1 Introduction
Nonlinear optical (NLO) and electro-optical (EO) properties of π-conjugated systems have been the subject of intense interest during the past several decades [1]. This field has been fuelled by the large number of potential applications for conjugated materials in electronic and electro-optical devices. The π-conjugated systems based on the alkyne functionality have long been a cornerstone for the formation of semiconducting materials since the monumental discoveries of highly conductive polyacetylene [2] and the topochemical solid-state polymerization of diacetylenes [3]. On the other hand, advances in alkyne chemistry are appearing at an astounding rate, and acetylenes now constitute a principal class of compounds nearly in all areas of chemistry; including synthetic organic, material, natural product and biochemistry [4].
Structural rigidity and electronic communication are the hallmarks of the acetylene functionality [4]. These attributes make alkynes a highly versatile component for conjugated scaffolds of conducting materials such as polyacetylenes 1, arylacetylene macrocycles 2 [5] and arylene ethynylene framework 3 [6] (Fig. 1). The successful development of these materials in functional devices will be facilitated by a richer understanding of the key aspects of their synthesis, electronic structure, and special properties, including film formation [4]. The fruits of these efforts include synthetic polymers with programmed solid-state organization and transition metal acetylides 4 (Fig. 1) [7]. Poly arylene ethynylene (PAE) structures incorporating silole subunits 5 (Fig. 1) provide yet another appealing avenue to these materials. Because of the current vast body of work on conjugated alkynes, in this brief review we draw largely upon the silole work from our own lab and will briefly discuss related compounds with well-defined extended conjugation.

Examples of acetylene based π-conjugated semiconducting materials.
2 Silole-containing polymers and chromophores
The chemistry of silole based π-conjugated systems has continued to receive much attention due to their potential as conducting materials for novel applications such as light-emitting devices [8], nitroaromatic sensors [9] and biosensors [10]. The unique electronic features of silole ring arise from its low-lying LUMO, which is substantially different from cyclopentadiene and other heterocycles. This particularly low-lying LUMO is due to the σ∗–π∗ conjugation between the σ∗ orbital of exocyclic Si–R bond and the π∗ orbital of the butadiene moiety on the ring [11]. Structural rigidity and electronic communication of the alkyne functionality beside the outstanding properties the silole ring encouraged us and others to explore the combination of siloles and alkynes in new, and hopefully, interesting ways. The sustained advancement of silole based acetylene chemistry lies in no small part from the pioneering efforts by the groups of Tamao and West to construct the silole ring, including the development of new synthetic methodology based on transition metal and metal acetylides, and meticulous exploration of silole properties has provided a solid foundation for others to build upon [12].
In 1997, Barton and co-workers utilized the versatile dibromo silole 6 [12] to synthesize the silole–acetylene polymers 7 and 8 [13]. If compared to the corresponding poly(phenyleneethynylene)s (λmax = 425 nm) [14] and poly (thiopheneethynylene)s (λmax = 438 nm) [15], the silole-containing polymers significantly showed red-shifted absorptions implicating the importance of the silole ring in the properties of these substances (Fig. 2).

