1 Introduction
The Baeyer-Villiger oxidation of ketones is a reaction of major synthetic interest in organic chemistry with a large range of possible applications spanning such diverse areas as the synthesis of antibiotics and steroids, the synthesis of pheromones for agrochemistry, the synthesis of monomers for polymerization. These reactions have been commonly carried out using peracids, such as persulfuric acid, perbenzoic acid, m-chloroperbenzoic acid (m-CPBA) and hydrogen peroxide (H2O2) [1–3]. Homogeneous catalysts have been proposed for various types Baeyer-Villiger reactions [4–8] allowing to reach regio- or enantioselective oxidations [9–12]. Heterogeneous catalysis has several advantages over homogeneous catalysis:
- • simplicity in synthetic operations;
- • prevention of the production of salt wastes during neutralization of the catalysts or reagents;
- • reusability of the solid catalyst. Solid acids [13], polymer-anchored Pt complexes [14] or Bi(III) triflate [15] have been used as catalysts with aqueous H2O2 solution.
Corma et al. [16–21] reported that Sn/zeolite-beta was very active and selective for the Baeyer-Villiger oxidation of cycloketones or aromatic aldehydes with H2O2, leading to the synthesis of fragrance compounds. Natural clay minerals are eco-friendly and have been frequently used as catalyst support. Both palygorskite, (Mg,Al)5Si8O20(OH)2(H2O)4·4H2O [22] and MgAl hydrotalcite exchanged by Sn [23] have been reported to catalyze the oxidation of cyclic ketones with H2O2.
Strategies consisting in the preparation of a peracid “in situ” have also been described: one approach uses nitriles as solvents and H2O2 in the presence of solid bases [24,25], leading to the intermediate formation of an iminoperacid. In this case the nitrile is a reactant, which is consumed in the reaction with formation of an amide. Murahashi et al. reported on this reaction forming a peracid in situ by reaction of benzaldehyde and oxygen for the first time [26]. This proposal was later extended by Kaneda et al. using a combination of molecular oxygen and aldehydes over Fe containing catalysts [27–34]. This process has been extended to various redox systems supported by cation exchangers [35], MCM-41 [36], modified silica [37] or zeolites [38] using O2/PhCHO at low reaction temperatures. The catalyst was reusable without any appreciable loss in its activity and selectivity.
The use of Fe catalysts is attractive because the iron-containing compounds are considered to be of lower toxicity and are easily available. In this process, benzaldehyde can be converted to perbenzoic acid, which performs at room temperature, the reactions reported in Scheme 1. Fe-MCM has recently been reported to catalyse this reaction [36]. We report herein the Baeyer-Villiger oxidation of cyclohexanone by O2-PhCHO in the presence of Fe-pillared clays and iron tetrasulfophthalocyanine covalently supported onto silica (FePcS-SiO2).
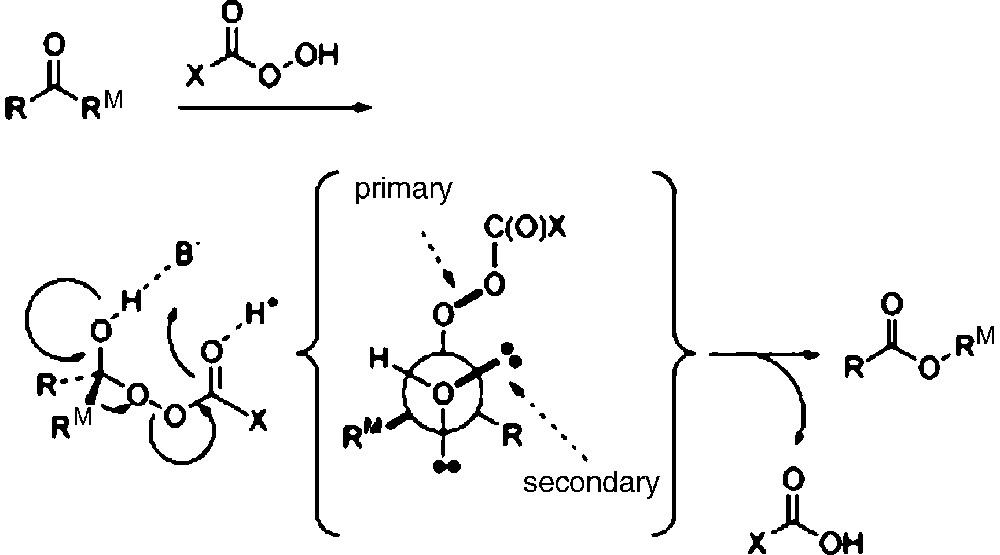
Mechanism of Baeyer-Villiger oxidation of aldehydes and ketones.
2 Experimental
2.1 Preparation of the catalyst
The starting clay used was a montmorillonite from Maghnia, with a (001) spacing of 1.26 nm. The natural clay was purified by sedimentation in order to obtain small particles (< 2 μm) of pure montmorillonite (so-called Lalithe) [39]. The pillaring solution was prepared by slowly adding a measured amount of a 0.1 M FeCl3.6H2O (97%, Aldrich) solution to a 0.1 M solution of chlorhydrol Al2(OH)5Cl,2H2O, then aging this mixture during 18 h at room temperature. This solution was then added under stirring, to a 1 wt % suspension of the colloidal clay in water, then heated at 353 K, and maintained under stirring for 2 h at this temperature. The flocculating clay was then washed with distilled water until chloride free. The pillared clay was then dried and calcined 5 h to 623 K, reached with a temperature ramp of 5 K/min. The two solids used here were prepared with a ratio Al/clay = 10 mmol/g for the Al-pillared clays, and 10 mmol Al3+ mmol Fe per g for the AlFe-pillared clays (Table 1). They show after intercalation an interlayer spacing of about 2 nm, reduced to 1.7 nm after calcinations above 300 °C. The surface areas of the calcined materials are reported in Table 2. Both types of compounds show a surface area above 210 m2/g, and similar size of pores.
