1 Introduction
Chiral shape-persistent macrocycles are of great interest because their unique structures provide potential utilities for chirality sensing, chiral guest recognition, chiral separation, as well as ligands for asymmetric catalysts [1–4]. When the chirality of the molecules is controllable by external stimuli, they would be utilized as molecular devices for a chirality sensing and a chiroptical memory [5,6]. Recently, we have studied phenylacetylene macrocycles (PAMs) with novel structures such as cyclic [n]paraphenyleneacetylene [7], cyclic [n]metaphenyleneacetylene [8,15a] and their related compounds [9]. The syntheses were carried out using the McMurry coupling of appropriate dialdehydes as a key step [10]. In the course of the studies, we have designed and synthesized macrocyclic cyclophanes 1 and 2 composed of two binaphthol and biphenol units (Scheme 1) [11,12]. The biarylonophanes have two possible diastereomers, achiral (meso) and chiral (racemic) forms owing to two asymmetric axes of the biaryl units (Fig. 1). The considerably high energy of racemization barrier of a binaphthol skeleton (37.8 kcal mol−1) [13] enables the obtaining of the compound 1 in enantiomerically pure forms. On the other hand, the energy barrier of interconversion between two diastereomers of 2 would depend upon the bulkiness of substituents at 2,2′-positions of diphenyl units. Actually, a tetramethoxy derivative 2b exists in an equilibrium mixture between meso and racemic forms in a chloroform solution; 1H NMR spectrum at −50 °C indicated the ratio between major and minor isomers to be ca. 5:1. Variable-temperature (VT) NMR experiments allowed the evaluation of the activation energy for the interconversion as ca. 12 kcal mol−1. The crystal analysis reveals that 2b adopts the meso-form in the crystals. Combined with the theoretical predictions, the meso-form should be predominant in solution. The biphenylonophane framework of 2 seems unsatisfactory to our purpose for the construction of molecular devices such as a chirality sensing and a chiroptical memory.
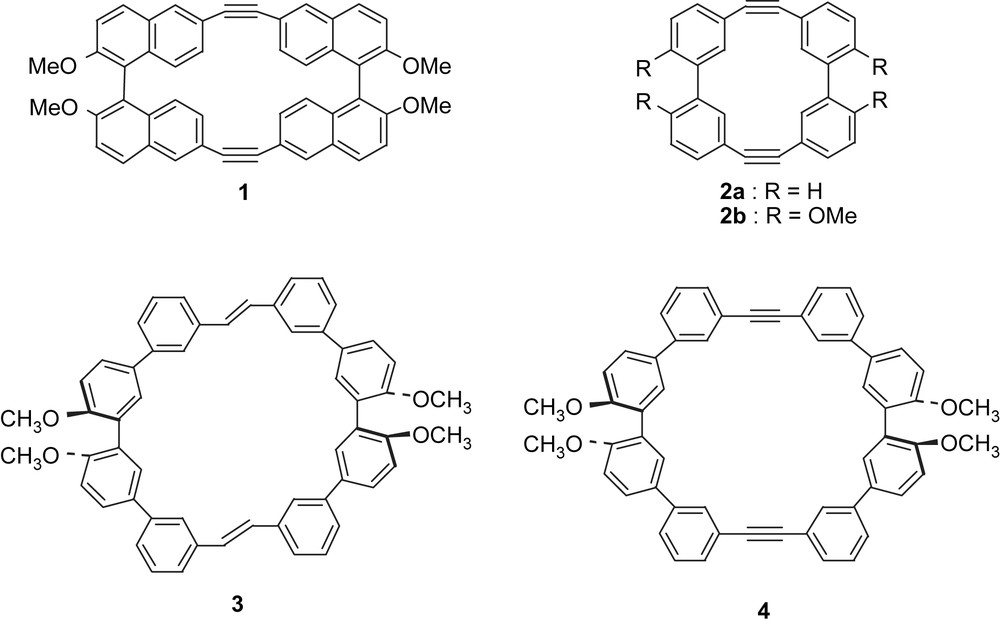
Biarylonophanes 1 and 2, and macrocyclic diene 3 and diyne 4 bearing two quaterphenyl units.
