Agriculture leads to the production of many by-products. Hemicelluloses obtained from the straw of cereals enable to get low-cost molecules such as d-xylose and l-arabinose, which can be valorized. Since many years, we are involved in a regional program in charge of promoting renewable biosources in the field of surfactants and using both pentoses as starting material.
1 Telomerization reactions
At first, we built some surfactants from the telomerization of butadiene with d-xylose and l-arabinose as nucleophiles. Since its discovery in 1967 [1], the palladium catalysed telomerization of butadiene, in the presence of alcohols or phenols as nucleophiles, has been extensively studied and applied to a variety of other nucleophiles [2]. The reaction constitutes an elegant method to provide a large range of functionalised compounds, which can be used as building blocks for fine chemicals for industrial applications [2–4]. Due to the increasing importance of carbohydrates as cheap and renewable starting material, the use of these compounds as nucleophiles in telomerization is of great interest in regard to the production of biodegradable non-ionic surfactants [5]. Tetraacetylated galactose has been reported as the first carbohydrate derivative used for the telomerization with butadiene on the anomeric position [6]. Later on, the subject has been largely studied from both academic and industrial aspects: the telomerization was carried out using free sugars, mainly sucrose and also glucose or its derivatives and the reaction was developed in organic (mainly isopropanol mixtures) [7,8] or aqueous medium [9–11]. The catalyst system is built from palladium salts (especially Pd(acac)2 or Pd(OAc)2) associated to a phosphine which was hydrosoluble when processes were performed in water [9–11]. Different variations have been made on the reaction conditions allowing to reach high efficiency [8] but whatever the medium, the transformation led to a complex mixture of polyethers. The best selectivity to monooctadienyl ether of sucrose was reported in using aqueous NaOH and isopropanol as a cosolvent leading to an average degree of etherification of 1.3 [10]. However, the process described in aqueous NaOH was not compatible with reducing sugars such as aldoses [9,10]; furthermore, when the solvent contains an alcohol, this latter competes with the sugar as nucleophilic species [7,8]. The mechanism of telomerization of butadiene with methanol, established by the careful studies of several German teams [12–14], has been supported by density functional calculations (DFT) [15] (Scheme 1).
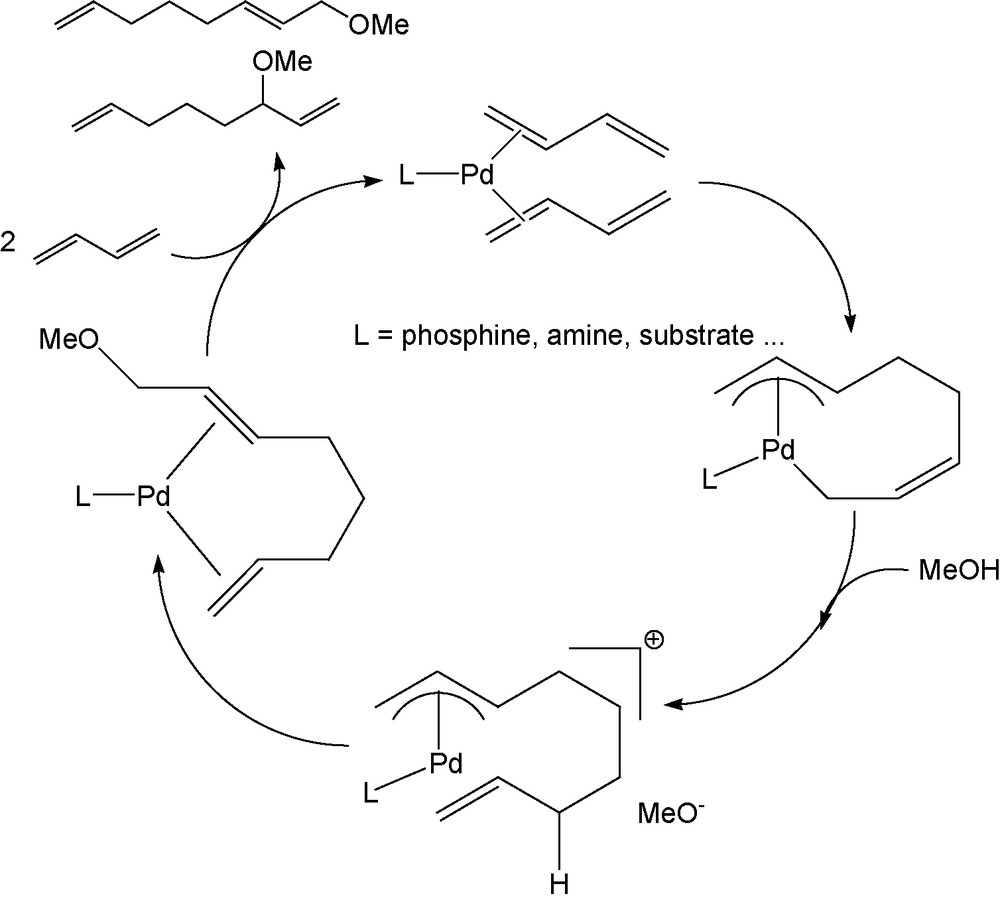
Mechanism for the telomerization of butadiene with MeOH [13].
