1 Introduction
According to IUPAC, zwitterions are neutral compounds having formal unit electrical charges of opposite sign [1]. This review focuses on 1,3-phosphorus-containing zwitterions (1,3-P-zwitterions) with cyano-group at the anion center, i.e., inner salts in which a negative charge formally is localized on the carbon atom bearing cyano-group and a positive charge on a phosphorus atom and in which negatively and positively charged centers are separated just by one carbon atom (cyano-1,3-P-zwitterions).
More than 50 years ago, Horner and Klüpfel [2] discovered formation of 1,3-P-zwitterions upon reaction of tertiary phosphines with vinylidene derivatives. A typical example of reactions between phosphine 1 and alkene 2 that gives 1,3-P-zwitterion 3 is shown in Scheme 1.

Since their first synthesis, 1,3-P-zwitterions have been deserving significant attention from the scientific community [3–8]. General analysis of their properties is beyond the scope of this publication. Our review focuses on 1,3-P-zwitterions with one cyano-group at the anion center, i.e., cyano-1,3-P-zwitterions. Their general structure is shown in Fig. 1, where R1, R2, R3, are various alkyl- and alkylamino-substituents, R4 is aryl-group or hydrogen atom and X is either alkoxy- (cyano-1,3-P-zwitterions of type one) or N(Ar)C(O)OAlk-group (cyano-1,3-P-zwitterions of type two).
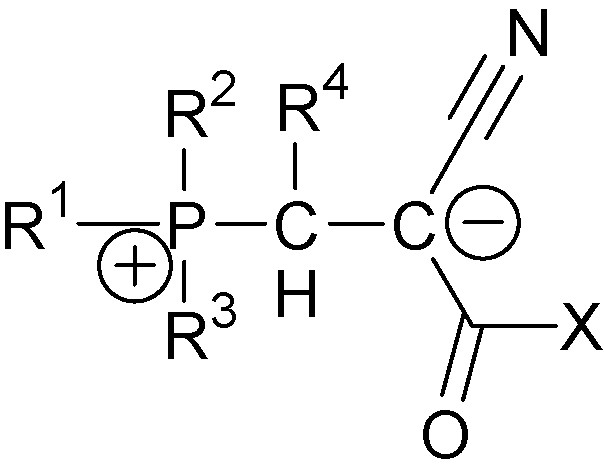
1,3-P-zwitterions of type one.
Y.G. Gololobov and his colleagues have been studying these compounds at the A.N. Nesmeyanov Institute of Organo-Element Compounds of the Russian Academy of Sciences since 1995 [9,10]. This review summarizes results they have obtained since that time.
2 Reactions of P(III) compounds with alkyl-2-cyanoacrylates and synthesis of cyano-1,3-P-zwitterions
Until now, the reaction between tertiary phosphines and substituted alkenes remains the main general method to synthesize 1,3-P-zwitterions. This reaction is usually considered an equilibrium process [3,5,11,12]; its net result depends on both the equilibrium position and the other reactions that might occur to the starting materials and/or the products. The latter depends on properties of P(III) compounds and alkenes, which in turn mostly depend on the effect of the substituents on the properties of these compounds. For the formation of stable 1,3-P-zwitterions, substituted alkenes should have strong electron-acceptor groups [13]. In this case, the equilibrium of the reaction is shifted completely to the zwitterion side and stable 1,3-P-zwitterions are the only products.
1,3-P-zwitterions of type one (Fig. 1) are synthesized by reacting P(III) nucleophilic compounds with alkyl-2-cyanoacrylates. Alkyl-2-cyanoacrylates bear strong electron-acceptor groups and therefore one might think they would always reacted smoothly with P(III) compounds with the formation of 1,3-P-zwitterions. However, alkyl-2-cyanoacrylates readily polymerize [14,15] under the action of nucleophiles and, as it is briefly discussed below, the rate of this polymerization strongly depends on the properties of P(III) compounds that react with cyanoacrylates.
Based on reactivity toward alkyl-2-cyanoacrylates, P(III) compounds can be divided into three groups [16]. The first group includes PCl3 and similarly low nucleophilic phosphorus halides that display no reactivity with respect to 2-cyanoacrylates at room temperature. The second group includes weak nucleophiles such as (EtO)3P, Ph3P. They do form 1,3-P-zwitterions to some extent by reacting with 2-cyanoacrylates, but since the equilibrium is shifted to the starting materials, concentration of alkyl-2-cyanoacrylates remains high and the major product is the polycyanoacrylate polymer bearing phosphonium group as the head group [15]. A typical example of these reactions is shown in Scheme 2.