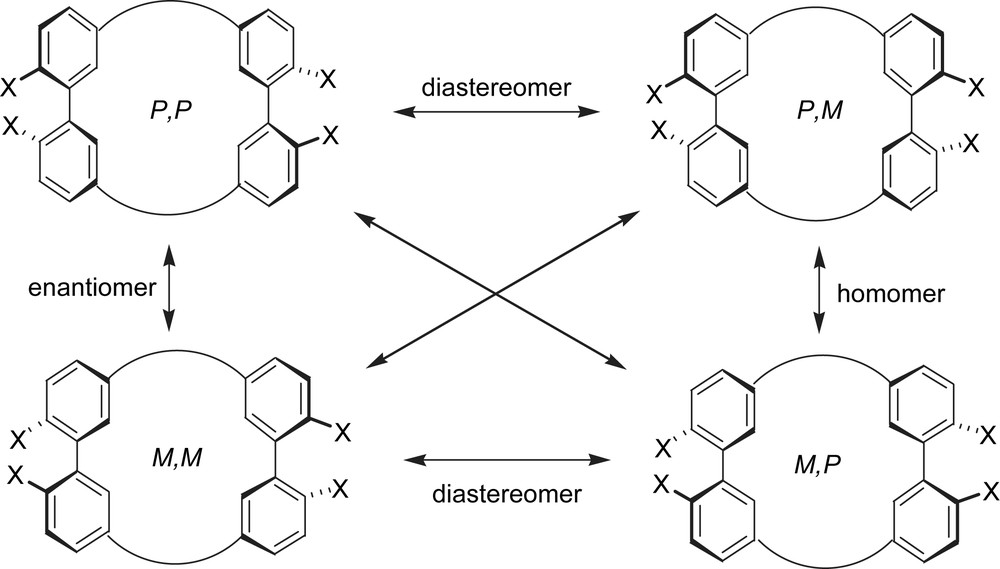
Relationship among stereoisomers of a molecule with two biaryl units.
In this context, we have newly designed macrocyclic diene 3 and diyne 4 having two quaterphenyl units. Theoretical calculations (PM3) [14] predict that chiral-forms 3a and 4a (Fig. 2) are about 1 kcal mol−1 lower than achiral (meso)-forms 3b and 4b in the heat of formation. The relatively large differences suggest that these macrocycles should predominantly exist as the chiral-forms. On the other hand, racemization barrier through their diastereomers would be relatively low, because two oxygen functionalities at 2,2′-positions are not so bulk. Here we report the synthesis, properties and the molecular structure of 4. Moreover, methyl groups of the diene 3 can be replaced with chiral camphorsulfonyl groups, though similar reaction of 4 resulted in failure. The chiroptical properties of 3 as a potential segment for chiral sensor are also discussed.
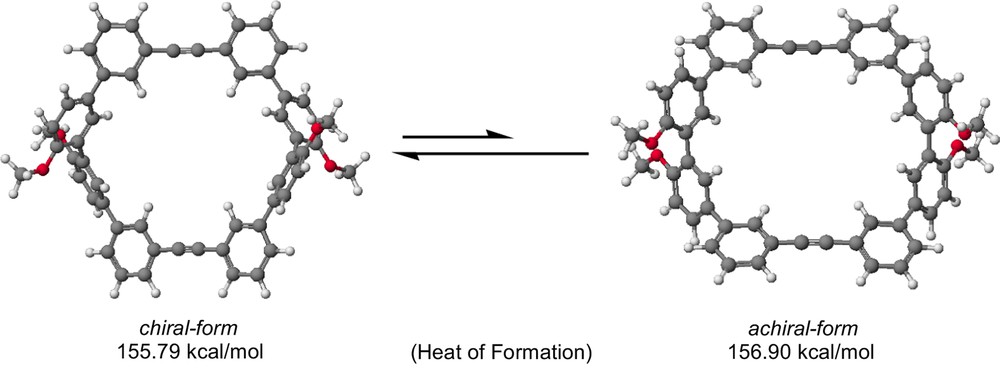
Predicted molecular structures of chiral and achiral conformers, 4a and 4b.