Absorption data for polymers in THF at room temperature.
In 1998, Tamao and co-workers reported a series of 2,5-diethynylsilole monomers 9 and their polymers 10 (Fig. 3) [16]. While PAE type polymers normally have relatively large band gaps of about 2.1–2.6 eV, the synthesized diethynylsilole-based polymers significantly showed narrower band gaps up to 1.8 eV. Also, an additional red shift in the absorption spectrum was observed when diethynylthiophene moiety was incorporated. Despite the unique features of these novel siloles, the conductivities of the synthesized polymers upon doping with iodine were found to be moderate.
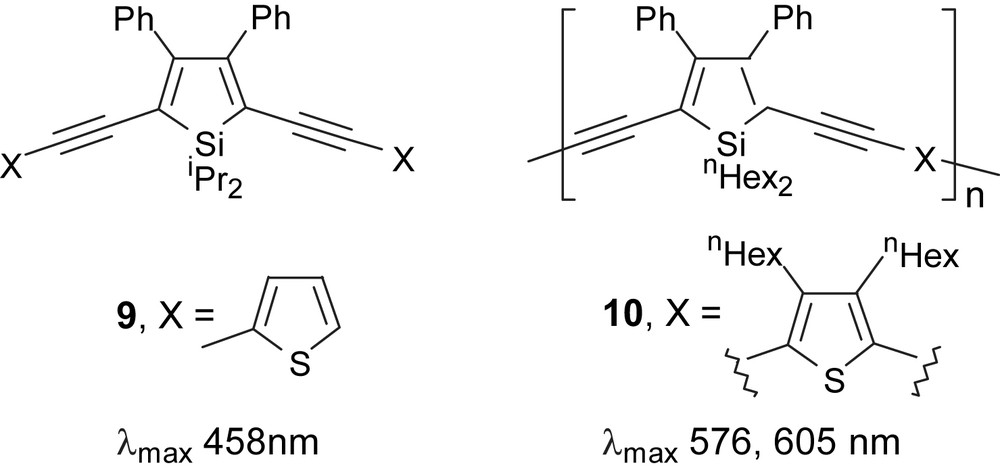
Absorption data for polymers in chloroform at room temperature.
In the same year, Ohshita et al. reported polymers having 2,5-diethynylenesilole systems linked by an organosilicon bridge (e.g. 11, Fig. 4) and demonstrated that these polymers exhibit an enhanced conjugation along the polymer backbone due to the presence of the silicon spacer. As a consequence, these polymers were presented as a new class of conjugated polymers with small band gaps and high thermal stability [17].

Absorption data for polymers in THF at room temperature.
A classic way to fine tune the electronic properties of a chromophore is to modify substituents at the periphery, but the ability to vary the functional groups at the silole termini has been impeded by the challenges of synthesizing dissymmetric siloles [18]. Additionally, the synthesis of length-specific oligomers requires the use of a dissymmetric building block that can serve either as an end cap or as a starting point for iterative chain extension [19]. In this regard, we reported a more direct route to obtain the dissymmetric siloles 14 through site-specific cross-coupling methodology, utilizing ZnCl2 as an oxidizing agent for residual LiNaph [18]. The important consequence of using excess ZnCl2 was the presumed transmetallation of the silole (to give 13), which, compared to the dilithio-silole, is considerably less basic and less nucleophilic. This attenuation in activity allowed formation of a dissymmetric silole by clean monochlorination (16.2:1 ratio) using N-chlorophthalimide (NCP), and followed by iodination with I2 to afford the new chloroiodosilole 14 in 81% yield (Scheme 1) [18]. The synthesis of this strategic intermediate was a milestone step for us, and opened a new pathway toward structurally tuned silole chromophores and oligomeric siloles of precise composition.
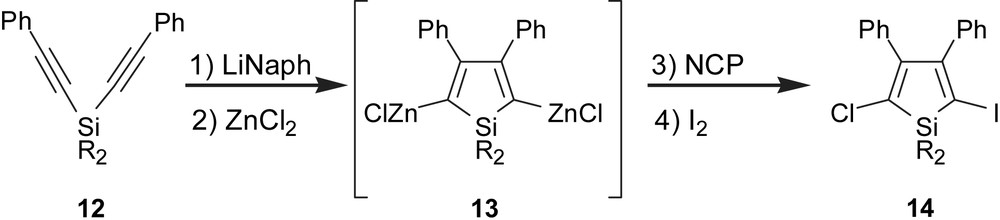
1Synthesis of dissymmetric silole building block.
Working with 14 can be complicated by its high light sensitivity and instability, but a quick cross-coupling of the more reactive iodide generally gives a stable chloro intermediate. Also, this strategy was employed to successfully develop a direct, two-step and highly efficient sequence for the preparation of dissymmetric siloles. Therefore, in-situ engagement of intermediate 14 in two successive cross-coupling reaction afforded the first silole-containing extended chromophores bearing electronically dissimilar functional groups at C(2) and C(5) 15 (Fig. 5) [18].

