 1.18088 | 2.30802 | 23.95997 |
W1(2) | 0.64908 | 1.34771 | 1.83738 | 24.65892 |
W2(2) | 0.35541 | 1.65086 | 2.34870 | 24.33542 |
a SAPR-8: D4d, square antiprism; DD-8: D2d, triangular dodecahedron; JBTP-8: C2, biaugmented trigonal prism J50; SBTP-8: C2v, Spherical biaugmented trigonal prism.
Magnetic properties. The magnetic behaviors for 1 and 2 were investigated on polycrystalline samples (crushed crystals) in the temperature domain 300–302 K with an applied field of H = 1000 Oe. The temperature dependence of the molar magnetic susceptibility, χM, for each compound is given as χMT plots in Fig. 4. For 1, the value of χMT at 300 K is 1.72 cm3 K mol−1, slightly larger than the expected value of 1.437 cm3 K mol−1 for one Ni ion (S = 1, g = 2.1) and one W ion (S = 1/2, g = 2) in the absence of exchange interaction. This value steadily increases as the temperature is lowered and reaches a maximum of 3.5 cm3 K mol−1 for 6 K, below which it sharply decreases to 0.7 cm3 K mol−1 at 2 K. The variation of χM with T shows also a maximum around 5 K. Such a behavior supports the anticipated ferromagnetic Ni–W interactions [34] within the chain but also highlights in the low temperature domain the occurrence of antiferromagnetic interaction among the chains. This is supported by the field dependence of the magnetization recorded at 2 K (Fig. 5) that tends to a saturation value of 3 μB but exhibits a meta-magnetic like behavior evidenced by a slope change at low fields. The weak antiferromagnetic interaction between chains is attributed to the H2O molecule hydrogen bonded between the W centers of two adjacent chains.

Experimental ( for 1; ○ for 2) and calculated (−for 2) χMT versus T behaviors for 1 and 2.

Field dependence of the magnetization for 1 and 2 recorded at 2 K.
The χMT plot for 2 displays a behavior similar to that for 1. The value for χMT at 300 K is 3.45 cm3 K mol−1, slightly larger than the 2.95 cm3 K mol−1 anticipated for two Ni and two W ions without interaction. This value steadily increases when the temperature is lowered and reaches a broad maximum of 3.93 cm3 K mol−1 for 25 K. The χMT product then decreases with further lowering of the temperature and attains a value of 2.04 cm3 K mol−1 at 2 K. Here again, ferromagnetic and antiferromagnetic interactions are operative. For compound 2, several origins can be invoked to account for the antiferromagnetic contribution. One is the linkages between {W(CN)8} moieties realized by H2O molecules hydrogen bonded to the cyanide ligands as evidenced from the crystal structure. A second contribution comes from the superexchange interaction mediated by the diamagnetic metal ion (also called next-neighbor interaction) [1] that is known to be rather efficient for 4f ions [1,5].
A suitable theoretical model for a quantitative analysis of the magnetic behavior for a ferromagnetic chain such as in 1 is not available. For compound 2, the magnetic behavior has been analyzed considering the exchange coupling between two Ni with a W center, the second W having a paramagnetic contribution. The antiferromagnetic contributions which are anticipated to be weak have been considered within the mean-field approximation (zJ′). The interaction parameter between WV and NiII was determined from least-squares fittings of a theoretical expression for χMT [34] deduced from the spin Hamiltonian H = −J(SNi1·SW + SNi2·SW) + β[gNi(SNi1 + SNi2) + gWSW]H and including the paramagnetic contribution of one WV ion. Best fit to the experimental data of 2 was obtained for J = 20.8 ± 0.4 cm−1, zJ′ = −1.35 ± 0.01 cm−1, gNi = 2.29, and gW = 2 (fixed). The strength of the ferromagnetic Ni–W interaction found here is in good agreement with the values reported for related cyanide bridged systems. Moreover, this value well fits with the value anticipated for square antiprismatic {W(CN)8}3− [34,35].
3 Concluding remarks
Two original {3d–5d–4f} heterometallic architectures have been obtained by assembling bimetallic {Ni–Ln}3+ and {W(CN)8}3− units. The difference of supramolecular organization for YIII and LaIII, i.e. chain compound and hexanuclear aggregate, respectively, is rather unexpected. For both the compounds ferromagnetic Ni–W interactions are operative, the parameterization leads to J = 20 cm−1 for 2. It is interesting to note that this value is in good agreement with the exchange coupling anticipated for {W(CN)8}3− in square antiprism geometry (SAP) with NiII ions. An investigation of the incidence of the geometry of a d1 {M(CN)8} unit on the spin distribution has shown that the spin population on the cyanide N atom for the SAP geometry should lead to an exchange coupling strength intermediate between those of the two CN sites of a dodecahedral geometry [34]. This is now experimentally confirmed by the exchange parameter of compound 2, J = 20 cm−1, that is intermediate between the reported 14 and 37 cm−1 for bridging cyanides located, respectively, on sites A and B of {W(CN)8}3− in DD geometry [34].