Chemical compositions and basal d001 spacings of the clays used as catalysts.
Sample | % SiO2 | % Al2O3 | % Fe2O3 | d001 |
Original clay | 67.18 | 20.49 | 2.39 | 1.25 |
Al10Fe0 | 54 | 38 | 2 | 1.95 |
Al10Fe3 | 51 | 28 | 17 | 2.14 |
Specific surface areas and porosities of the pillared clays.
Sample | Al10Fe0 | Al10Fe3 | Original clay |
S BET (m2/g) | 209 | 261 | 10 |
Sμp (m2/g) | 152 | 144 | / |
Vμp (mL/g) | 0.075 | 0.074 | / |
Pores diameter (nm) | 2.48 | 2.43 | 9.60 |
The heterogeneous catalyst FePCS-SiO2 was prepared according to published procedure [40]. A solution of 52 mg of FePc(SO2Cl)4 in 20 mL of pyridine was stirred for 18 h. Then this solution was added to a suspension of 1.5 g of amino-modified amorphous silica (0.5 mmol of NH2 per 1 g, prepared from Degussa Aerosil 200 and 3-aminopropyltriethoxysilane) in 12 mL of pyridine at 20 °C under Ar. The resulting mixture was stirred at 20 °C for 1.5 h, then at 80 °C for 24 h. A green material was separated by filtration and washed with acetone and water. The material was dried under vacuum at 80 °C for 24 h and characterized by chemical analysis, surface area determination (185 m2/g) and the diffuse reflectance UV-vis spectroscopy that evidenced the effective complex grafting (360, 436 and 682 nm). The complex loading was determined by metal analysis using an inductively coupled plasma-mass spectrometry method to be equal to 26.9 μmol/g. The covalent linkage is provided by a sulfamide bonds between amino-groups of silica surface and sulfochloride functions of FePcS.
2.2 Catalytic reaction
The catalytic reaction was the Baeyer-Villiger oxidation of cyclohexanone using benzaldehyde and oxygen at room temperature. O2 was bubbled at the rate 30 mL/min through the reaction mixture consisting typically of benzaldehyde (20 mmol), cyclohexanone (10 mmol) and 50–200 mg of catalyst in 60 mL of benzene solvent. Fe-pillared clay catalyst was kept in the oven at 120 °C and was taken prior to catalytic experiment. The conversion of cyclohexanone was measured by gas chromatography (GC) using a Perkin-Elmer Autosystem equipped with a capillary column coated with DB5 (8% diphenyl-95% polysiloxane). The products of reaction, caprolactone and benzoic acid were identified by GC-MS using the same column. Samples were taken at different times and analysed by GC to measure the conversion and yield. A calibration was made for the quantitative analysis of cyclohexanone, benzaldehyde, caprolactone and benzoic acid, allowing of measuring the conversion of cyclohexanone and the yield in caprolactone. The selectivity into caprolactone is then defined as the ratio yield/conversion. The carbon balance has systematically been calculated and is better than 98%.
3 Results and discussion
3.1 General trends of oxidation in the presence of iron-pillared clays
The reaction of cyclohexanone with dioxygen and benzaldehyde yielded caprolactone and benzoic acid. The oxidation proceeds via the intermediate oxidation of the aldehyde to a peracid. When benzene was used as the solvent the reaction was quite selective to give above 80% selectivity in caprolactone. The main by-product detected by GC-MS was 6-hydroxyhexanoic acid resulting from the acid catalysed ring opening of the lactone by water.
The conditions allowing to achieve reproducible results were determined. In order to avoid that the reaction could be limited by external mass transfer, the stirring speed was above 900/min when using 0.1 g of catalyst. The time course of conversion showed an induction time of about 60 min (Fig. 1). Notably, the reaction rate was rather insensitive to the amount of catalyst and stirring speed thus suggesting that the reaction was probably not truly heterogeneous.
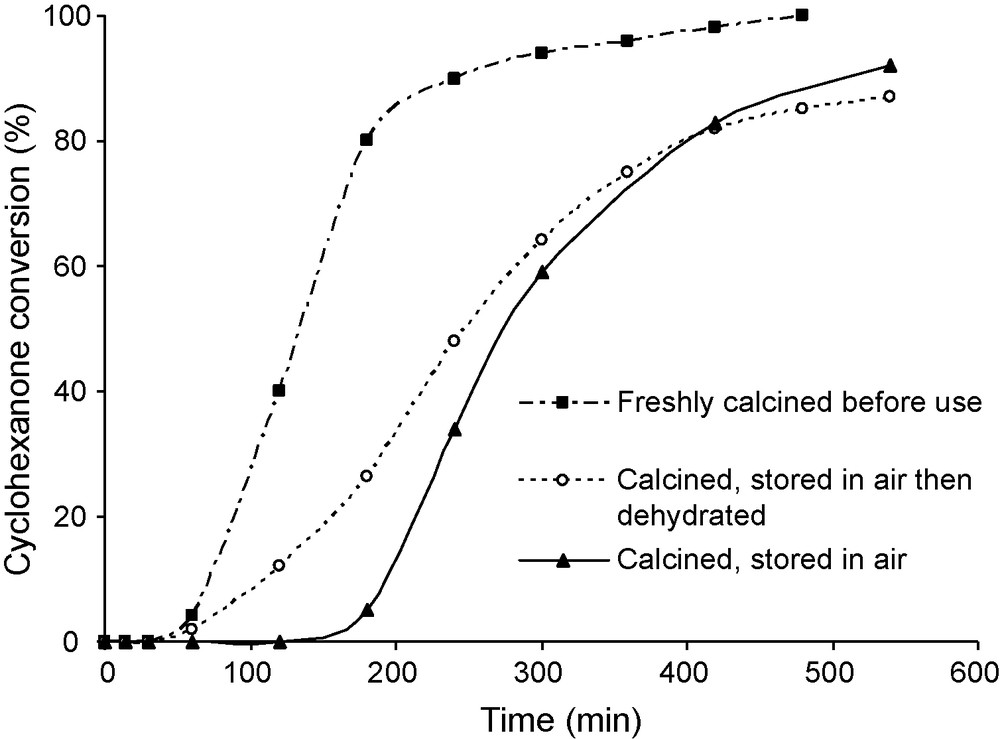
Effect of the pre-treatment of Al10Fe3 on the conversion of cyclohexanone. Reaction conditions: mass of catalyst 50 mg, reaction temperature 20 °C, 60 mL of benzene 30 mmol of benzaldehyde, 10 mmol of cyclohexanone and an O2 flux of 30 mL/min.