Donor–acceptor desymmetrized siloles.
The electronic spectra of DA siloles 15a–j are shown in Table 1. A stepwise bathochromic shift was observed in the electronic absorption spectra from 429 nm (parent silole 15a) to 496 nm (the most polar silole 15j), indicating the important role of electron delocalization in these substances which can be fine tuned by manipulation of peripheral push–pull substituents at the C(2) and C(5) positions. This synthetic advance made it possible to bring siloles into the family of electronically tuneable DA chromophores [18]. The consequences of varying the nature of the DA groups were also manifested in the photoluminescence spectra (Table 1). Although it exhibited only the fifth longest wavelength absorption in this series, silole 15e displayed the longest wavelength emission, at 649 nm. To the best of our knowledge, this is the longest wavelength emission for a compound possessing only a single silole in the chromophore. We suspect that dimerization of 15j is responsible for emission quenching. This communication constituted the first successful alkyne cross-couplings under Negishi conditions and demonstrated the ability to manipulate the electronic and physical properties of siloles through judicious combinations of peripheral functional groups.
Summary of data of DA siloles determined in dichloromethane at room temperature.
Silole | Donor (D) | Acceptor (A) | Absorption λmax | Emission λmax |
a | H | H | 429 | 520 |
b | OCH3 | H | 435 | 528 |
c | OCH3 | CN | 443 | 559 |
d | NH2 | H | 445 | 558 |
e | OCH3 | NO2 | 456 | 649 |
f | NH2 | CN | 457 | 594 |
g | NMe2 | H | 465 | 598 |
h | NH2 | NO2 | 471 | 613 |
i | NMe2 | CN | 476 | 611 |
j | NMe2 | NO2 | 496 | Not detectable |
We also illustrated the importance and utility of the mixed silole 14 as a powerful intermediate for iterative oligomeric silole synthesis [19]. The crucial building block 16, obtained from 14, served either as an end cap or as a starting point for iterative chain extension to obtain a structurally homologous family of novel oligomeric chromophores 17a–d (Fig. 6).
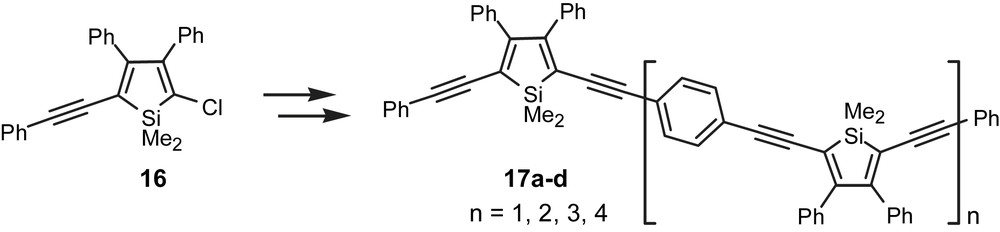
Homologous family of novel oligomeric silolene chromophores.
The electronic spectra of the oligomers 17a–d are summarized in Table 2. When compared to the analogous high molecular weight silole-containing polymers 7 (Fig. 2), the relatively short tetramer 17c and pentamer 17d displayed similar absorption maximum. From these observations it appears that the effective conjugation length within the corresponding silole polymers was established for the first time, and that it was found to be approximately equal to that of the tetramer 17c [19]. On the other hand, the quantum efficiencies were modest, with the monomer being most efficient at 9%. Yet, the unprecedented molar absorptivities observed for these oligomers (extinction coefficients are greater than 120 000 M−1 cm−1) make them potential candidates for photovoltaic applications.
Summary of data of oligomeric siloles 17, determined in dichloromethane at room temperature.
Oligomer | n | Absorption λmax | Emission λmax |
a | 1 | 467 | 526 |
b | 2 | 479 | 543 |
c | 3 | 492 | 550 |
d | 4 | 492 | 538 |
To gain insight into the influence exerted by a single silole ring on the properties of an extended chromophore, we synthesized 18 (Fig. 7) for direct comparison with its silole counterpart, trimer 17b. The absorption and emission maxima of 18 were blue-shifted relative to 17b, but interestingly the quantum efficiency is 20.11 × 10−2 in the case of 18 versus 0.37 × 10−2 for trimer 17b [19].