The parameters governing the regioselectivity of the chain grafting are quite well controlled with nucleophiles like methanol [13] or ammonia [16]. The proportion of mono- or polysubstitution by the octadienyl chain has only been studied starting from bifunctional active hydrogen compounds: a high selectivity to monosubstituted linear telomer has for example been achieved from ethylene glycol in biphasic systems [17] or in using polymer-bound palladium(0) complexes as catalysts [18].
For the valorisation of pentoses, mainly l-arabinose and d-xylose, which are now easily extracted from wheat straw and bran [19], the telomerization reaction constitutes an attractive route to prepare monoethers directly without the use of protection and deprotection steps (Scheme 2).
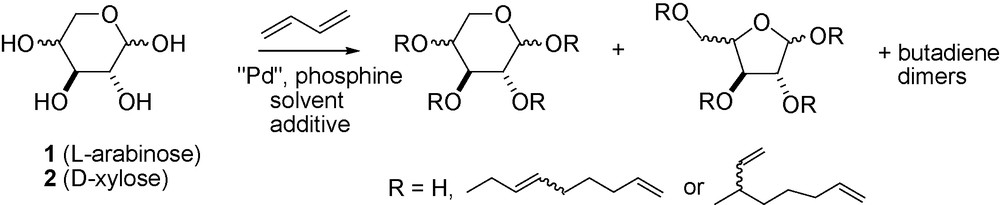
Telomerization reaction of butadiene with l-arabinose and d-xylose.
By optimisation of the experimental conditions, we succeeded in the selective glycosylation of free pentoses via a Pd-catalyzed telomerization of butadiene in dimethylformamide DMF [20]. The catalytic system has been modified by adjusted quantities of various amines [21] and phosphines, the latter having medium donating properties.
Best conditions mentioned on Scheme 3 require a high concentration of sugar [21] in the medium and a simple catalytic system based on Pd(OAc)2 and PPh3.

Telomerization of butadiene with l-arabinose and d-xylose in dimethylformamide.
The process did not require any activation or protection of the sugar or the glycosyl acceptor and led to surfactant molecules having a non-ionic and biodegradable head [20a].
The structures of the major adducts (Fig. 1) have been established after their transformation in peracetylated derivatives, followed by purification. These products have been compared with thoses resulting from the telomerization of butadiene with 2,3,4-tri-O-acetylated pentopyranoses [22] and peracetylated l-arabinose and d-xylose [23].
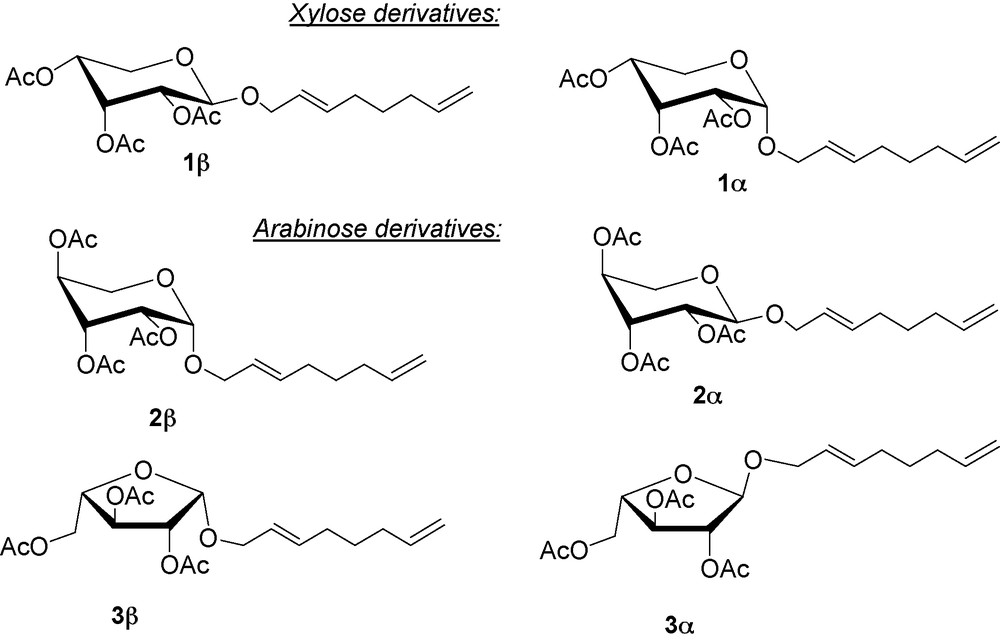
Structures of the major compounds resulting of telomerization in dimethylformamide after their acetylation.
