1 Introduction
Novel high-quality components of consumer friendly market products require surfactants with multifunctional characteristics such as biodegradability, mildness, low potential toxicity, water solubility, wide range of hydrophilic–lipophilic balance, and ability to form lamellar phases and vesicles for potential use in biomedical applications [1].
Amino acid glycerolipid conjugates constitute a novel class of lipoamino acids, which can be considered analogues of partial glycerides and phospholipids. They consist of one or two aliphatic chains and one amino acid polar head, linked together through a glycerol moiety (Fig. 1). The resulting structures resemble the acid esters of monoglycerides such as lactic, citric, tartaric and succinic glyceride esters widely used as food emulsifiers [2,3].
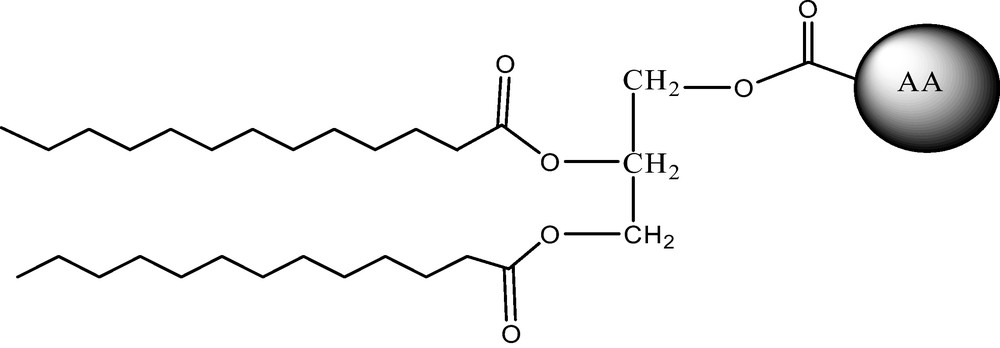
Structure of an amino acid (AA) diacyl glyceride conjugate.
Diacylglyceride conjugates as novel multifunctional soft biocompatible surfactants from diacylglycerides and different amino acids have been prepared and studied in our group during the last decade. We have synthesized anionic, cationic and non-ionic compounds generated from the condensation of mono- and di-acylglycerides with amino acids such as arginine, lysine, glutamic acid, aspartic acid, glutamine, asparagine and tyrosine [4,5]. The physicochemical and biological properties of amino acid glyceride conjugates indicate that these novel compounds combine the advantages of both partial glycerides and lipoamino acids [6]. These surfactants are characterized by phasic phospholipid-like behaviour. They possess antimicrobial activity, typical of lipoamino acids, and form lamellar phases and vesicles, characteristics of partial glycerides and phospholipids [7]. They form thermotropic liquid crystals, and in aqueous media exhibit broad phasic polymorphism leading to micelles, bilayers, vesicles or lyotropic liquid crystals depending on the concentration and temperature [8]. They can also simultaneously stabilise W/O droplets and O/W, forming multiple emulsions [7]. Moreover, the introduction of different ionic groups (i.e. selecting the amino acid) increases the swelling properties by promoting the electric repulsion between charged group bilayers. For all these reasons, they constitute a promising field with a great potential interest in pharmaceutical and food formulations.
Cationic surfactants act as antimicrobials that function by disintegrating bacterial membranes through tandem electrostatic and hydrophobic adsorption with negatively charged membrane components [9]. This activity clearly results from a combination of the interfacial activity of the compounds and the ionic structure of their hydrophilic portions. Compared to conventional cationic surfactants, cationic arginine glycerolipids also are antimicrobials but less toxic and are non-irritating [10]. The physicochemical study shows that these surfactants have self-aggregation behaviour similar to that of lecithins [6]. In this article, a description of diacyl glycerolipid conjugates from acetyl-arginine including preparation, physicochemical properties, membrane interaction and its relation with biological properties are described. In particular, we will give details about the following compounds: 1,2-dilauroyl-rac-glycero-3-O-(Nα-acetyl-l-arginine) (1212RAc), with 12 carbon atoms in the alkyl chains (Fig. 2, n = 10) and 1,2-dimiristoyl-rac-glycero-3-O-(Nα-acetyl-l-arginine) (1414RAc), with 14 carbon atoms in the alkyl chains (Fig. 2, n = 12).