2 Results and discussion
2.1 Synthesis
The synthesis of 3 and 4 is outlined in Scheme 2. The Suzuki–Miyaura coupling of 2,2′-dimethoxy-5,5′-bromobiphenyl 5 with commercially available 3-formylbenzene boronic acid afforded diformylquaterphenyl 6 (66%). Treatment of 6 with TiCl4–Zn in DME–toluene (3:1) afforded the cyclophane diene 3 (37%) together with a corresponding trimer with a ratio of ca. 10:1 (from 1H NMR). If the reaction was performed in DME only, the yield was reduced to 19%. The relatively high field shift of etheno-protons of 3 (7.19 ppm) is indicative of the Z-configuration in comparison with the corresponding protons of (E)- and (Z)-stilbenes. We have previously reported that the presence of toluene as a co-solvent gives rise to highly E-selective double bond formation in the McMurry reaction [15]. The high E-selectivity seems to play a key role for the relatively high yield. The dimer 3 can be purified with gel permeation chromatography (GPC). The dimeric structure was established on the basis of NMR spectroscopic and mass spectrometric analyses. Bromination–dehydrobromination of 3 led to the diyne 4 (80%). The macrocycles were obtained as fairly stable colorless prisms.

Synthesis of 3 and 4.
2.2 Spectral properties
1H and 13C NMR spectra of the cyclophanes 3 and 4 exhibit expected simple pattern in agreement with the high symmetry of the molecule at 30 °C. In contrast to cyclophane 1b, VT-NMR spectra of the macrocycles show little change down to −50 °C. The results clearly indicate that the interconversion between the diastereomers occurs with relatively low energy barrier (<10 kcal mol−1). The protons at 8 positions of quaterphenyl units of 3 and 4 (3 for 7.77 ppm and 4 for 7.67) appear in low fields in the 1H NMR spectra, which would be due to the anisotropy effect of olefin and acetylene bonds. The sp carbons of 4 were resonated at 89.3 ppm, which is almost identical to corresponding carbons of diphenylacetylene (89.4 ppm). The results indicate the less strain nature of the compound.
UV–vis and fluorescence spectra in dichloromethane of 3 and 4 are shown in Fig. 3. Both compounds exhibit intense absorption bands at 278 nm and 272 nm, respectively. The molar absorptivity of 4 at λmax is about 1.5 times as high as that of 3, probably due to highly planar conformation of the diyne. The diene 3 has a shoulder band at 334 nm. The UV–vis spectra of 3 were measured under several concentrations (1.28 × 10−6–6.38 × 10−4 mol l−1). The shapes and intensities of the absorption bands including the shoulder band only show little changes. The results indicate that the shoulder band seems not an aggregation band. The cyclophanes 3 and 4 exhibit emission bands at 380 nm and 361 nm, respectively. The shoulder band of 3 would be due to the HOMO–LUMO transition. But, the transition of 4 would be submerged by the intense absorption band at 270 nm. The exact values of the Stokes' shift cannot be evaluated.
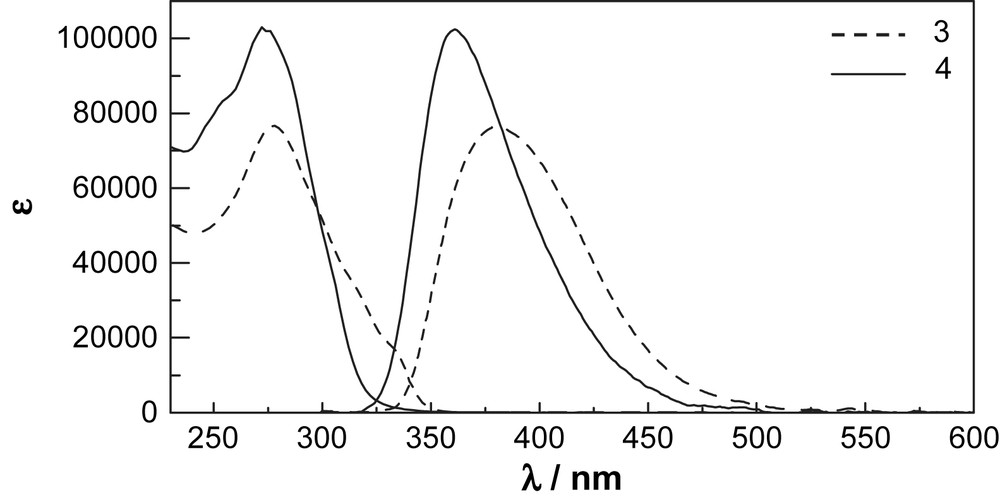
Absorption and emission spectra of 3 and 4 in CH2Cl2. Emission spectra were obtained upon excitation at absorption maximum, and the intensities are normalized with those of absorption spectra.