Trimer 17b and extended chromophore 18.
These observations suggested that silole luminescence attenuation classically attributed to the 3,4-diphenyl rings can be overcome by manipulation of the steric bulk of the C2, C5, and Si substituents which would increase the energy barriers for non-emissive decay processes and ultimately result in increased photoluminescence. Thus, we investigated synthetically some silole modifications intended to impart “rigidity” or restricted rotation compared to the parent chromophore 15a. The outcome of this effort was the synthesis of the first highly luminescent 3,4-diphenylsilole chromophore 19 (Fig. 8), having a quantum efficiency of 63% (determined with reference to fluorescein) [20]. When compared to that of 15a (quantum efficiency is 9%) [18], this pioneering discovery welcomed siloles as promising structurally tunable luminophores and unambiguously refutes the notion that all 3,4-disubstituted siloles will possess intrinsically low quantum efficiencies.

Sterically locked, highly luminescent silole.
The high quantum efficiency observed (at least) for this family of siloles motivated us to investigate their electrogenerated chemiluminescence (ECL) properties [21] and these compounds were found to be consistently more efficient fluorophores and generally exhibited greater radical ion stability than their ethylene-substituted counterparts. While increasing steric protection in ethynyl compounds improves both fluorescence efficiency and radical ion stability, it attenuates that of ethylene counterparts [21].
In 2007, Ding et al. reported a series of 3,4-diphenylsiloles incorporating arylene ethynylene strands at the 2,5-positions (e.g. 20) [22]. Additionally, the effect of chain length on the photoluminescence properties was investigated. In comparison to our silole 18 (quantum efficiency is 20%), double incorporation of arylene ethynylene strands in 20 (quantum efficiency is 50%) was found to be effective for enhancing the photoluminescence. Also, elongation of the arylene ethynylene chain involving at least three aromatic rings led to high quantum yields even without any chemical modifications of the chain, Fig. 9 [22].