An important observation is the effect of the pre-treatment of the pillared clays, attributed to an inhibition of the reaction by water. The comparison of three different samples:
- • freshly calcined;
- • calcined then stored in air;
- • stored in air and dehydrated at 393 K before use, is reported in Fig. 1.
Significant differences in catalytic activity of these samples were observed. The most active catalyst was the freshly calcined one, i.e. containing less amount of water.
This reaction was also sensitive to solvent as illustrated in Fig. 2. Benzene was the best solvent providing a higher conversion. Polar solvents such as acetonitrile and methanol lead to lower catalytic activity. With methanol as solvent, the main reaction, competing with Bayer-Villiger oxidation, became the acid catalyzed reaction of cyclohexanone with methanol forming acetals. Therefore, benzene was selected as standard solvent.
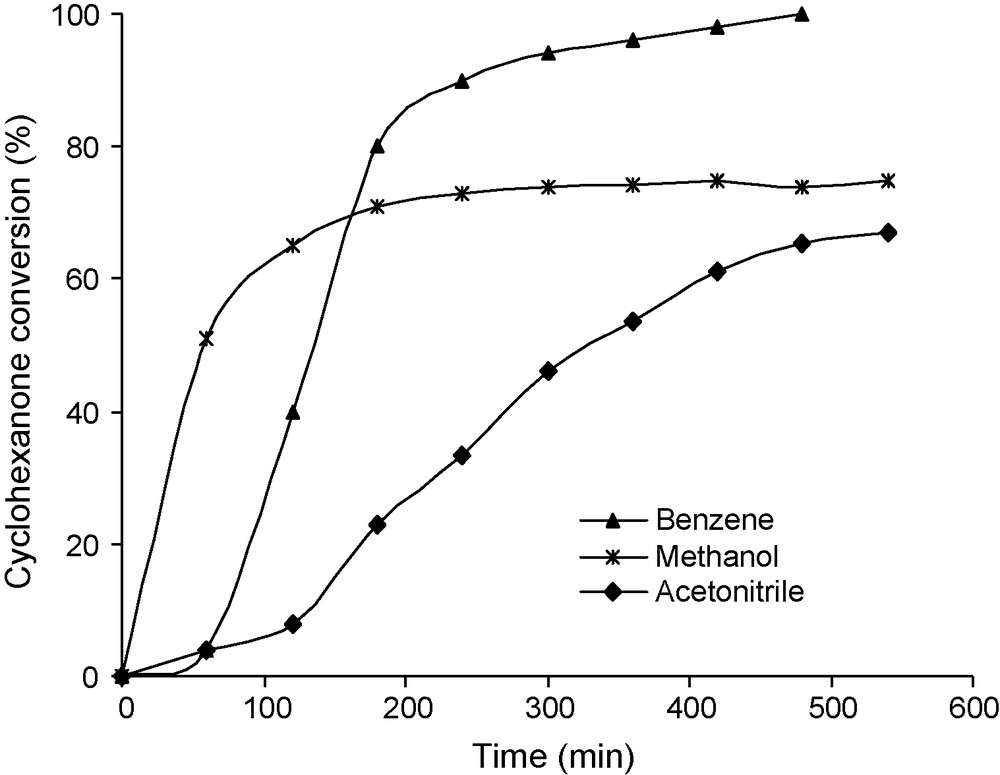
Effect of different solvents on the kinetics of the reaction. Reaction conditions: mass of catalyst 50 mg, reaction temperature 20 °C, 60 mL of solvant 30 mmol of benzaldehyde, 10 mmol of cyclohexanone and an O2 flux of 30 mL/min.
3.2 Influence of the benzaldehyde concentration
The effect of the concentration of benzaldehyde was investigated by varying the amount of this reactant from 20 to 50 mmol dissolved in 60 mL of benzene (Fig. 3). After an initial induction period, a first order rate law was observed. The rate constant determined from this plot, first increases with the concentration of benzaldehyde, goes through a maximum at about 30 mmol, then decreases. The yield in lactone (ratio moles of lactone formed in the reaction per moles of benzaldehyde reacted) is about 40% for 20 mmol of benzaldehyde and decreases when the concentration in benzaldehyde increases (Fig. 4). This decrease was therefore attributed to the decomposition of perbenzoic acid formed in higher concentrations. The lower yield observed when the temperature was increased is also consistent with this proposal (Fig. 5). It was checked (not shown) that benzoic acid, formed as by-product, had no inhibitory action in the reaction.