2.3 Molecular structure
A chloroform–hexane solution provided moderately good single crystals of the cyclophane-diyne 4 by a vapor-diffusion method. The crystal contains three chloroform molecules per single macrocyclic molecule. During the sampling operation of the single crystal of 4 for X-ray crystallography, the solvent molecules desorb so rapidly from the crystal, leading to the slight loss of transparency of the crystal. The X-ray data quality of 4 was not satisfactory due to the reduction of the single crystallinity (the R1 value is 0.140). Fig. 4 shows ORTEP drawings: (a) top view, (b) side view, and (c) a molecular packing. The molecular structure of 4 is quite similar to the one predicted by PM3 calculations; it possesses a chiral conformation and almost linear acetylenic bonds (averaged bond angle of acetylenic carbons is 179°). The aromatic rings of quaterphenyl units have an alternating up-down arrangement. The averaged dihedral angle between the planes of aromatic rings bearing a methoxy group (∠AH and ∠DE) is 77.2°, while that of other aromatic rings (∠AB, ∠CD, ∠EF, and ∠GH) is 35.2°. The congestion of methoxy groups increases the molecular twisting. The dihedral angles between the aromatic rings of diphenylacetylene moieties (∠BC and ∠FG) are smaller than 30°, which indicates the good planarity of the segments. The crystal has P-1 space group; therefore, it includes both enantiomers. Each enantiomer forms a layer structure that gives rise to columns with channels (Fig. 4c). Two chloroform molecules are situated in the sizable and chiral cavity. The third chloroform molecule occupies the interspace of four columns. The preparation of co-crystals with chiral molecules is now in progress.

ORTEP plots (50% probability): (a) top view and (b) side view. Third chloroform molecule is omitted for clarity. (c) A molecular packing of 4.
2.4 Potential utility as a chiral sensor
The crystallographic analysis of 4 indicates that the chiral conformer is more stable in the crystals. The configurationally flexible biphenyl chromophore for which induction of axial chirality by interaction with chiral auxiliaries is well documented and has been exploited in molecular recognition studies [16]. To explore potential utilities of the macrocycles as a chiral sensor or a memory device, we introduce a chiral auxiliary, camphorsulfonyl (CS) group, in place of the methyl groups.
Demethylation of 3 with BBr3 in dichloromethane at room temperature successfully afforded a tetraphenol 7 in high yield (88%). Although 7 is poorly soluble in organic solvents, treatment with chiral camphorsulfonyl chloride ((+)- or (−)-CSCl) in the presence of triethylamine in THF led to corresponding tetra-camphorsulfonyl, [(+)- or (−)-CS]4-macrocycles, (+)-8 and (−)-8, in moderate yields (Scheme 3). Unfortunately, demethylation of the diyne 4 under several conditions resulted in failure. The acetylenic bonds of 4 are susceptible to the reaction conditions.
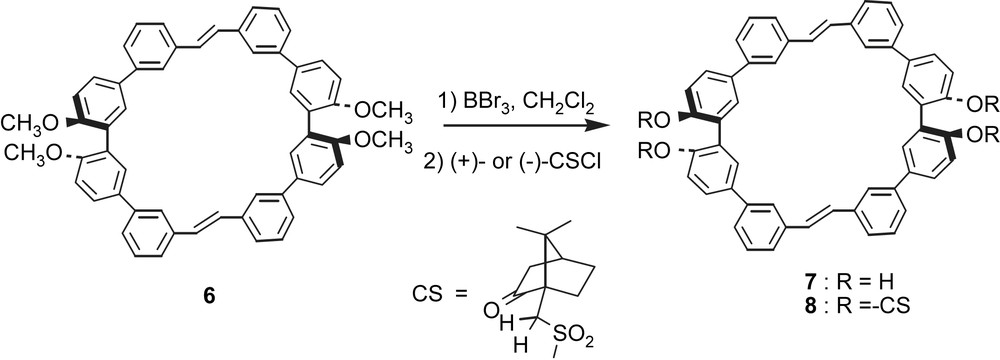
Preparation of tetra-camphorsulfonylated macrocycle 8.