The magnetic behaviors of compounds 1 and 2 also revealed that crystallization lattice H2O molecules hydrogen bonded to the W cyanide ligands and bridging two such units mediate antiferromagnetic interactions among the supramolecular architectures. Work is in progress to extend this approach towards heterotrimetallic {3d–5d–4f} compounds with other 3d ions and paramagnetic Ln ions.
4 Experimental section
The compounds Cs3{W(CN)8} [36] and {LMe2NiLn(NO3)3} [37] have been prepared by reported procedures. IR spectra were recorded with a Perkin–Elmer Spectrum 100 FT-IR spectrometer. Elemental analyses were performed using a Perkin–Elmer 2400 series II instrument. Magnetic measurements down to 2 K were carried out with a Quantum Design MPMS-5S SQUID susceptometer. The molar susceptibility was corrected for sample holder and for the diamagnetic contribution of all the atoms by using Pascal's tables [38,39].
4.1 Synthesis of [{LMe2NiY(H2O)4}{W(CN)8}]∞·9.5H2O, 1
A solution of [LMe2Ni(H2O)2Y(NO3)3] (38 mg, 0.05 mmol) in water (6 mL) was layered with a solution of Cs3[W(CN)8] (43 mg, 0.05 mmol) in water (6 mL). Green crystals formed after 1 day. These were isolated and washed with H2O and THF. Yield: 28 mg (55%). Anal. calcd for C29H37N10O10.5Ni1Y1W1: C, 33.98; H, 3.63; N, 13.66. Found: C, 34.15; H, 3.30; N, 13.10. IR (υCN): 2165, 2147 cm−1.
4.2 Synthesis of [{LMe2NiLa(H2O)4.5}2{W(CN)8}2]·9H2O, 2
A solution of [LMe2Ni(H2O)2La(NO3)3] (41 mg, 0.05 mmol) in H2O (4 mL) was layered with a solution of Cs3[W(CN)8] (43 mg, 0.05 mmol) in H2O (6 mL). Green crystals formed after 2 days. These were isolated and washed with H2O and THF. Yield: 20 mg (35%). Anal. calcd for C58H90N20O29Ni2La2W2: C, 30.36; H, 3.95; N, 12.21. Found: C, 30.57; H, 3.62; N, 11.81. IR (ATR, υCN): 2177, 2161, 2150, 2114 cm−1.
4.3 Crystallographic studies
Intensity data for compound 1 were collected at 180 K on an Xcalibur Oxford Diffraction diffractometer using a graphite-monochromated Mo Kα radiation source. C29H32N10NiO8WY, 9.5(H2O); M = 1151.25, planar pale brown crystal, 0.02 × 0.15 × 0.30, orthorhombic, space group Pca21, a = 24.2341(9), b = 10.4181(5), c = 35.3851(18) Å, V = 8933.8(7) Å3, Z = 8, d = 1.712, μ(Mo Kα) = 4.352 mm−1. 2θmax = 58.2°. 508 parameters, 77,876 reflections measured, 23,824 unique (Rint = 0.13), 6027 reflections used in the calculations [I > 3σ], R = 0.0326, wR = 0.0429, residual electron density = −0.64/1.53 (e/A−3).
Data for compound 2 were collected at 180 K on an Apex2 Bruker diffractometer. C58H70La2N20Ni2O19W2, 16(H2O); M = 2402.49, planar brown crystal, 0.05 × 0.20 × 0.25, monoclinic, space group P21/n, a = 18.2294(6), b = 27.1887(10), c = 18.6077(7) Å, β = 90.451(1)°, V = 9222.3(6) Å3, Z = 4, d = 1.730, μ(Mo Kα) = 3.877 mm−1, 2θmax = 69.00°, 1072 parameters, 158,665 reflections measured, 39,032 unique (Rint = 0.07), 19,957 reflections used in the calculations [I > 3σ], R = 0.0315, wR = 0.0356, residual electron density = −1.60/3.25 (e/A−3).
The structures were solved by direct methods using SIR92, and refined by means of least-squares procedures on F using the programs of the PC version of CRYSTALS. Atomic scattering factors were taken from the International Tables for X-ray Crystallography. For compound 2, all non-hydrogen atoms were refined anisotropically. For complex 1, only tungsten, Y and nickel atoms were refined anisotropically, due to the lack of reflexions and high number of parameters.
5 Supplementary material
The supplementary crystallographic material (cif) has been deposited to the Cambridge Crystallographic Data Center with reference CCDC 682938 (1) and CCDC 682937 (2) and can be obtained free of charge via http://www.ccdc.cam.ac.uk/conts/retrieving.html.