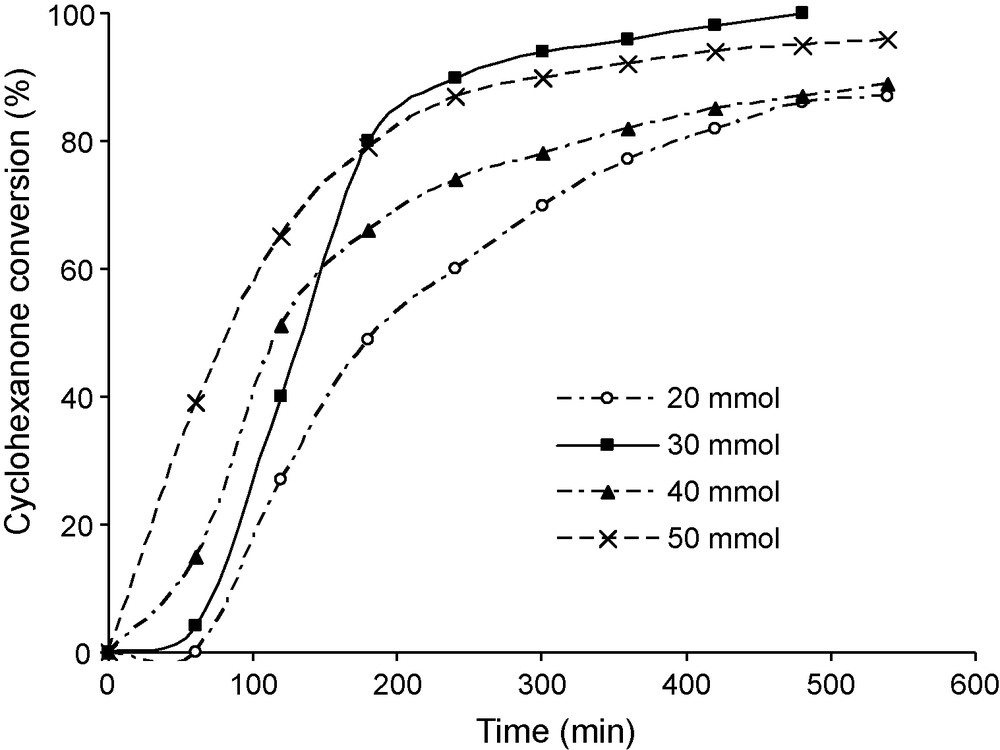
Effect of benzaldehyde concentration on the conversion of cyclohexanone. Reaction conditions: mass of catalyst 50 mg, reaction temperature 20 °C, 60 mL of benzene, 10 mmol of cyclohexanone and an O2 flux of 30 mL/min.
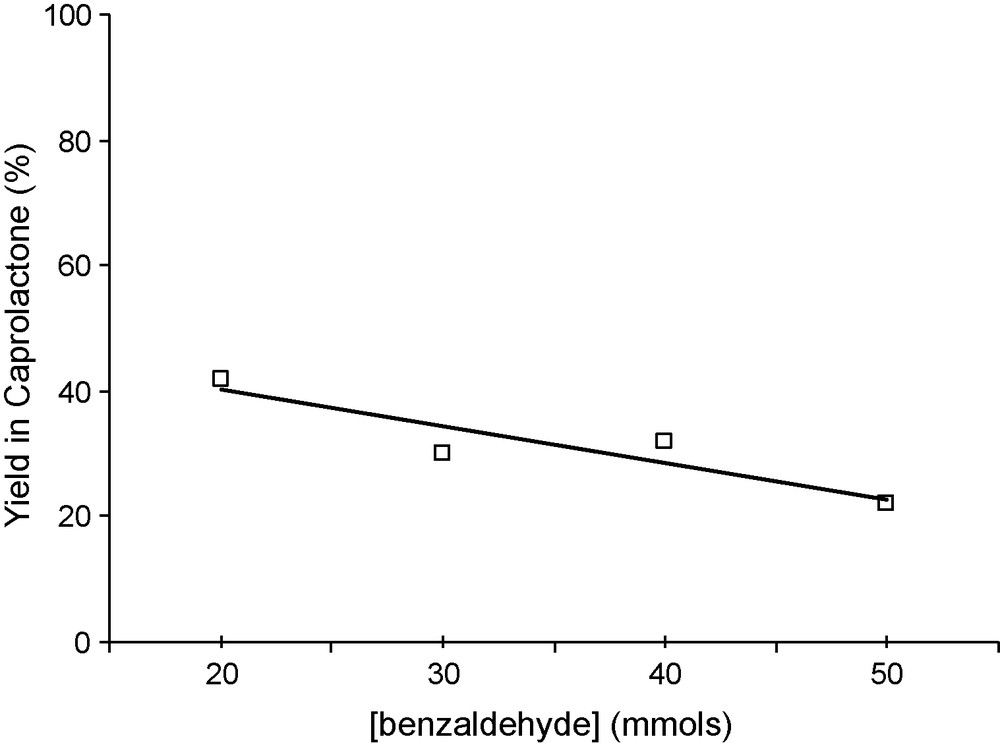
Yields in caprolactone versus benzaldehyde conversion as a function of starting concentration in benzaldehyde. Reaction conditions: mass of catalyst 50 mg, reaction temperature 20 °C, 60 mL of benzene, 10 mmol of cyclohexanone and an O2 flux of 30 mL/min.