While equilibrium of the reaction of alkyl-2-cyanocrylates with weak P-nucleophiles is shifted to the initial compounds, these reactions may still have interesting implications from the synthetic standpoint. All that is needed to avoid cyanoacrylate polymerization is to turn a newly formed intermediate 1,3-P-zwitterion into another compound [15]. This would shift the equilibrium between a weak nucleophile and alkyl-2-cyanoacrylate to the right side. Protonation of the negatively charged carbon with trifluoroacetic acid is one of the ways to implement this approach (Scheme 3). Protonation turns zwitterion 6 into unstable phosphonium salt 7. This salt spontaneously gives another P-derivative 8 by eliminating trifluoroacetic acid ester. As a result of all these reactions, the overall equilibrium is completely shifted to new P-derivative 8.
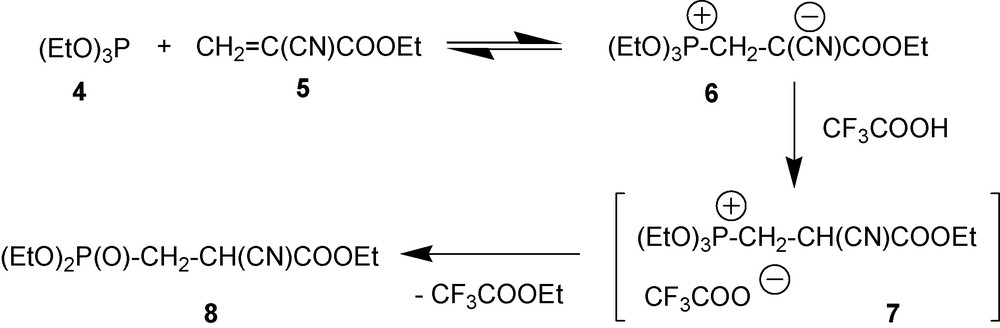
Rearrangement of newly formed zwiterrions into stable five-membered spirophosphoranes is another way of shifting the equilibrium (Scheme 4) [17]. For example, reaction of cyclic phosphite 9 with ethyl-2-cyanoacrylate 5 gives stable spiro-phospholane 10, mostly likely, through the formation of intermediate 11.
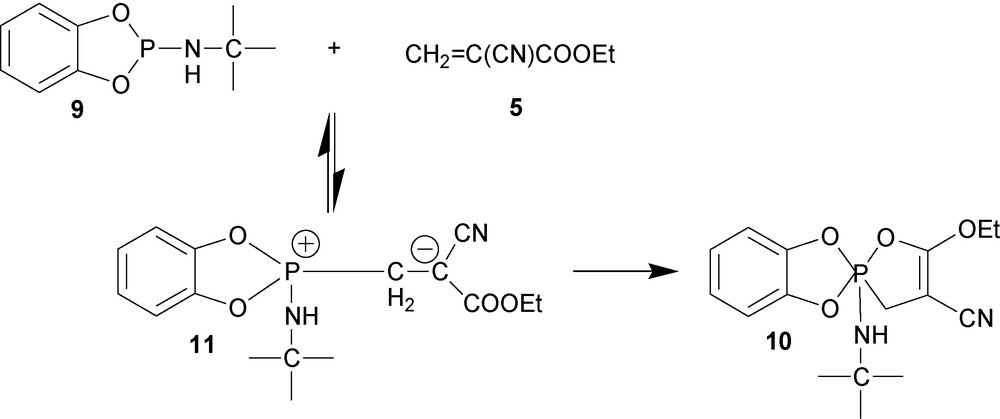
Reaction between ethyl-2-cyanoacrylate 5 and weak P(III) nucleophile 12 can be also driven to the complete consumption of the initial components by “trapping” the anionic charge of zwitterion 13 with the acidic hydrogen as shown in Scheme 5. This pathway can take place only if this proton transfer results in the formation of a stable five-member ring as in product 14.