There are three possible diastereomers, [(+)-CS]4-PP, [(+)-CS]4-MM and [(+)-CS]4-PM, in (+)-8. These diastereomers are distinguishable and separable under appropriate conditions. 1H NMR spectrum of 8 exhibits two sets of unequivalent signals assignable to α-protons of camphorsulfonyl groups (Fig. 5). VT-NMR spectra in toluene-d8 did not show coalescence of the signals even at 100 °C, which suggests that the activation energy of interconversion between diastereomers is relatively high (>19 kcal mol−1). The diastereo excess (d.e.) can be estimated to be ca. 10% from the integration of the signals. Despite the small d.e., the well-defined circular dichroism (CD) spectrum was observed (λext (THF); Δɛ = +9.9, 266 nm). Because the spectral curve is apparently different from that of (+)-camphorsulfonic acid (Δɛ = ±1.535, 290 nm) [17], the CD absorption is attributed to chirality of the macrocycle moiety. The two diastereomers can be separated with a chiral HPLC (CHIRALCEL OD, Daicel Co. Flow rate 1.0 ml/min, Hexane:EtOH = 1:1, 35 °C). The CD absorption (270 nm) of two elusions shows almost mirror images, which indicate that two diastereomers, [(+)-CS]4-PP and [(+)-CS]4-MM, were separated under the conditions (Fig. 6a). The CD curve of a separated diastereomer, [(+)-CS]4-8, decreases with time even at 25 °C (Fig. 6b). The half-life period is estimated to be about 2.5 h from the spectral change, which gives the activation energy to be ca. 23.0 kcal mol−1. These results indicate the potential ability of the macrocylic system as a chiral sensor. The intensity at ca. 260 nm decreases with time, however; the intensity at 325 nm appears unchanged with time. The weak CD band at 325 nm would not come from the cyclophane framework, but the biaryl moieties with chiral camphorsulfonyl groups. Theoretical studies to determine the absolute configuration of diastereomers will be reported in due course [18,19].
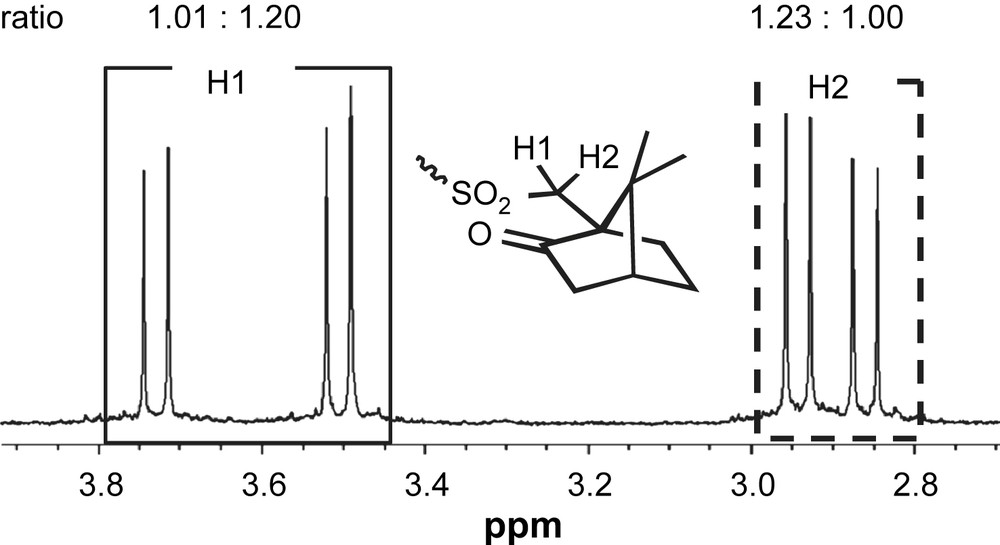
A partial NMR spectrum of (+)-8.
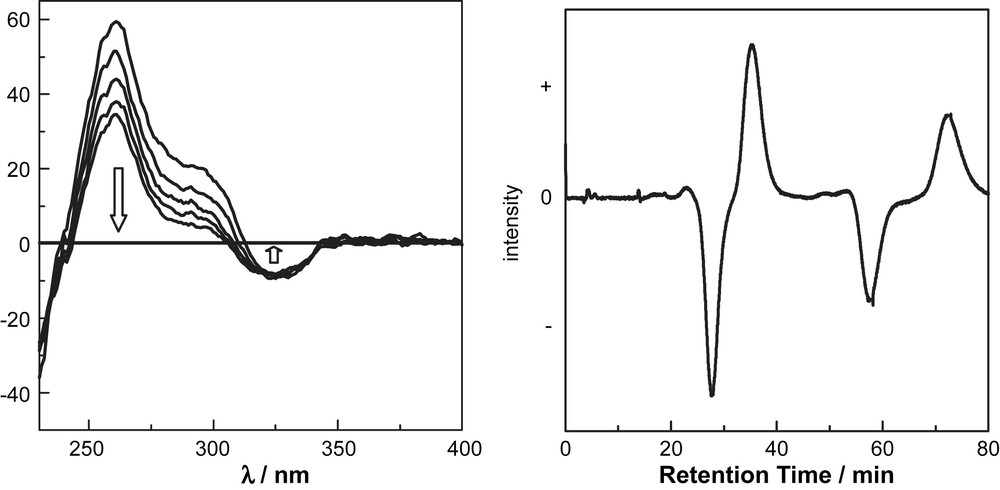
(a) CD spectral changes of a diastereomer (the first eluted fraction) of (+)-8 in THF with each 30 min at 25 °C. (b) Chromatogram for the diastereomeric separation of (+)-8. CD detection (λ = 275 nm). Conditions: CHIRALCEL OD, flow rate 1.0 ml/min, Hexane:EtOH = 1:1 at 35 °C.