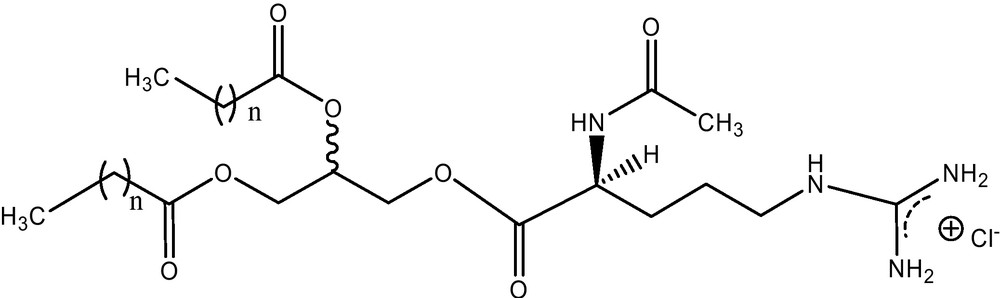
1,2-Dilauroyl-rac-glycero-3-O-(Nα-acetyl-l-arginine), 1212RAc, (n = 10) and 1,2-dimiristoyl-rac-glycero-3-O-(Nα-acetyl-l-arginine), 1414RAc, n = 12.
In some cases, the properties of diacyl glycerolipid conjugates from acetyl-arginine (one cationic charge) will be compared to those of diacyl glycerolipid conjugates from non-acetylated arginine homologues, 1,2-diacyl,3-O-(l-arginyl)-rac-glycerol (XXR) with alkyl chain lengths (X) in the range from eight to 14 carbon atoms (two cationic charges) [6].
2 Synthesis
Diacyl glycerolipid conjugates from acetyl-arginine are made of by a central glycerol backbone that links the hydrophobic part formed by two fatty acid chains of a variable length to the hydrophilic part formed by the amino acid derivative acetyl-arginine linked to the glycerol by its terminal hydroxyl through an ester bond.
This particular structure provide certain advantages over the conventional phospholipids:
- a) the introduction of the arginine as the polar part of the amphiphilic molecule will increase their solubility in an aqueous solution;
- b) the presence of arginine will provide cationic characteristics to the amphiphilic molecule and thus, compounds will be obtained with antimicrobial activity;
- c) the existence of two hydrophobic tails in the hydrophobic moiety confer to the molecule in water the capability to aggregate spontaneously forming cubosomes and/or liposomes useful for biomolecules transport or delivery.
Our group has synthesized these products using chemical and enzymatic methodologies [11,12]. The synthesis of 1212RAc and 1414RAc object of this paper was achieved following a two-step procedure (Scheme 1).
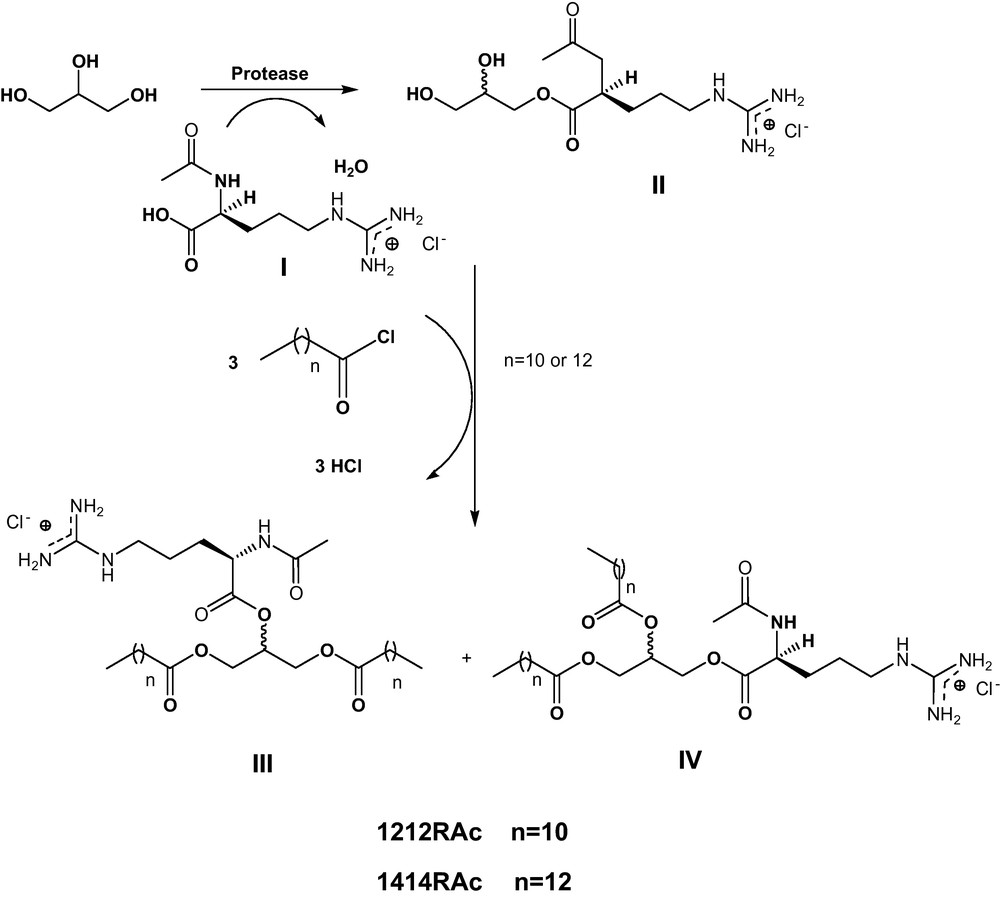
Most important steps for the chemo-enzymatic synthesis of 1212RAc and 1414RAc.