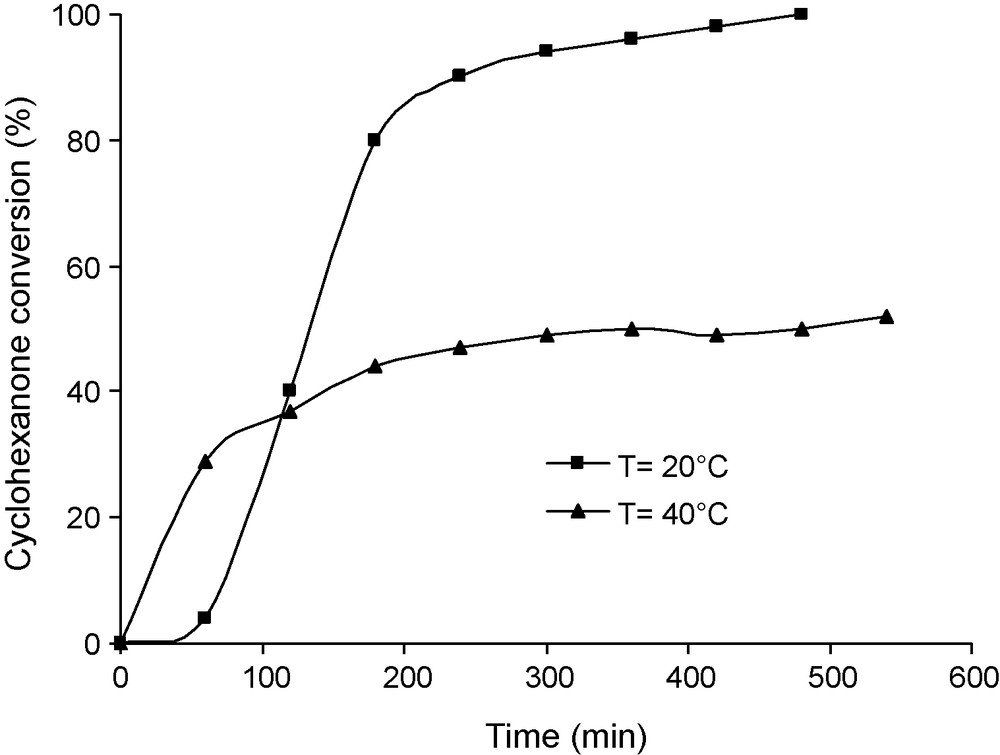
Effect of the reaction temperature on the kinetics of the decomposition of the perbenzoic acid. Reaction conditions: mass of catalyst 50 mg, 60 mL of benzene 30 mmol of benzaldehyde, 10 mmol of cyclohexanone and an O2 flux of 30 mL/min.
3.3 Influence of the composition of the clay catalyst
The activities of the original clay, Al-pillared clay and without catalyst were also determined (Fig. 6). The original non-pillared clay of low surface area, containing 2.3% Fe substituted in the lattice, shows a lower activity than the Al10Fe3, but reaches 80% conversion of cyclohexanone. The Al-pillared clay also containing 2% Fe but showing a higher surface area, was almost as active as Al10Fe3. This suggested that the induction period could be due to the dissolution of the part of the Fe3+ cations from the solid mediated by either the perbenzoic acid intermediate or the benzoic acid co-product. This proposal was supported by the disappearance of the induction period when a small amount of Fe(acac)3 was added to the reaction medium. The amount of iron present in the solution at the end of the reaction was then analysed (Table 3). About 4–5 ppm of Fe were found in the solution after decantation of the Al10Fe3. The different activities of the clays were then related to different Fe contents and accessible surface areas.
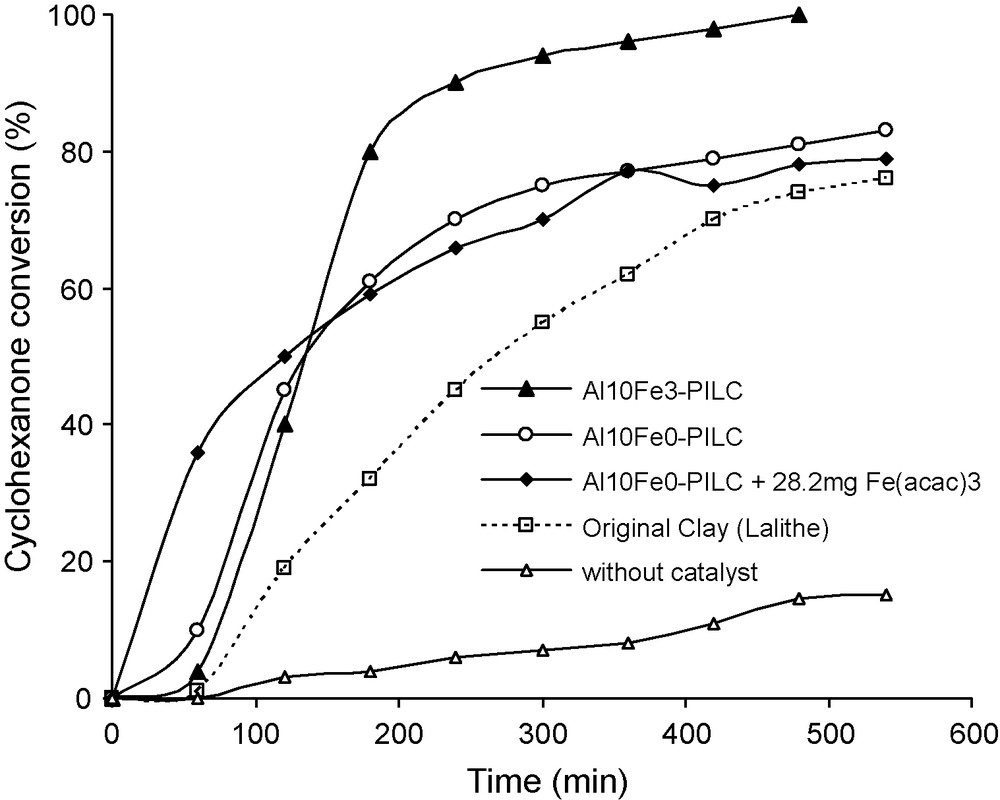
Comparison of the catalytic activity of different pillared and non-pillared clays, with and without addition of Fe acetylacetonate, and without catalyst for the Baeyer-Villiger oxidation of cyclohexanone. Reaction conditions: mass of catalyst 50 mg, reaction temperature 20 °C, 60 mL of benzene 30 mmol of benzaldehyde, 10 mmol of cyclohexanone and an O2 flux of 30 mL/min.