3 Experimental
3.1 General remarks
Melting points were recorded on a Yanaco MP 500D apparatus and uncorrected. FAB, EI and ESI mass spectra were measured on JEOL JMS-SX102, Shimadzu GCMS QP-5050A, and Applied Biosystems QSTAR, respectively. 1H NMR spectra (tetramethylsilane; 0 ppm as an internal standard) and 13C NMR spectra (CDCl3; 77.0 ppm as an internal standard) were recorded on JEOL lambda-500, JEOL JNM-GSX-400, and JEOL EX-270 apparatus. The chemical shifts are given in ppm. IR spectra were obtained from a JASCO FT-IR-460K2 spectrometer. Electronic (UV–vis) and circular dichroism (CD) spectra were obtained on Jasco V-570 and J-725 spectrophotometers, respectively. Column chromatography was performed with Merck Kiesel-gel 60.
3.2 Materials
All reagents of commercial quality were used as supplied unless otherwise stated; tetrahydrofuran (THF) and 1,2-dimethoxyethane (DME) were dried where necessary using standard procedures.
3.2.1 5,5′-bis(3-formylphenyl)-2,2′-dimethoxybiphenyl 5
A 100 ml of two-necked round-bottomed flask equipped with a reflux condenser bearing a nitrogen inlet tube and septum was charged with 5,5′-dibromo-2,2′-dimethoxybiphenyl (372 mg, 1 mmol), 3-formylphenylboronic acid (450 mg, 3 mmol), Na2CO3 (636 mg, 6 mmol), Pd(PPh3)4 (173 mg, 0.15 mmol) and a magnetic stirring bar. DME (10 ml) and water (5 ml) were added, and the mixture was heated at reflux under a nitrogen atmosphere for 17 h. The reaction mixture was cooled to room temperature, and diluted with 20 ml of water, and extracted with CH2Cl2 (20 ml × 3). The separated organic layers were combined, washed with water and brine, and dried over Na2SO4. After filtration, the filtrate was concentrated under reduced pressure. The residue was purified by column chromatography on silica gel (30 g), eluted by n-hexane-CH2Cl2 (v/v = 1/1) to give 5 (280 mg: 66%) as a colorless solid.
5. Colorless fine solid; mp 176.1–176.8 °C; 1H NMR (270 MHz, CDCl3) δ 10.1 (s, 2H), 8.09 (t, J = 1.5 Hz, 2H), 7.86 (dt, J = 7.6, 2.0 Hz, 2H), 7.79 (dt, J = 7.6, 1.5 Hz, 2H), 7.63 (dd, J = 8.6, 2.5 Hz, 2H), 7.59–7.53 (m, 4H), 7.09 (d, J = 8.6 Hz, 2H), 3.84 (s, 6H); 13C NMR (67.8 MHz, CDCl3) δ 192.4, 157.3, 141.6, 136.9, 132.7, 131.9, 130.2, 1290.4, 128.1, 128.0, 127.7, 127.5, 111.6, 55.9; MS (EI, 70 eV) m/z (rel intensity) 422 (100, M+), 364 (9), 336 (8); IR (KBr, cm−1) 2995 (w), 2950 (w), 2828 (w), 2727 (w), 1698 (s), 1603 (s), 1505 (s), 1479 (m), 1438 (m), 1277 (s), 1182 (s), 1023 (m), 800 (s), 693 (m), 651 (m), 576 (m); Anal. Found: C, 79.21; H, 5.23. Calcd. for C28H22O4: C, 79.60; H, 5.25.