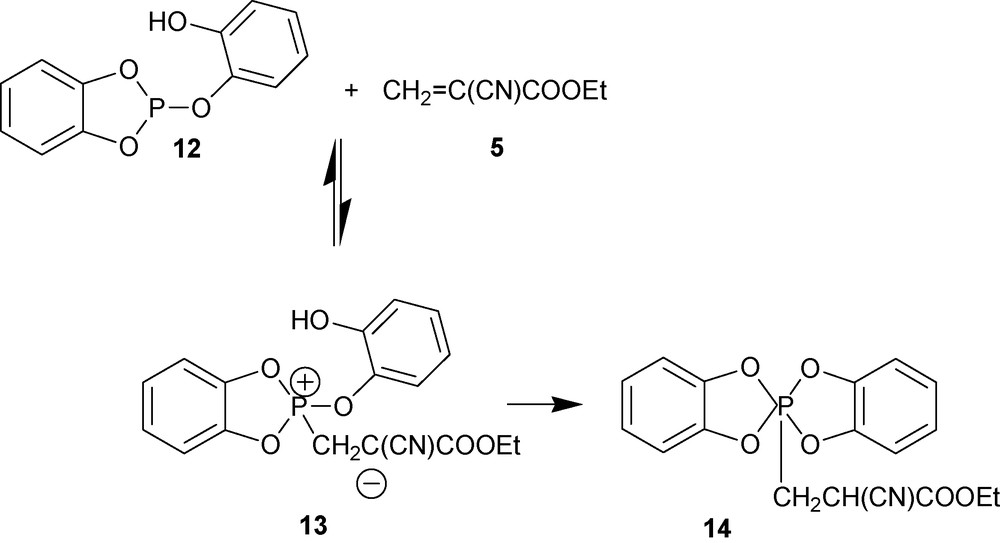
The third type of P(III) compounds includes strong nucleophiles like trialkylphosphines and hexaethyltriamidophosphite. These nucleophiles react with cyanoacrylates with the formation of stable cyano-1,3-P-zwitterions if certain conditions are obeyed [9,17,18]. High phosphine nucleophilicity is one of these conditions. The order of mixing of the reagents is another mandatory condition. For successful synthesis of target compounds, cyanoacrylate 15 must be added to the solution of phosphine 16 in a non-polar solvent in which the product is not soluble. Under these conditions, during the whole reaction a phosphine is in excess. As a result, an instantaneous formation of crystalline cyano-1,3-P-zwitterions 17 with yields of up to 50% takes place (Scheme 6).
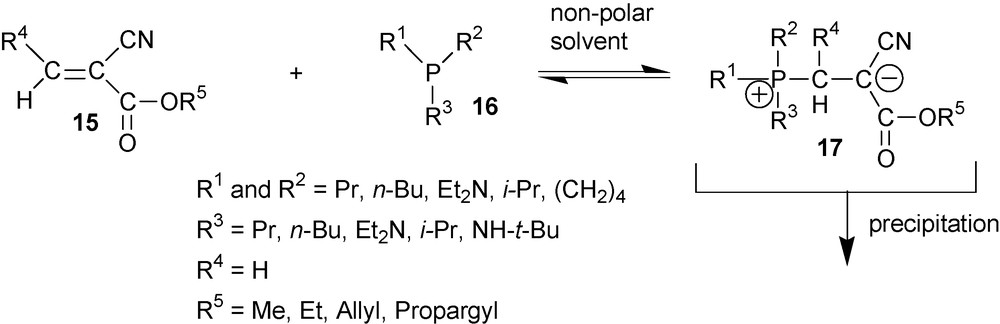
3-aryl-2-cyanoacrylates 18 react with tributylphosphine 19 in acetonitrile in a similar fashion and afford respective cyano-1,3-P-zwitterions 20 with good yields (Scheme 7) [18].
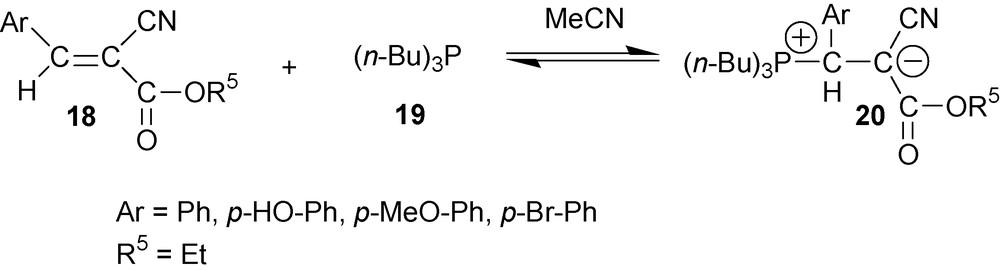
3 Type one cyano-1,3-P-zwitterions and their physicochemical characteristics
Table 1 lists spectral properties and melting points of cyano-1,3-P-zwitterions of type one that have been synthesized using approaches shown in Schemes 6 and 7.
Spectral properties and melting points of cyano-1,3-P-zwitterions 17 and 20.