The major pentosides consist of about 50% of 2,3,4-tri-O-acetyl-2’-(E)-7’-octadienyl-α-d-xylopyranoside 1α and 26% of 2,3,4-tri-O-acetyl-2’-(E)-7’-octadienyl-β-d-xylopyranoside 1β starting from d-xylose and 39% of 2,3,4-tri-O-acetyl-2’-(E)-7’-octadienyl-α-d-arabinopyranoside 2α, 22% of 2,3,4-tri-O-acetyl-2’-(E)-7’-octadienyl-β-d-arabinopyranoside 2β, 4% of 2,3,4-tri-O-acetyl-2’-(E)-7’-octadienyl-α-d-arabinofuranoside 3α and 4% of 2,3,4-tri-O-acetyl-2’-(E)-7’-octadienyl-β-d-arabinofuranoside 3β starting from l-arabinose. It appears that the major glycosylation occurred at the anomeric hydroxyl, which is probably due to its higher acidity compared to other hydroxyl groups. Indeed, the anomeric hydroxyl group (pKa ≈ 12) is more acidic than simple alcohols (pKa ≈ 16) due to inductive electron withdrawal by endocyclic oxygen [24]. As a consequence, the anomeric proton was more operative during the key step of protonation of the intermediate (η1,η3-octadienyl)palladium complex [12,13], inducing the observed selectivities. The xylose derivatives adopted the major “all equatorial” pyranosic form, while the furanosic forms were also present in arabinose derivatives. As previously discussed [23] the ratios of the glycosides tautomers are different from those in starting material.
Next, telomerization of butadiene with the two pentoses was performed in water. The use of raw sugars as telogens is of great interest due to the direct transformation of these renewable materials into biodegradable non-ionic surfactants [25]. Telomerization reactions have thus been carried out with glucose or its derivatives [7], sucrose [8–11] and even with polysaccharides such as starch [11b]. However, with these nucleophiles, the situation is much more complicated, due to the existence of tautomers adding to the multiplication of reactive sites. Nevertheless, conditions have been described to carry out the selective monoetherification of sucrose in isopropanol–water mixtures [10,11] and we have previously disclosed the selective grafting of one octadienyl chain on the anomeric hydroxyl of l-arabinose and d-xylose in DMF [20b].
Next, in order to develop greener methodologies, we have continued our studies in aqueous media and we wish to report here conditions which give the selective etherification of the above pentoses with one or two octadienyl chains. The telomerization of butadiene with pentoses can be carried out in an aqueous medium using Pd(acac)2–TPPTS (TPPTS = triphenylphosphine trisulfonate sodium salt, P(m-C6H4SO3Na)3 [26,27] as a catalytic precursor and a tertiary amine as a promoter. A simple variation of the experimental conditions allowed us to prepare two classes of surfactant molecules having a different hydrophilic–lipophilic balance. The choice of the amine was crucial with regard to the control of the octadienyl chain grafting. With a crowded amine like ethyldiisopropylamine, the monooctadienylpentosides were the major products, especially in the presence of isobutylmethylketone as cosolvent. With the amphiphilic dimethyldodecylamine, a second grafting occurred which afforded a mixture of dioctadienylpentosides.