Chemical analysis of Fe in the reaction medium after extraction of the solids.
Catalyst Tested mass | Fe(III) acac 28 mg | Al10Fe3 50 mg | Al10Fe3 100 mg | Al10Fe3 200 mg |
Fe (ppm) | 15 | < 1 | 4 | 5 |
3.4 Homogenous catalysis in the presence of iron salts
The hypothesis that the reaction could be homogeneously catalysed was checked using Fe salts as catalysts. FeCl3 is not soluble in benzene and has been reported to be a poor catalyst, probably as a result of this insolubility. By contrast, Fe acetylacetonate shows a better solubility in benzene and was found to be a very active catalyst. In this case the induction period disappeared (Fig. 7). It was observed that 5.8 mg of Fe acetylacetonate (2.8 × 10−5 mol) were sufficient to provide 96% conversion in about 9 h, with a selectivity better than 86%. At higher Fe concentration, a lower rate was observed with only marginal changes of selectivity. The decrease of activity at higher concentration could be explained by the formation of higher amount of perbenzoic acid promoting its direct hydrolysis to benzoic acid at the expense of its reaction with cyclohexanone. This effect is similar to that produced by increasing the initial benzaldehyde concentration as described above (Fig. 4). Under the same conditions, the addition of benzoic acid to the initial reaction mixture did not inhibit the reaction rate (Fig. 8), but rather slightly promoted the activity, selectivity being unchanged at about 86%. No specific data allowing to account for this effect have been obtained.
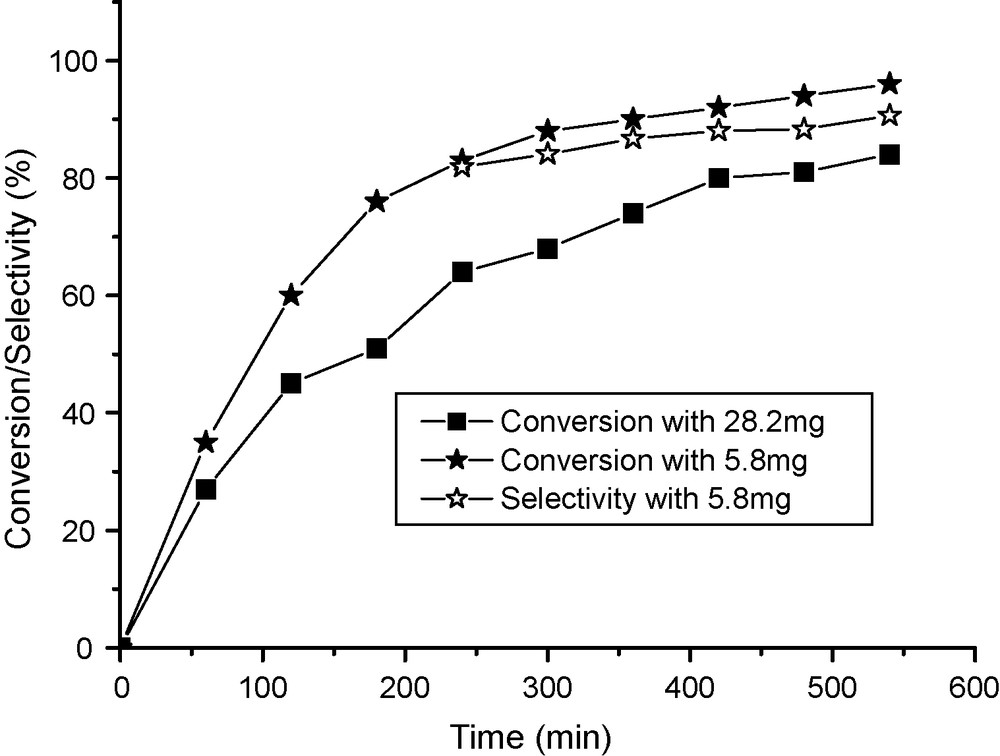
Baeyer-Villiger oxidation of cyclohexanone catalysed by Fe(acac)3. Reaction conditions: reaction temperature 20 °C, 60 mL of benzene 30 mmol of benzaldehyde, 10 mmol of cyclohexanone and an O2 flux of 30 mL/min.