Zwitterion 17 or 20 | M.p. (°C) | IR (ν, cm−1) | 31P NMR (ppm) | Lit. | |||||
R1 | R2 | R3 | R4 | R5 | CN | C(O)OR5 | |||
Pr | Pr | Pr | H | Et | 85–86 | 2148 | 1611 | N/A | [17,19] |
n-Bu | n-Bu | n-Bu | H | Et | Oil | 2148 | 1611 | 32.7 | [17,19] |
Et2N | Et2N | Et2N | H | Et | 69–70 | 2148 | 1611 | 57.5 | [17,19] |
Et2N | Et2N | NH-t-Bu | H | Et | 82–83 | 2148 | 1611 | 57.6 | [17,19] |
n-Bu | n-Bu | Et2N | H | Et | 49–50 | 2148 | 1611 | 81.9 | [19] |
i-Pr | i-Pr | i-Pr | H | Me | 133–134 | 2146 | 1627 | 38.7 | [20] |
i-Pr | i-Pr | i-Pr | H | Et | 146–147 | 2136 | 1602 | 44.9 | [20] |
(CH2)4 | Et2N | H | Et | 82 - 83 | – | – | 57.6 | [17] | |
i-Pr | i-Pr | i-Pr | H | Allyl | 123–124 | 2143 | 1619 | 38.8 | [21] |
i-Pr | i-Pr | i-Pr | H | Propargyl | 122–124 | 2160 | 1621 | 39.18 | [21] |
n-Bu | n-Bu | n-Bu | p-HOPh | Et | 106–107 | 2138 | 1598 | 36.4 (in d-DMSO) | [18] |
n-Bu | n-Bu | n-Bu | p-MeOPh | Et | 69–70 | 2140 | 1608 | 36.3 (in d-DMSO) | [18] |
n-Bu | n-Bu | n-Bu | p-BrPh | Et | 99–100 | 2140 | 1597 | 36.1 (in d-DMSO) | [18] |
n-Bu | n-Bu | n-Bu | Ph | Et | 62–63 | 2133 | 1601 | 36.4 (in d-DMSO) | [18] |
Delocalization of the negative charge is the key structural characteristic of cyano-1,3-P-zwitterions 17 and 20. While formally this negative charge is assigned to the central carbon atom, in reality electrons are spread from nitrogen of the cyano group to the carbonyl oxygen. Delocalization of the negative charge that is schematically shown in Scheme 8 follows from the experimental data [17]. As is shown in Table 1, because of this delocalization, absorption bands of the CN-groups are very intense and shift to the 2144–2160 cm−1 range. A similar shift to the region of 1597–1627 cm−1 is observed for the C(O))OR5 absorption bands. X-ray diffraction analysis of zwitterions 17 [20] and 20 [18] also support electron conjugation in cyano-1,3-P-zwitterions 17. According to these data, bond lengths are distorted from their typical values. This suggests delocalization of the negative charge along the NCCCO skeleton.
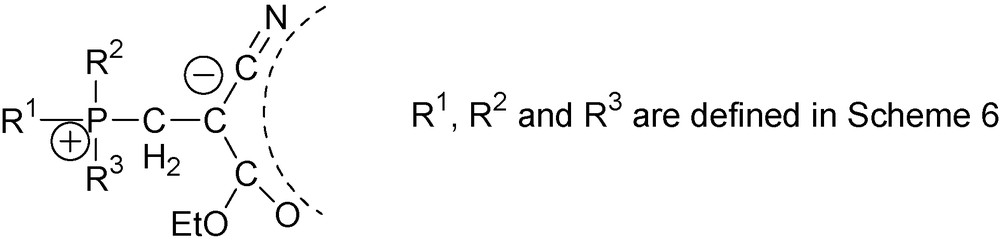
Similar phenomenon for cyano-1,3-P-zwitterions of type two is discussed in Section 5.
4 Chemical properties of type one cyano-1,3-P-zwitterions
4.1 Storage stability of type one cyano-1,3-P-zwitterions 17 and 20
Upon crystallization, zwitterions 17 are relatively stable. At 0–5 °C, they stay intact for several months. In solution, cyano-1,3-P-zwitterions 17 are much less stable and their stability varies significantly and depends on the solvent. In general, storage of these compounds in solution is not recommended. For example, 31P NMR analysis of the solutions of cyano-1,3-P-zwitterions with isopropyl substituents at the P-atom in methylene chloride, acetonitrile, acetone and THF shows presence in these solutions of several percent of tri-iso-propylphosphine from which these zwitterions were synthesized. Alkyl-2-cyanoacrylate, the second product of the zwitterion decomposition, polymerizes easily and it shifts the equilibrium to the complete decomposition of the zwitterion. Boiling solutions of zwitterions 17 in benzene results in their complete decomposition [19].
Dependence of stability on the type of the solvent is particularly striking for cyano-1,3-P-zwitterions 20 [18]. In polar solvents, such as MeCN, MeNO2, Me2SO2 [22] these zwitterions are relatively stable, whereas in solvents of low polarity tributylphosphine and tributylphosphineoxide (the product of tributylphosphine oxidation) are detected practically immediately after the dissolution of zwitterions 20 in these solvents. After several minutes, composition of the reaction mixture stabilizes and then remains unchanged almost indefinitely because, contrary to 2-substituted cyanoacrylates, 3-substituted cyanoacrylates that are formed in the reaction do not polymerize. The amounts of zwitterions 20 (Scheme 7) that remain intact in the solutions are listed in Table 2.