After acetylation and laborious separation of crude mixtures, the major diethers from d-xylose consisted of three compounds in similar quantities (about 25% of 4β, 25% of 5β and 30% of 6β (Fig. 2) as well as unidentified isomers in small amounts. These compounds were identified by comparison of their NMR spectra with those of the peracetylated starting sugars [29] and monooctadienylpentosides [20b,23]. As previously observed for the telomerization of butadiene with pentoses in DMF [20b], the first grafting mainly occurred at the anomeric hydroxyl, probably due to its higher acidity compared to that of the other groups [30]. The second etherification took place on the other hydroxyls of comparable acidity with similar rates leading to a similar distribution for 4, 5 and 6 (Scheme 4).

Structures of the major compounds resulting of telomerization in water of butadiene with d-xylose after their acetylation.
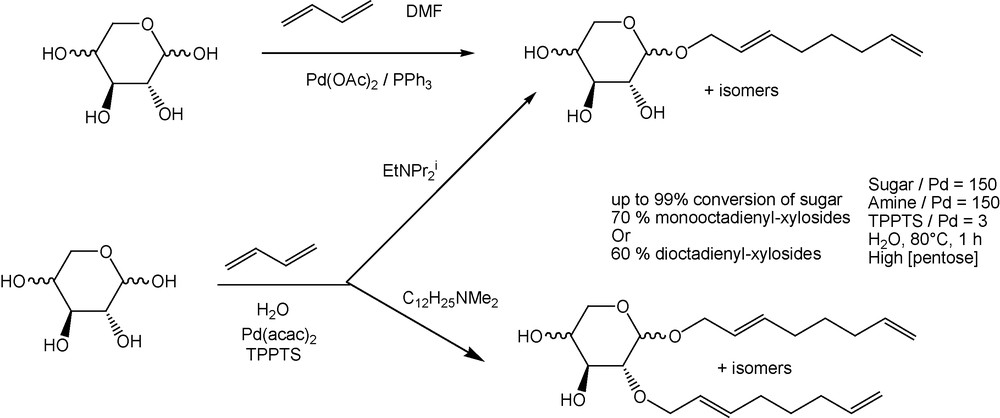
Telomerization of butadiene with l-arabinose and d-xylose in water [28].
The identified xylose derivatives were all in a pyranose form with substituents in an equatorial position and with an (E)-configuration of the double bond for the hydrophobic chains. It is essential to know the configuration of the double bond for further derivatization of such compounds through epoxidation or dihydroxylation.
After thorough separations of major synthesized products and deacetylation in basic medium, the study of their surface activity showed their interesting capacity of lowering surface tension down to 30–35 mN/m at relatively low concentrations in the range of 1–4 mmol/L (Table 1) [31]. No CMC value could be established for xyloside 1β (β anomer) compared to the α anomer analogue which exhibits a clear amphiphilic behavior. Similarly to alkyl glucosides [32][32a], the α anomers showed stronger surface activity than the β anomers; these observations are in accordance with the fact that among the alkyl glucosides α-anomers are less hydrophilic than β-anomers involving different thermotropic properties [32b].
Surfactant properties of mono- (1β,1α,2α) and di-octadienyl (D) ethers and mixtures.
Entry | Compounds | CMCa | γCMCa | amina | |
(mg/L) (mmol/L) | |||||
1 | 2’-(E)-7’-octadienyl-β-d-xylopyranoside 1β | – | – | 36b | – |
2 | 2’-(E)-7’-octadienyl-α-d-xylopyranoside 1α | 500 | 1.94 | 31 | 41.5 |
3 | 2’-(E)-7’-octadienyl-α-l-arabinopyranoside 3 | 180 | 0.70 | 34 | 43.0 |
4 | Xylosyl monooctadienyl ethers (90% 1β, 10% 1α) | – | – | 33.5c | – |
5 | Xylosyl dioctadienyl ethers D | 70 | 0.19 | 35 | 57.5 |
6 | Crude mixture of xylosyl telomersd | 55 | 0.19 | 35 | – |
a CMC = critical micellar concentration, γCMC = surface tension at CMC, a = area of polar head per molecule.
b Above 1000 mg/L, the value of surface tension tends to slowly level off but the solution becomes turbid so that the existence of a true CMC is questionable.
c Surface tension and area determined at a concentration of 1 mmol//L.
d Composition of the crude mixture: 6% xylose, 62% xylosyl monooctadienyl ethers, 27% xylosyl dioctadienyl ethers, 5% xylosyl trioctadienyl ethers (composition evaluated by GC).