An example of Ding's siloles.
In 2005, Wong et al. reported the synthesis and characterization of the first examples of a soluble, thermally stable, low band gap, silole-containing platinum (II) polyynes 22 and the corresponding model monomer 21 [23]. Inclusion of the metal ethynyl unit resulted in intense π–π∗ transitions (λmax = 470 and 504 for 21 and 22, respectively) suggesting an intramolecular interaction between metal ethynyl units and silole rings that substantially perturbs the electronic structure of the parent silole unit [23]. In contrast, the quantum efficiencies dramatically decreased from the model compound 21 (40%) to that of the polymer 22 (10%). It was suggested that the reason for the poor PL observed with compound 22 may be the greater number of quenching sites and/or the possibility of bimolecular decay Fig. 10 [23].
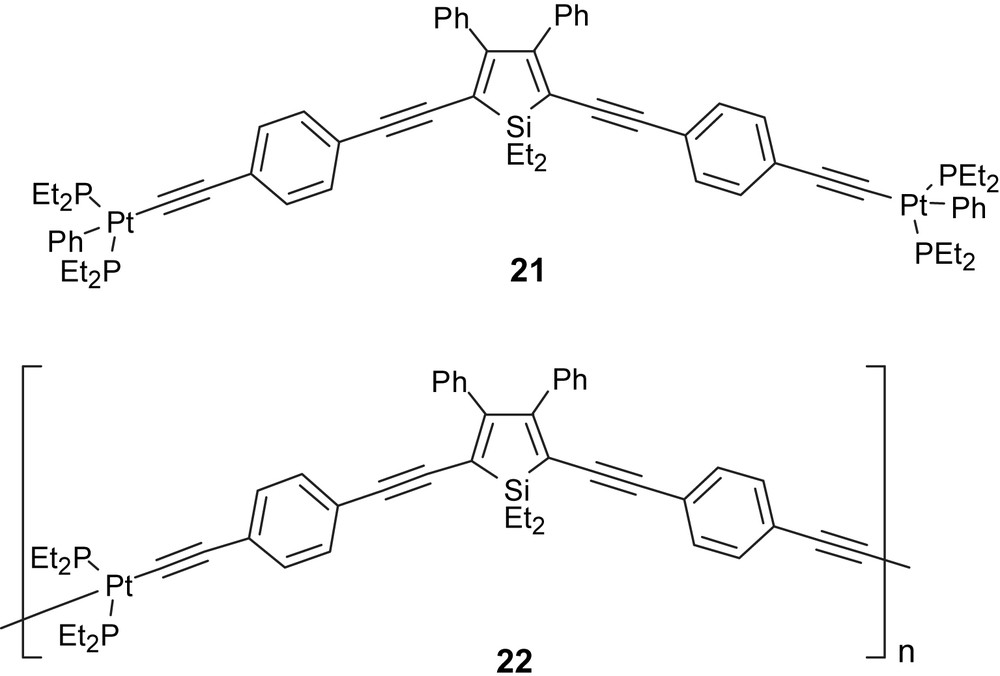
Polyplatinaynes siloles.
Anionic siloles have been investigated as cyclopentadienyl ligand analogs [24], and recently Ohshita et al. reported the synthesis of related neutral Fe(CO)3-coordinated poly(disilanylene-3,4-diethynylenesiloles) 24 [25]. As expected, the coordination of Fe(CO)3 to the 3,4-diethynylsilole unit leads to significant changes in the polymer electronic states and the UV absorptions move to longer wavelength from those of the parent non-coordinated polymer 23, indicating the enhancement of p-conjugation by the Fe(CO)3-coordination. This red shift was attributed to the increase of the bond order between silole C3–C4 atoms, through which the two ethynyl groups may be conjugated more effectively Fig. 11 [25].

Fe(0)-coordinated 3,4-diethynylsilole.
To know how the substituent position about the silole ring affects the electronic states of Fe(CO)3-coordinated diethynylsilole-containing polymers, Ohshita and co-workers synthesized 2,5-diethynylsilole 25 (Fig. 12) [26] to compare their properties with those of 3,4-diethynylsilole derivative 27. Although, the non-coordinated compound 25 exhibited red-shifted absorptions relative to 27, Fe(CO)3-coordination to 2,5-diethynylsilole system in 26 led to blue shifts of the absorption maxima. On the other hand, the absorption maxima of 3,4-diethynylsilole are red-shifted by Fe(CO)3-coordination 28.

Fe(0)-coordinated 3,4- and 2,5-diethynylsilole.
In 2001, a rare and fascinating example related to some of the silole systems described here was reported by Marder and co-workers, in which the reductive coupling of two butadiynes at a rhodium centre was utilized successfully to construct 2,5-bis(arylethynyl)metallacyclopentadiene 29 (Fig. 13) [27]. In addition to its stability, the high luminescence observed with this compound makes it an attractive system for further study as an emissive material in OLEDs.

Marder's metallacyclopentadiene.
3 Conclusion
The rapid advances in alkyne synthetic processes have facilitated an astounding abundance of new π-conjugated systems with fascinating properties and potential for materials applications in a variety of important areas, including NLOs, ECL and PV. This mini-review has highlighted some of our work in this area, focusing on those featuring a silole core, and some related systems were also discussed.
Acknowledgments
We thank the Natural Sciences and Engineering Research Council of Canada (NSERC) and the Egyptian Academy of Scientific Research and Technology for partial financial support.