Remaining fraction (in %) of zwitterions 20 after their storage in different solvents at room temperature (Unless stated otherwise, R is the substituent at the para-position of phenyl-group; E is dielectric permeability [18,22]).
R/Solvent | H | OH | OCH3 | Br | m-NO2 | NO2 | E |
CH3CN | 91.3 | 98.8 | 96.9 | 100 | 100 | 100 | 37.5 |
Me2SO2 | 87.9 | 94.3 | 95.9 | 97.7 | 96.8 | 98.2 | 48.9 |
(CH3)2CO | 40 | 21.6 | 30.7 | 21.4 | 27.9 | 29.2 | 20.70 |
CHCl3 | 1.1 | 3.1 | 1.7 | 2.5 | 2.8 | 3.3 | 4.806 |
THF | 0 | 3.8 | 1.3 | 2.6 | 2.2 | 3.1 | 7.4 |
4.2 Reactivity of type one cyano-1,3-P-zwitterions 17
4.2.1 Protonation of zwitterion 17a
Zwitterions 17 can be easily protonated. For example, reaction of zwitterion 17a with CF3SO3H formed in situ from CF3SO2SiMe3 in wet acetone leads to salt 21 (Scheme 9) [20].

Compounds 17a and 21 provide a good example of how protonation of the central carbon atom of cyano-1,3-P-zwitterions affects their spectral characteristics. Comparison of 1H NMR spectrum of compounds 17a and 21 shows that protonation of the negative charge in 17a results in the 5–6 ppm upfield shift for all protons.
4.2.2 Alkylation: reaction of zwitterions 17 with alkylhalogens
Zwitterions 17 are nucleophiles that are strong enough to be alkylated by alkyl halides as shown in Scheme 10 [23].

Alkyl halides alkylate cyano-1,3-P-zwitterions 17 at the central atom of the NCCCO pentade with the formation of salts 22. In IR spectra of the reaction mixture, characteristic zwitterion band disappears to give way to absorption bands at 1730–1750 cm−1 and 2240 cm−1 caused by oscillations of the ester and nonconjugated nitrile moieties, respectively. In the case of the zwitterion formed by i-Pr3P, reaction of this zwitterion with 1,3-dichloroacetone links two zwitterions together resulting in molecule 23 bearing two positive charges.
4.2.3 Arylation: reactivity of zwitterion 17a toward 2,4-dinitro- and 2,4,6-trinitrofluorobenzenes
Reactions above occur at the central carbon atom of the NCCCO skeleton. It would be reasonable to assume that due to electron delocalization other nucleophilic atoms of the NCCCO skeleton may also serve as nucleophiles. As it is shown below, reaction of zwitterion 17a with such polynitrofluorobenzenes as 2,4-dinitrofluorobenzene (DNFB) and 2,4,6-trinitrofluorobenzene (TNFB) may result in either C- or N-derivatives depending on the structure of the polynitrofluorobenzene and the temperature for reaction with DNFB.
Depending on the temperature, zwitterion 17a reacts with DNFB via different pathways (Scheme 11).

At −15 °C, the reaction occurs predominantly according to the traditional pathway (“a”) with the formation of new aromatic compound 24. On the contrary, at room temperature, unexpected pathway “b” takes place that gives compound 25. Structure of compound 25 was established by IR, NMR (1H, 13C, 31P) spectroscopy and X-ray diffraction analysis [24]. The most feasible mechanism includes the rupture of the weak CC bond between the α-C atom and the carbonyl group in the α-C–C(O)OEt moiety [25] upon breakdown of σ-complex 26. It is noteworthy that at room temperature compound 24 does not turn into compound 25. This suggests formation of 25 directly from σ-complex 26.
However, contrary to the reaction between zwitterion 17a and DNFB, the same zwitterion reacts with TNFB with the formation of N-derivative 27 [26] (pathway “c”). At 3 °C, according to the 31P and 19F data, the reaction between zwitterion 17a and TNFB in methylene chloride quantitatively affords the only product, i.e., crystalline dark-red compound 27. Structure of this compound was established by a single-crystal X-ray diffraction analysis. In all likelihood, the reaction pathway follows Scheme 11. Zwitterion 17a may be depicted as a sum of two resonance forms, one with the negative charge at the carbon atom, and another with the negative charge at the nitrogen atom of the cyano-group. Reaction of TNFB with this nitrogen atom results in the formation of compound 27 most likely directly upon breakdown of σ-complex 28. Differences between reaction pathways for reaction of zwitterion 17a with DNFB and that with TNFB can be explained by the steric effect of the second ortho-NO2-group. Clearly, the approach of the C-anionic center of zwitterion 17a to the CF bond should be sterically hindered by the second ortho-NO2-group. At the same time, the nitrogen atom of the N-resonance form of this zwitterion should lack these steric interferences. This reaction may also include initial formation of unstable dead-end C-σ-complexes.