3.2.2 Macrocyclic diene 3
A 100 ml of well-dried, three-necked round-bottomed flask equipped with a reflux condenser bearing a nitrogen inlet tube and a pressure-equalizing funnel was charged with zinc powder (1.309 g, 20 mmol), copper(I) iodide (136 mg, 0.7 mmol) and a magnetic stirring bar. Freshly distilled DME (15 ml) and dried toluene (5 ml) were added through a rubber septum by syringes, followed by the addition of TiCl4 (1.1 ml). The mixture was heated to reflux for 3 h under a nitrogen atmosphere and cooled to room temperature. A solution of dialdehyde 5 (310 mg, 0.73 mmol) in DME (15 ml) was added dropwise through the dropping funnel over 30 min at room temperature. The mixture was stirred overnight at the temperature and then refluxed for 8 h. The reaction mixture was passed through a short alumina column chromatography (50 g) with CH2Cl2. The filtrate was concentrated and residue was purified by column chromatography on silica gel (50 g) eluted by CH2Cl2 to give a macrocycle 3 (106 mg, 37%).
3: White powder; mp 333 °C (decomp); 1H NMR (500 MHz, CDCl3) δ 7.68 (s, 4H), 7.54 (dd, J = 8.5, 2.4 Hz, 4H), 7.50 (d, J = 2.4 Hz, 4H), 7.48–7.39 (m, 12H), 7.18 (s, 4H), 7.03 (d, J = 8.5 Hz, 4H), 3.76 (s, 12H); 13C NMR (67.8 MHz, CDCl3) δ 156.7, 141.6, 137.8, 133.4, 130.5, 129.1, 128.9, 127.9, 127.4, 126.4, 125.4, 124.8, 111.3, 55.8; MS (EI, 70 eV) m/z (rel intensity) 780 (100, M+), 390 (52, M2+); UV–vis (CH2Cl2) λmax (log ɛ) 278 nm (4.88), 334 nm (4.22); Fluorescence (CH2Cl2) λmax 380 nm; IR (KBr, cm−1) 3023 (w), 2952 (w), 2834 (w), 1600 (s), 1499 (s), 1252 (s), 1028 (m), 799 (m); Anal. Found: C, 85.94; H, 5.53. Calcd. for C56H44O4: C, 86.13; H, 5.68.
3.2.3 Macrocyclic diyne 4
The diene 3 (96 mg, 0.12 mol) was dissolved in chloroform (15 ml) in a 50 ml of round-bottomed flask equipped with a calcium chloride tube. A few drops of bromine (ca. 100 mg) were added and stirred at room temperature for 5 min. The solvent and bromine were removed under reduced pressure and dried in vacuum to give tetrabromide as pale yellow solid. Without further purification, the solid was dissolved in 15 ml of THF. To the solution was added potassium t-butoxide (275 mg, 2.45 mmol) and stirred at room temperature overnight. After quenching with water, the reaction mixture was extracted with CH2Cl2 (20 ml), and the organic layer was washed with water and brine, and dried over Na2SO4. After filtering the dry agent, the solvent was removed by evaporator. The residue was purified by column chromatography on silica gel (30 g) eluted by n-hexane-CH2Cl2 (v/v = 1/1) to give 4 (76 mg: 80%) as a colorless solid.
4. White powder; mp 290 °C (decomp); 1H NMR (270 MHz, CDCl3) δ 7.77 (s, 4H), 7.63–7.37 (m, 20H), 7.03 (d, J = 8.2 Hz, 4H), 3.81 (s, 12H); 13C NMR (67.8 MHz, CDCl3) δ 156.9, 140.8, 132.6, 130.2, 130.0, 129.5, 128.6, 128.3, 127.1, 126.6, 123.5, 111.2, 89.2, 55.9; MS (EI, 70 eV) m/z = 777 (100, [M + H]+), 776 (97, M+), 428 (55), 388 (M2+); UV–vis (CH2Cl2) λmax (log ɛ) 272 nm (5.01); Fluorescence (CH2Cl2) λmax 361 nm; IR (KBr, cm−1) 2793 (w), 2834 (w), 1601 (s), 1500 (s), 1254 (s), 1029 (m), 795 (m); Anal. Found: C, 80.85; H, 4.95. Calcd. for C56H40O4·0.5CHCl3: C, 81.11; H, 4.88.