Submitting crude mixtures of telomers of xylose (Fig. 2) or bran syrup (Fig. 3) to the same examination established that the surface-activity was not improved after the separation process. Therefore, for an industrial application of these surfactants, the use of the lower cost, non-separated mixture can be recommended.
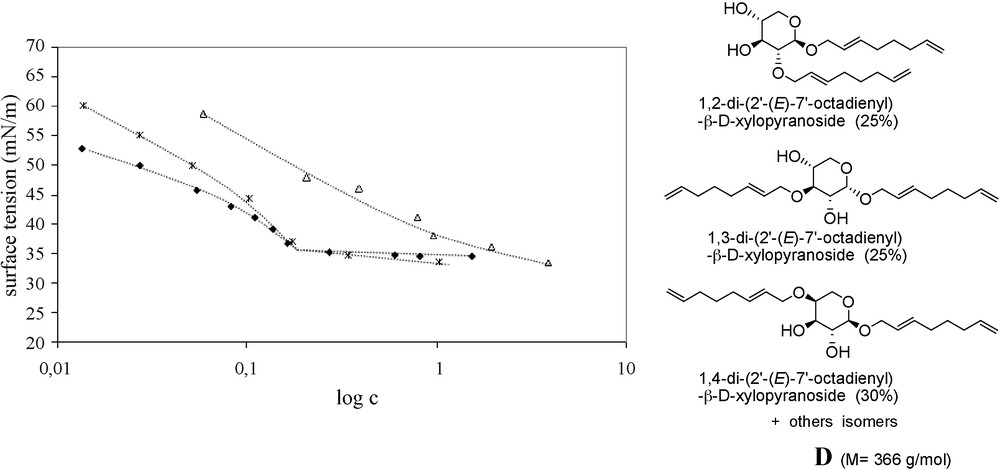
Surface tension of the telomer mixtures. Δ: xylosyl monooctadienyl ethers (1β + 1α); ♦: xylosyl dioctadienyl ethers (D); *: crude mixture.
Since another advantage of the biphasic systems could be the immobilisation of the active catalyst, we looked for recycling possibilities with Me2NC12H25 or KF/Al2O3 as a heterogeneous base. Only one recycling was possible when using KF/Al2O3, which could be explained by partial destruction of the catalyst, since a precipitate of palladium black was observed (Fig. 4).
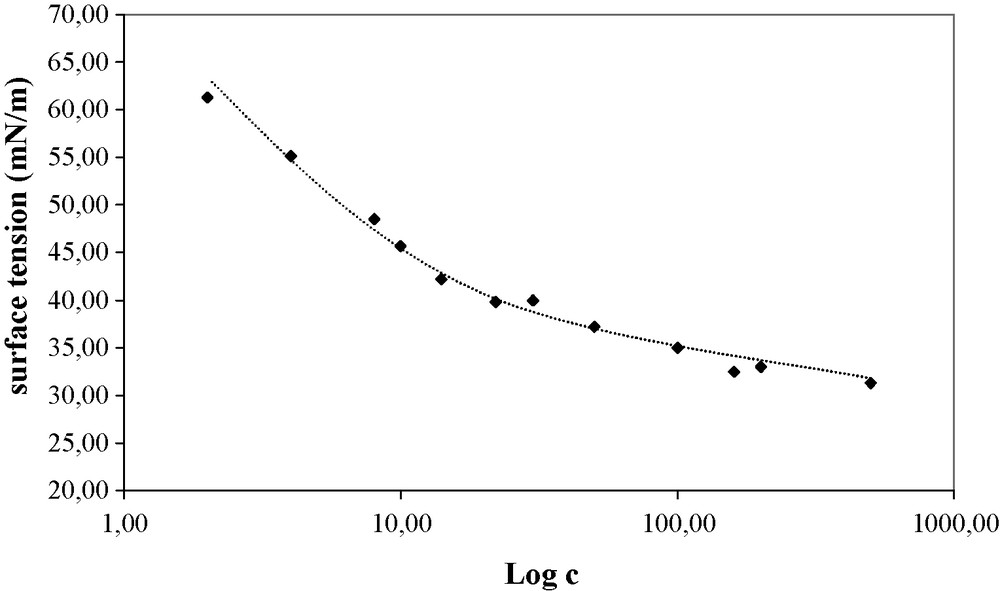
Surface tension of a crude telomer mixture issued from the telomerization of butadiene with bran syrup as a function of concentration. Composition of the bran syrup 99.5% purity: 72.6% dry material (DM), 33.5% l-Arabinose/DM, 54.2% d-Xylose/DM, 8.9% d-Glucose/DM, 1.7% d-Galactose + d-Mannose/DM, 1.2% Polysaccharides/DM. Composition of the telomer mixture: 1% bran syrup, 67% monooctadienylethers, 31% dioctadienylethers and 1% trioctadienylethers.