4.2.4 Formation of carbamate 1,3-P-zwitterions (cyano-1,3-P-zwitterions of type two)
Zwitterions 17 react with unsubstituted phenyl, orto-, meta- and para-substituted phenylisocyanates, 1-naphtyl isocyanate and phenylisothioisocyanate 29 to give carbamates 30 [9,27,28] (Scheme 12).

Mechanism of this reaction has been studied spectrophotometrically by following its kinetics in a number of solvents at temperatures between 20 °C and 60 °C. It was established that the reaction followed the first order with respect to each reagent; the reaction is second order overall. Most likely, the reaction follows the mechanism shown in Scheme 13 [29].
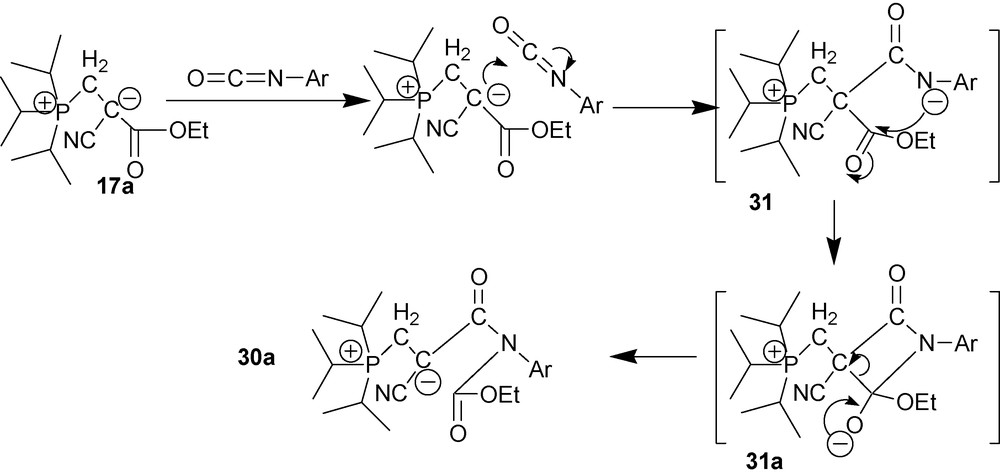
As shown in Scheme 13, the reaction follows an unusual pathway: a carbanion rather than the ethoxy anion (as in the case of ester aminolysis) acts as the leaving group. Apparently, the unique situation in which breaking a CC-bond is preferred over breaking a CO bond is due to the energetic gain from the more effective delocalization of the anionic charge in carbamate 30a being formed.
The rate of the reaction between zwitterion 17a and ArNCO strongly depends on the volume of ortho-substituent [30]. According to the 31P NMR data, the insertion of the isocyanate moiety into the CC bond of zwitterion 17a in the reactions with ortho-substituted phenyl isocyanates proceeds slower that the same reactions with phenyl unsubstituted isocyanates. Consequently, bulky ortho-substituents create substantial hindrances during the reaction. Most likely, these steric hidrances have particluar effects during nucleophilic substitution at the C-atom of the ethoxycarbonyl group of 31 [31]. X-ray diffraction studies confirm that in carbamate 30a ortho-substituents and ester group are at a short distances from each other [31]. In its turn, this overcrowding in the vicinity of the carbamate carbonyl group created by the isocyanate ortho-substituent at the aromatic ring leads to the hindered rotation around the NCAr bond and, as a consequence, to the emergence of chirality as 1H NMR data show [31,32] (atropisomerism for 30a with Ar having bulky ortho-substituents).
4.2.5 Reactions of zwitterions 17 with Iso(thio)cyanates not leading to carbamates
Not all iso(thio)cyanates form carbamates upon reaction with zwitterions 17. Exceptions to Scheme 12 were studied using zwitterion 17a. As shown below, zwitterion 17a reacts differently with highly electrophilic tosyl-isocyanate and extremely sterically hindered arylisothiocyanates.