3.2.4 Tetrahydroxyl 7
Diene (45 mg, 0.058 mmol) 3 was dissolved with dichloromethane (10 ml) in a 50 ml of round-bottomed flask equipped with a calcium chloride tube. To the solution was added BBr3 (64 mg, 0.25 mmol) and the mixture was stirred for 24 h at room temperature. The reaction mixture was quenched with water (10 ml), and the separated aqueous layer was extracted with dichloromethane (10 ml). The combined organic layer was washed with brine and dried over anhydrous Na2SO4. After removing the dry agent by filtration, the filtrate was evaporated to give sparingly soluble white solid (36 mg: 88%).
3.2.5 Tetra-(+)-camphorsulfonyloxy-diene 8
In a 100 ml of two-necked round-bottomed flask equipped with a reflux condenser and a nitrogen inlet tube, a suspension of tetrahydroxy-diene 7 (37 mg, 0.052 mmol), camphorsulfonyl chloride (657 mg, 2.62 mmol), THF (25 ml) and triethylamine (1 ml) was heated at reflux with stirring for 17 h under N2. After cooling at room temperature, the reaction mixture was quenched with water (10 ml) and extracted with ethyl acetate (10 ml × 3). The separated organic layer was washed with water and brine, and dried over anhydrous Na2SO4. After removing the dry agent by filtration, the filtrate was evaporated. The residue was diluted with ethanol to precipitate white solid. The filtered solid was purified by GPC to give (+)-8 as white solid (40 mg, 48%). Similar procedure afforded (−)-8 as white solid (9 mg, 14%). 8; white powder; mp 211.5–212.3 °C; MS (FAB) m/z 1582 [(M + 2H)+]; HRMS(ESI) Found: m/z C92H92O16NaS4 1603.5234; Calcd for C92H92O16NaS4 [M+], 1603.5160; 1 H NMR (500 MHz, toluene-d8) δ 7.92–7.89 (4 peaks, 4H), 7.75–7.64 (4 peaks, 4H), 7.53–7.51 (2 peaks, 4H), 7.39–7.22 (m, 16H), 6.89 (s, 4H), 3.75–3.49 (4 peaks, 4H), 2.96–2.85 (4 peaks, 4H), 2.35–2.22 (m, 4H), 1.92–1.82 (m, 4H), 1.43–1.34 (m, 12H), 0.84–0.78 (m, 8H), 0.86–0.72 (2 peaks, 12 H), 0.43–0.42 (2 peaks, 12H); UV–vis (THF) λmax (log ɛ) 263 nm (4.96), 311 nm (4.66), 334 nm (4.32); (+)-8, CD (THF) λext (Δɛ) 243 nm (−3.1), 266 nm (+9.9), 299 (+2.2), 329 (−1.2); (−)-8, CD (THF) λext (Δɛ) 242 nm (+3.4), 263 nm (−7.8), 302 (−2.9), 321 (+1.1).
3.2.6 X-ray crystallographic analysis of 4
Intensity data were collected on a Rigaku/MSC Mercury diffractometer CCD at −123 °C. The structure was solved with direct method on F2 with SHELXS-97. A total of 11,879 reflections were collected, 5561 unique reflections were measured and used in the refinement, 649 parameters, R1 = 0.140, Rw = 0.444 for 1687 reflections with I > 2σ(I), GOF = 1.31, max. peak in final diff. Map 0.78 eÅ−3. All non-hydrogen atoms were refined anisotropically. Hydrogen atoms were placed geometrically and refined by using a rigid model. Crystal data for 4·3(CHCl3), M = 1135.06; triclinic, P-1(#2), a = 12.27(9) Å, b = 14.76(4) Å, c = 15.40(5) Å, α = 92.0(2)°, β = 103.3(2)°, γ = 102.5(2)°, V = 2640(2) Å3, Z = 2, ρ = 1.428 g cm−3. CCDC 701618. The supplementary material has been sent to the Cambridge Crystallographic Data Centre, 12 Union Road, Cambridge CB21EZ (fax: +44 1223 336 033; e-mail: deposit@ccdc.cam.ac.uk).
Acknowledgements
This work was supported by Grant-in-Aids for Scientific Research (B) (No. 16350073) and for Exploratory Research (18655016) from the Ministry of Education, Culture, Sports, Science and Technology (Japan). We thank Prof. S. Kaizaki (Osaka Univ.) for measurements of the CD spectra.