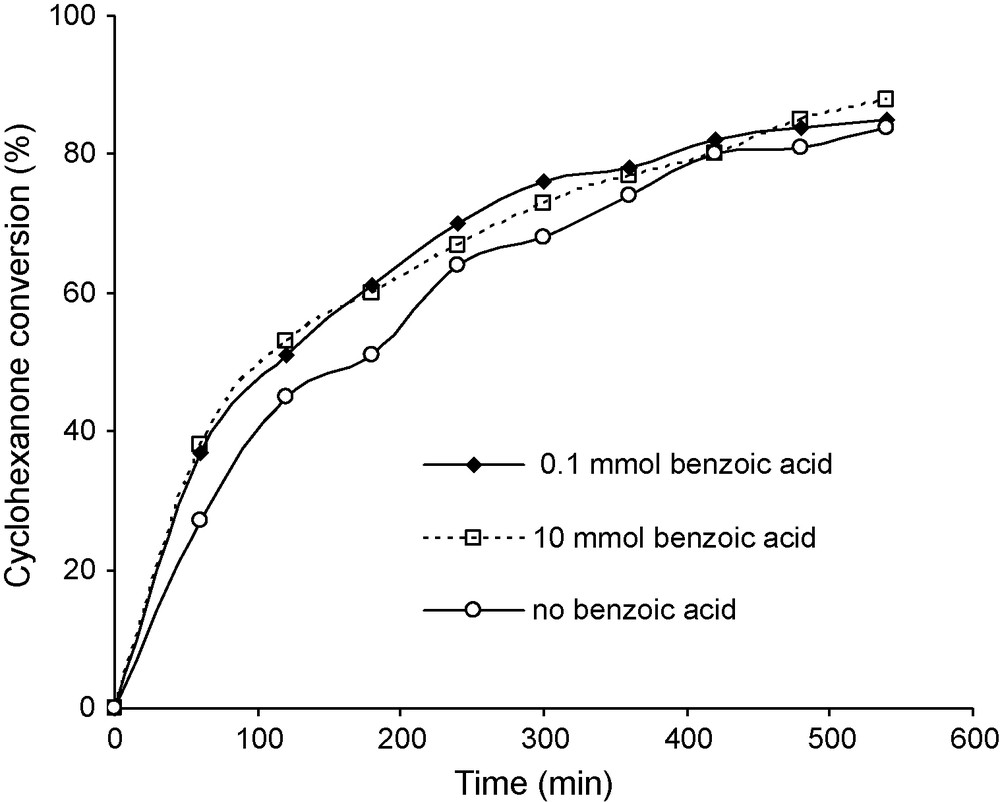
Kinetic influence of benzoic acid on the conversion of cyclohexanone catalysed by Fe(acac)3. Reaction conditions: mass of catalyst 28.2 mg, reaction temperature 20 °C, 60 mL of benzene 30 mmol of benzaldehyde, 10 mmol of cyclohexanone and an O2 flux of 30 mL/min.
In the case of heterogeneous catalysis, it can be expected that trace amounts of Fe can be extracted by the reaction mixture from most Fe-based solids to give potential homogeneous catalysts for this reaction. The amount of iron thus dissolved in the reaction mixture could be rather small and this phenomenon could go unnoticed unless the solution is analysed. A Fe-rich sample is then expected to be recyclable, as reported by many authors.
3.5 Heterogeneous catalysis in the presence of supported iron-phthalocyanine
To avoid a leaching of iron into reaction solution, iron-containing catalyst covalently grafted onto support would be interesting. Fe-phthalocyanine based catalyst [39,41] were thus applied. Pure Fe-tetrasulfophthalocyanine showed a lower activity than Fe(acac)3, probably because of the lack of solubility. Nevertheless, the conversion reached 60% after 9 h, with a selectivity to lactone of about 80% in the presence of 9.1 mg of complex (1.4 μmol FePcS). Then, FePcS covalently anchored onto silica was used as catalyst. The results are reported in Fig. 9. In the presence of 50.9 mg FePcS-SiO2, containing also 1.4 μmol of complex, the induction period was suppressed. The selectivity was close to 100% for the lactone, and the conversion reached about 60% after 10 h. When the catalyst amount was doubled the conversion increased only slightly (Fig. 10) and the selectivity decreased below 90%, suggesting possible mass transfer limitations.
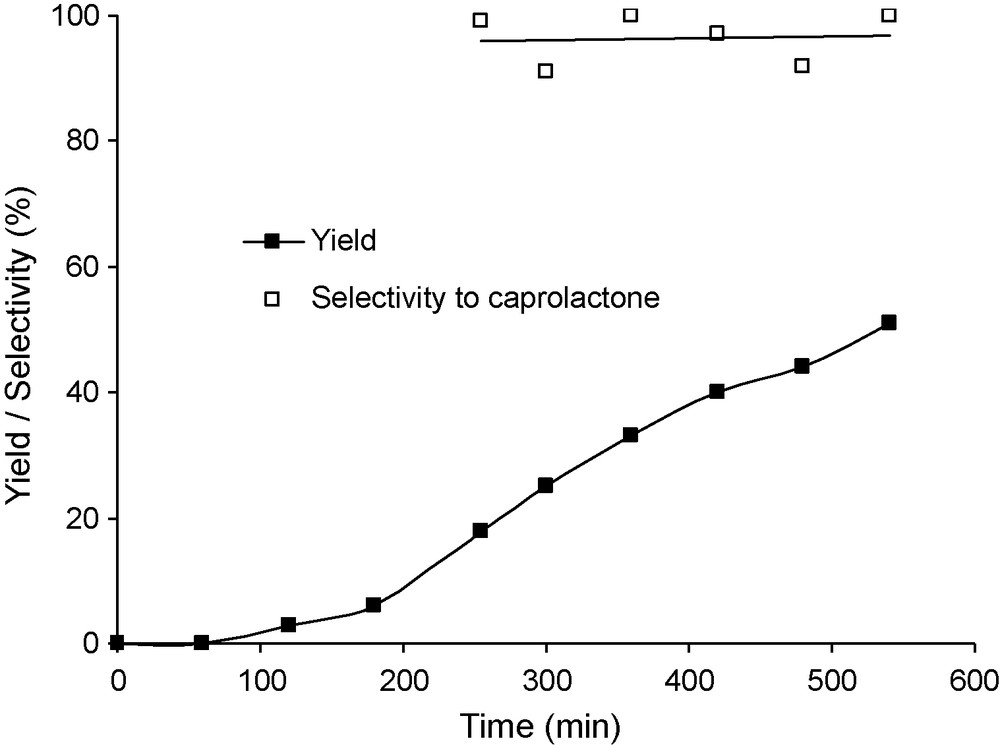
Conversion and yield as a function of time for a reaction catalysed by silica-supported phtalocyanine. Reaction conditions: mass of catalyst 50 mg, reaction temperature 20 °C, 60 mL of benzene, 30 mmol of benzaldehyde, 10 mmol of cyclohexanone and an O2 flux of 30 mL/min.