4.2.5.1 Reaction of zwitterion 17a with tosylisocyanate
Reaction of zwitterion 17a with highly electrophilic tosyl isocyanate leads to derivative 32 [20] (Scheme 14). 1H and 31P NMR data show that at the first moment tosylisocyanate reacts with zwitterion 17a with the formation of compound 33. However, negatively charged nitrogen atom possesses negligible nucleophilicity due to highly electron withdrawing tosyl-group. Therefore decomposition of adduct 33 cannot proceed according to the mechanism shown in Scheme 13. Since formation of 33 from zwitterion 17a with tosylisocyanate is a reversible reaction, with time the overall equilibrium completely shifts to compound 32 due to irreversible polymerization of cyanoacrylate 5.

4.2.5.2 Reactions of zwitterion 17a with 2,6-dichloro- and 2,4,6-trichloroisothiocyanates
Zwitterion 17a reacts with these isocyanates according to Scheme 15 that also does not lead to carbamates [33]. This reaction gives P,N-zwitterion 34 and the cyanoacrylate polymer following the pathway that is similar to that with tosyl isocyanate (Scheme 15). However, contrary to the reaction with tosylisocyanate when electronic factors are the most likely major reasons for such a pathway, in the case of 2,6-dichloro- and 2,4,6-trichloroisothiocyanates steric factors probably play the major role [30]. The electron withdrawing effect of the chlorine atoms in the phenyl ring that should decrease nucleophilicity of the negatively charged nitrogen atom in 35 may also contribute to the overall effect.

4.2.6 Reaction of zwitterion 17a with C,N-diphenylnitrilimine
Reaction of zwitterion 17a with C,N-diphenylnitrilimine 36 in MeCN results in the formation of 2-pyrazoline 37. This is the first known example of a pyrazoline that bears phosphonium group (Scheme 16) [34].

.
However, the yield of pyrazoline 37 is just about 10%. The reason for such a low yield is that in this case zwitterion 17a serves not only as latent i-Pr3P but also as latent ethyl-2-cyanoacrylate 5. Both these compounds react with C,N-diphenylnitrilimine 36. As a result of these side reactions, corresponding phosphonium salt 38 and ethyl-1,3-diphenyl-5-cyano-4,5-dihydro-1H-pyrazole-5-carboxylate 39 is formed (Scheme 17).

4.2.7 Zwitterions 17a as the source of tri-iso-propylphosphine
As it was discussed above, formation of cyano-1,3-P-zwitterions is a reversible reaction between phosphines and alkyl-2-cyanoacrylates. Therefore, in some cases these zwitterions can serve as latent phosphines. For example, zwitterion 17a serves as a source of tri-iso-propylphosphine in the reactions with HgCl2 [33], TsN3 [20], TsNCO and 2.6-Br2C6H3NCO [33]. The products of the reactions are the same as with free i-Pr3P. Reactions are accompanied by the formation of the cyanoacrylate polymer.
5 Type two cyano-1,3-P-zwitterions and their physicochemical characteristics
Table 3 lists spectral properties and melting points of type two cyano-1,3-P-zwitterions.
Spectral properties and melting points of cyano-1,3-P-zwitterions 30 [30].
Zwitterion 30 | M.p. (°C) | IR (υ, cm−1) | 31P NMR (ppm) | Lit. | ||||
R | R′ | Alk | X | CN | C(O)OR5 | |||
Pr | Ph | Me | O | 36–37 | 2172 | 1718 | 34.1 | [9,28] |
Pr | Ph | Et | O | 141–142 | 2155 | 1715 | 34.0 | [9,28] |
Bu | Ph | Et | O | 70–72 | 2158 | 1695 | 35.2 | [9,28] |
Et2N | Ph | Et | O | Dense oil | 2165 | 1715 | 56.1 | [9,28] |
i-Pr | Ph | Et | S | 146–147 | 2185 | 1720 | 44.38 | [27] |
i-Pr | Ph | Et | O | 171–171.5 | 41.35 | [20] | ||
i-Pr | Me | Me | O | 163–164 | 2158 | 1690 | 41.1 | [20] |
i-Pr | o-FC6H4 | Et | O | 145–146 | 2158 | 1685 | 40.97 | [30] |
i-Pr | o-ClC6H4 | Et | O | 169–170 | – | – | 41.15 | [30] |
i-Pr | o-BrC6H4 | Et | O | 170–171 | 2143 | 1619 | 40.86 | [30] |
i-Pr | o-IC6H4 | Et | O | 170–170.5 | 2160 | 1621 | 41.04 | [30] |
i-Pr | o-MeC6H4 | Et | O | 177–178 | 2138 | 1598 | 41.33 | [30] |
i-Pr | o-EtC6H4 | Et | O | 151–151.5 | 2140 | 1608 | 41.03 | [30] |
i-Pr | o-i-PrC6H4 | Et | O | 164–165 | 2140 | 1597 | 41.01 | [30] |
i-Pr | o-PhC6H4 | Et | O | 106–107 | 2133 | 1601 | 36.4 | [30] |