In the same time, we developed an efficient heterogeneous Pd-TPPTS–KF/Al2O3 catalyst system, in telomerization reactions of butadiene with MeOH and PhOH [33], which could be easy to recover and reuse 5 to 8 times, without loss of its activity. This catalytic system was prepared according analogous procedures described by Sinou and al. for Pd-phosphane catalyst immobilised on silica under “supported aqueous” phase conditions [34], this kind of catalyst being effective for Tsuji-Trost allylic substitutions. Compared to previous work involving a Pd-complex with a 15-membered macrocyclic triolefin Ma [35], the heterogeneous support behaves as the macrocycle Ma in solution, trapping and stabilising an active low valence-palladium species. This palladium-TPPTS catalyst heterogenized on KF/alumina has been also shown to be effective and recyclable for the selective formation of monooctadienylxylopyranosides via the telomerization of butadiene with d-xylose [36] (Fig. 5).

Telomerization of butadiene with d-xylose using Palladium-TPPTS catalyst heterogenized on KF/alumina.
These telomerizations join well the concept of green chemistry because they are good examples of atom efficient reaction. Furthermore, The feedstock is inexpensive, the reaction can be 100% atom efficient and the products are valuable, solvent is water and a novel solid catalyst system is shown to be active, recoverable and recyclable.
The transformation of the telomers was then directed respectively to the functionalisation of the polar head or the hydrophobic chain.
At first, the functionalisation of the polar head was realized by phosphorylation of the free OH remaining on the sugar head. In fact, phosphates surfactants are well known as self-assembling molecules [37] and for their surface-active properties in metal-working industry or as emulsifiers in plant protection formulations [38], but sugar-based phosphate surface-active compounds are not commonly used. Phosphorylation on sugars is largely described in the literature [39] and it appears that the reaction takes place most of the time on the the anomeric position. Among various conditions described in the literature [40], we adapted the process to our telomers as described on Scheme 5 [41].
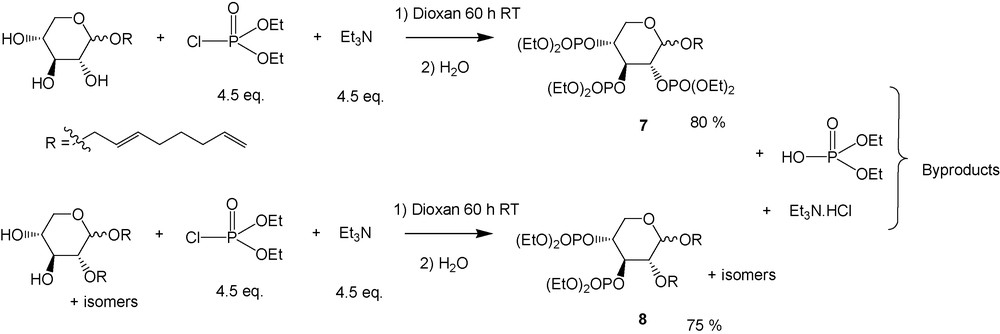
Phosphorylation of monooctadienyl-d-xyloside and of a mixture of dioctadienyl xylosides.
Next, hydrolysis of the ethoxy phosphate groups according to the Mewly and Vasella [39a] conditions led to phosphate derivatives (Scheme 6) with good yields [41].
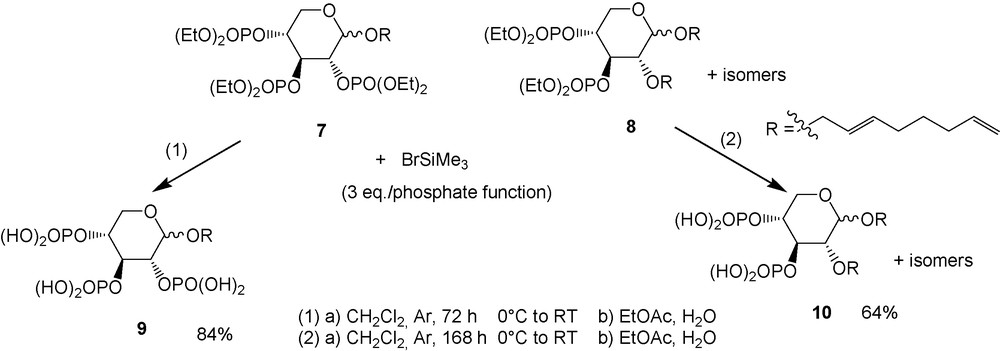
Hydrolysis of ethoxy phosphate functions.