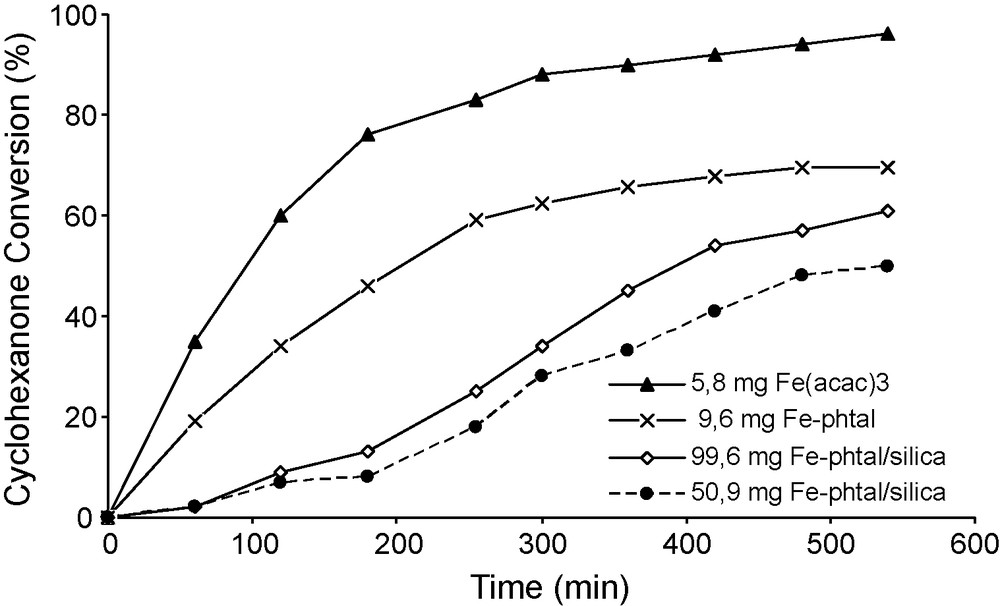
Comparison of the homogenous catalysis by Fe acetylacetonate and Fe-phtalocyanine, with the heterogeneous catalysis by silica anchored Fe-phatocyanine. Reaction conditions: reaction temperature 20 °C, 60 mL of benzene 30 mmol of benzaldehyde, 10 mmol of cyclohexanone and an O2 flux of 30 mL/min.
In order to determine a possible leaching of the complex, a decantation of the catalyst was done at the end of the reaction. This decantation is very slow (over a week) due to the small size of the silica particles, which prevent from any separation of the catalyst by filtration. Consequently this also prevent from any experiments on the filtrated solutions and reusability of the catalyst. The isolated solution was analyzed for Fe by inductively coupled plasma mass spectrometry method. The residual iron content of the solution was below the detection limit indicating the expected absence of complex leaching into solution; as expected because the phthalocyanine ligand strongly retains iron cation in the macrocycle and the complex itself is supported on the silica surface by covalent anchoring.
Since Baeyer-Villiger reaction is an important synthetic tool, it is interesting to compare the selectivities and yields obtained by different routes. The comparison of the results obtained on different catalysts in their optimum reaction conditions is reported in Table 4 in terms of catalytic cycles per metal centre and per hour or turnover frequencies. Selectivities for lactone above 95% are observed in all cases, but the yields are different depending on the catalyst. The couple Sn catalyst-H2O2 gives lower turn over frequency, around 50/h, whatever the solvent used, compared to 400/h with benzaldehyde. MnAlPo have been reported to give nearly quantitative conversions of cyclohexanone when using benzaldehyde and 30 bars of oxygen at 50 °C [41]. Mn can be extracted from MnAlPO by the acids produced in the reaction [42] therefore the catalyst may not be recyclable. Supported iron phthalocyanine competes favourably with the other catalysts, since a turnover number of about 400 mol of cyclohexanone per mol of Fe and per hour is observed, for a reaction at atmospheric pressure. Experiments with solvents other than benzene and with lower starting benzaldehyde concentrations have been undertaken in order to complete this preliminary study and to make the reaction process more economical and environmental friendly.
Baeyer-Villiger oxidation of cyclohexanone on different catalysts.
Catalyst | Oxidant | Temp-time | Yield (%) | TOF (h−1) | Reference |
Sn-BEA | H202 | 90 °C-3 h | 52 | 42 | [43] |
Sn-HDT | H202/CH3CN | 70 °C-24 h | 58 | 115 | [23] |
MgAlSn-HDT | H202/C6H5CN | 70 °C-6 h | 70 | 20 | [24] |
Ni anchored on SiO2 | PhCHO/O2 | 18 °C-3.5 h | 98 | 49 | [17,37] |
MnAlPO | PhCHO/O2 | 50 °C-6 h | 78 | 257 | [38] |
Fe-Phtal/Silica | PhCHO/O2 | 25 °C-8 h | 61 | 400 | This work |
No catalyst | m-CPBA | 20 °C-24 h | 85 | – | [44] |
4 Conclusion
Baeyer-Villiger oxidation on Fe containing oxide is most likely catalysed not in the heterogenous phase but in the liquid phase by the traces of Fe extracted from the solid. Fe phtalocyanine is to the best of our knowledge the first true iron based heterogeneous catalyst. This catalyst reaches greater than 95% selectivity at 60% conversion. The lower cost of H2O2 compared to benzaldehyde is at least in part compensated by that of the catalyst, and by the higher yield observed here.