i-Pr | o-CF3C6H4 | Et | O | 110–111 | – | – | 40.98 (19F NMR, 60.3) | [30] |
i-Pr | o-MeO-C6H4 | Et | O | 173–174 | – | – | 40.97 | [30] |
i-Pr | 1-Naphthyl | Et | O | 180–181 | 2170 | 1715 | 41.12 | [27] |
i-Pr | o,p-Me2C6H3 | Et | O | 171–171.5 | 2175 | 1718 | 41.01 | [31] |
i-Pr | p-ClC6H4 | Et | O | 142–143 | 2170 | 1720 | 41.59 | [27] |
i-Pr | m,p-Cl2C6H3 | Et | O | 169–170 | 2175 | 1730 | 41.63 | [27] |
i-Pr | p-NO2C6H4 | Et | O | 157–158 | 2180 | 1725 | 41.49 | [27] |
i-Pr | Ph | CH2C≡CH | O | 122–124 | 2160 | 1621 | 39.18 | [35] |
i-Pr | 1-Naphthyl | CH2CHCH2 | O | 123–124 | 2143 | 1619 | 38.81 | [35] |
In general, zwitterions 17 and 30 share many common features but chemical and physical properties of 30 suggest even higher degree of delocalization of the negative charge in the NCCCO skeleton than in 17 [20,27]. Higher degree of delocalization of the negative charge result, on one hand, in a weaker overall nucleophile reactivity of zwitterions 30 as compared to zwitterions 17. This is why zwitterions 30 can serve as nucleophiles with very strong electrophiles only, such as acids [36], methyl triflate [36] and TNFB [37,38]. On the other hand, higher degree of delocalization of the negative charge in the NCCCO pentade results, as it is evidenced from examples below, in triple nucleophile reactivity of this pentade as illustrated in Scheme 18.

6 Chemical properties of type two cyano-1,3-P-zwitterions 30
6.1 Storage stability of type two cyano-1,3-P-zwitterions 30
Cyano-1,3-P-zwitterions of type two are stable compounds. At room temperature, they can be stored in solid form practically indefinitely.
6.2 Reactivity of type two cyano-1,3-P-zwitterions 30
6.2.1 C-protonation of type two cyano-1,3-P-Zwitterions 30
Reaction of zwitterions 30 with unhydrous acids results in C-protonation [36] (Scheme 19).

6.2.2 O- and S-methylation of type two cyano-1,3-P-zwitterions 30
Methylation of zwitterions 30 requires the use of such a strong methylating reagent as methyl trifluoromethanesulfonate (methyl triflate) (Scheme 20) [36].

6.2.3 N-Arylation of type two cyano-1,3-P-zwitterions 30
Reactions of zwitterions 30 with TNFB lead to N-derivatives (Scheme 21) [37,38].

This is the first known example of N-arylation of cyano-carboanions. Zwitterions 30 react under mild conditions in methylene chloride with the formation of either substituted oxazine-2,4-dione 42 [37] or isomeric barbiturate 43 [38]. The first pathway occurs if aryl substituent at the nitrogen atom of the carbamate moiety has ortho-ethyl group. Most likely, the latter hinders conversion of oxazine into barbiturate. This suggestion is supported by the fact that zwitterions 30 with unsubstituted phenyl group at the nitrogen atom of the carbamate moiety reacts with TNFB with the formation of barbiturate 43. Structures of the products 42 and 43 were confirmed with X-ray diffraction analysis.
7 Conclusions
The review summarizes results that were obtained in a very interesting field of phosphorus-containing zwitterions of the new types. Reactions that were discovered for these zwitterions are also typical for other non-phosphorus carbanions. For example, carbanion derivatives of cyanoacrylic acid esters [39] also react with isocyanates with insertion of the isocyanate molecule at the C-C-bond with the formation of carbamates. Arylation of carbanions derivative of cyanoacrylic acid esters [24] and cyanoacrylic acid amides [40] with polynitrohalobenzenes gives negatively charged conjugated structures instead of aromatic compounds. Unusual breaking the CC-bond that was observed in the case of formation of 25 according to Scheme 11 occurs also in the reaction of cycloimmonium ylides with TNFB [41]. Therefore, the scope of P-zwitterion reactions discussed in this review is beyond of just this specific field of phosphorus chemistry and can be the foundation for expansion of frontiers of organic chemistry in general.
Acknowledgements
Yu.G.Gololobov is grateful to the Russian Foundation for Basic Research (Project 08-03-00196a).