Surfactant properties of mono- and diphosphate derivatives are given in Table 2. The mono and dioctadienyl ethoxy phosphate xyloside derivatives obtained from renewable resources, display good surfactant properties and could be used as crude mixtures in industrial applications. In contrary, phosphate derivatives are characterized by a very high hydrophilicity and a poor surface activity.
Surfactant properties of mono- and di-octadienylxyloside phosphate derivatives.
Entry | Compounds | CMC | CMC | γCMC |
(mg/L) | (mmol/L) | (mN/m) | ||
1 | 200 | 0.34 | 37 | |
2 | 100 | 0.16 | 36 | |
3 | 2160 | 4.3 | 47 | |
4 | 1200 | 2.3 | 58 |
So, phosphorylation of mono- or dioctadienyl-d-xylosides led to mono or di-ethoxy phosphate or phosphate derivatives; it was found that the introduction of these functional groups influences as well the solubility and the surfactant properties of the mono- or dioctadienyl-d-xylosides [39].
Next, concerning the transformation of the hydrophobic chain, we chose to study its reactivity towards metathesis conditions because our original purpose was to prepare easily some bolaform surfactants.
Bolaforms with carbohydrate heads connected by a long hydrocarbon tether can have interesting micellar or surfactant properties [42]. This led us to try to convert 1β into the corresponding bolaform using a metathesis reaction mediated by Grubbs catalysts 11 [43] and 12 [44] (Fig. 6).
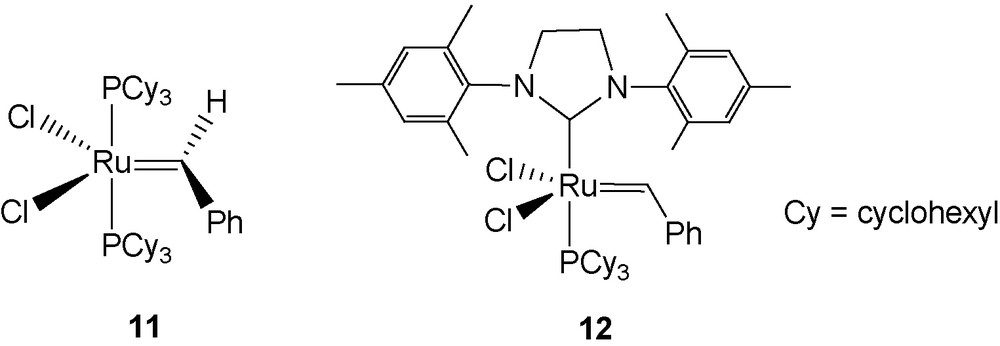
Grubbs catalysts.
The reaction of the octadienylether of xylosyle and of its peracetylated form in the presence of Grubbs catalysts has led to O-allyl carbohydrates, and from the protected carbohydrate, to the bolaform with a C4 tether with high yields (Scheme 7) [45]. This study was in agreement with preliminary metathesis work on 1-phenoxy-2,7-octadiene where metathesis of the internal double bond of 1-phenoxy-2,7-octadiene was found to be mediated by Grubbs catalysts, while o-substituted phenols were obtained in the presence of rhenium or tungsten-based catalysts [46]. Mechanistic studies led us to propose various reaction ways depending both on the nature of the catalyst and on the starting substrate.
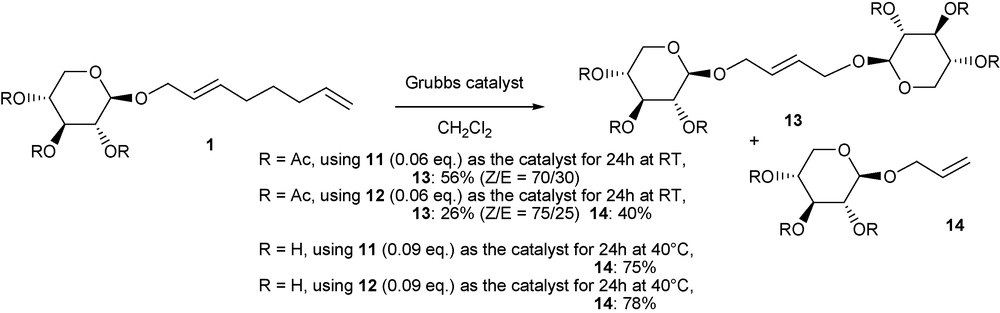
Metathesis reactions on monooctadienyl-d-xyloside.
2 Glycosylation and esterification reactions
Other methods to graft a hydrophobic chain on a sugar entity could be the classical reaction of glycosylation or the esterification one.
Alkenyl glucosides have been reported in the literature during recent years: C3–C9 alkenyl 2,3-unsaturated glucosides from glucose and alkenols by using Ferrier reaction [47a]; terminal unsaturated C3–C11 alkenyl glucopyranosides [47b]; galactopyranosides through cross-metathesis reaction of allyl galactopyranosides and olefins [48c]; C4–C22 alk(en)yl glucosides in admixtures [47d] and undecenyl glucopyranosides for the preparation of monolayers on silica surface [47e]. However, to our knowledge, no pure pentosides with an unsaturated terminal chain together with their surfactant properties were yet described. Since fatty esters are of great importance in cosmetics because of large skin and eye tolerance [48] we have also prepared a fatty ester of d-xylose in anomeric position.
Glycosylation of d-xylose has been performed with hex-5-enol, dec-9-enol and undec-10-enol using three different acidic catalysts: Amberlite IR 120-(H+) resin [49], p-toluenesulfonic acid (PTSA) [50] and acetyl chloride (AcCl) [51]. Several experimental conditions were explored with the three different fatty alcohols (Scheme 8) [52], but considering industrial processes of glycosylation of hexoses using PTSA as the acidic source [53], we explored an original work-up procedure.

Glycosylation on d-xylose.
After heating the d-xylose (1 g) at 80 °C for 24 h with 0.6 equiv of PTSA using undec-10-enol as solvent, the crude reaction mixture was distilled at 130 °C under reduced pressure to remove the alcohol. The residue was taken up with 1:50 EtOAc–water to separate residual xylose and PTSA from the pentoside. Evaporation of ethyl acetate afforded the xyloside (70%).
Direct and anomeric selective esterification was not possible. Therefore, esterification of (2,3,4-tri-O-acetyl)-d-xylopyranose [54] and 2,3,4-tri-O-benzyl-d-xylopyranose [55] was performed with undecenoyl chloride in the presence of triethylamine in refluxing CH2Cl2 (Scheme 9). Deprotection of the acetylated ester using various conditions (NaOMe or NaHCO3 in MeOH) led unfortunately to xylose and methyl undecenoate, even when using triethyl-amine in water as described by Bliard and al. for specific deprotection [56]. In contrast, deprotection of benzylated ester by Pd-catalyzed hydrogenolysis was very efficient and involved simultaneously the double bond hydrogenation, the two anomeric forms being unseparable by classical silica gel chromatography.

Esterifications on d-xylose derivatives [52].
1-O-Alkenoyl-d-xylose and alkenyl d-xylosides exhibit interesting surface active properties (Table 3). The ester derivative is characterized by a decrease in surface tension, which is lower than the surface tension generally obtained with sugar esters and displays interesting foaming properties at low concentrations. These compounds can be considered as new environmentally favorable nonionic surfactants, which could be included in personal care or cosmetic formulations.
Surfactant properties of undec-10’-enyl-d-xylopyranoside and undecenoyl-d-xylopyranose [52].
Entry | Compound | CMC (mmol/L)a | γCMC (mN/m)a | a (Å2)a |
1 | 0.18 | 39 | 42 | |
2 | 0.16 | 36.5 | 45 | |
3 | 0.18 | 25 | 32 |
a CMC = critical micellar concentration, γCMC = surface tension at CMC, a = area of polar head per molecule.
In conclusion, we prepared d-xylose and l-arabinose-based surfactants using telomerization of butadiene, glycosylation or esterification reactions. The syntheses are clean and quite easy to handle, the purifications too. Considered surfactants exhibit good to excellent amphiphilic properties and derivatization of these structures were also explored. In this field, the research is still in course to confer to new structures encapsulating and/or chiral power.