The first requires the prior obtaining of the derivative of the 1-O-l-arginyl glycerol ester type. The relevant bibliography describes the synthesis of these intermediates by using chemical catalysts. Valivety et al. [13] prepared a number of compounds of the 1-O-l-aminoacyl glycerol esters type from Nα-benzyloxycarbonyl-amino acids and glycerol in the presence of BF3-etherate. However, in no case, derivatives of arginine were involved. Although the obtaining of glyceryl esters with N-protected amino acids as starting materials has scarcely been developed, there are numerous publications based on the enzymatic synthesis of ester bonds [14–16]. Mitin et al. [17] describe how the glyceryl esters of N-protected amino acids are obtained for use in the synthesis of peptides, although no examples of arginine derivatives are found in the relevant literature. In our case, the first step involves the enzymatic preparation of the Nα-acetyl-l-arginine glyceryl ester derivative (II). This reaction consisted of the selective protease-catalysed esterification of one of the primary hydroxyl groups of the glycerol with the carboxylate group of the Nα-acetyl-l-arginine (I). The reaction yield was 80%. The second step consists of the preparation of 1,2-diacyl-rac-glycero-3-O-(Nα-acetyl-l-arginine) (IV) by chemical acylation of the two remaining free hydroxyls groups of the acetyl-arginine glyceryl ester derivative (Ac-arginine glyceryl ester) with the corresponding long chain acid chloride. We have found in the literature the use of enzymes of the lipase type for the acylation of the hydroxyl groups of the glycerol [10]. Proteases and lipases were also found to be versatile catalysts for this reaction. But in our case, better yield were obtained using chemical methodologies. The progress control of the reaction showed that the Ac-arginine glyceryl ester derivative was first esterified with 1 mol of acyl chloride to give the corresponding monoacyl derivatives. With a molar ratio acid chloride: Ac-arginine glyceryl ester derivative (1:3), the main reaction products were 1212RAc and 1414RAc with conversions higher than 98%. The overall yield of these reactions after purification was in the range of about 85–70%. Except when it is mentioned all of them were mixtures of diastereoisomers and regioisomers (30/70 mixture of 1,3/1,2 regioisomers, see III and IV in Scheme 1).
3 Phase behaviour
The surfactant phase behaviour is largely connected to the packing possibilities of the molecules [18]. Having these molecules, two hydrophobic chains, their section is larger than single chain surfactants and, from simple packing restrictions, it can be easily seen that the preferred packing will be in the form of flat structures. This has a concomitant effect on a small critical micellar concentration, the formation of vesicles at low concentration in water and the formation of lamellar liquid crystals at high concentrations.
3.1 Diluted aqueous phase behaviour
When the critical micelle concentration (CMC) of the dicationic compounds was systematically studied, a large discrepancy of values between methods was apparent [19]. Conductivity and fluorescence lead to values of 5 mM and 7 mM for 88R (dioctyl glycerolipid conjugates from non acetylated arginine) while a value of 0.07 mM was obtained from surface tension (Table 1).
CMC values obtained by different techniques with one or two charges on the head group.
Compound | ST (mM) | Conductivity (mM) | Fluorescence (mM) | LS (mM) | P (mM) |
88R | 0.07a | 5a | 7a | ||
1010R | 0.006d | 1.1a | 0.005d | ||
0.04d | |||||
0.5d | |||||
1212R | 0.008a | 0.3a | 0.3a | ||
1414R | 0.25a | ||||
1212RAc | 0.12b | ||||
1414 RAc | 0.002–0.008c | 0.09b | 0.002c |
This discrepancy was attributed to the formation of premicellar aggregates. Further studies established that the low value obtained by surface tension was due to the formation of vesicles at very low concentrations, which transformed to ribbons at higher concentrations. In addition, the existence of a large pKa shift was determined, which lowered the apparent pKa of arginine down to values around 5 [20]. Similarly, the acetylated arginine conjugates show even a stronger tendency to forming lamellar structures than the dicationic products.
A CMC value of 0.002 mM has been deduced for 1414RAc from proton activity measurements (Fig. 3), which agrees roughly with the surface tension values [21]. Below this concentration, no change of proton activity was detected compared to that of water, from this point up to 0.01 mM the proton activity increased with a slope close to unity, showing that the surfactant behaves at these extremely low concentrations as a strong acid, i.e. a strong pKa shift is apparent compared with mono acyl arginine compounds.
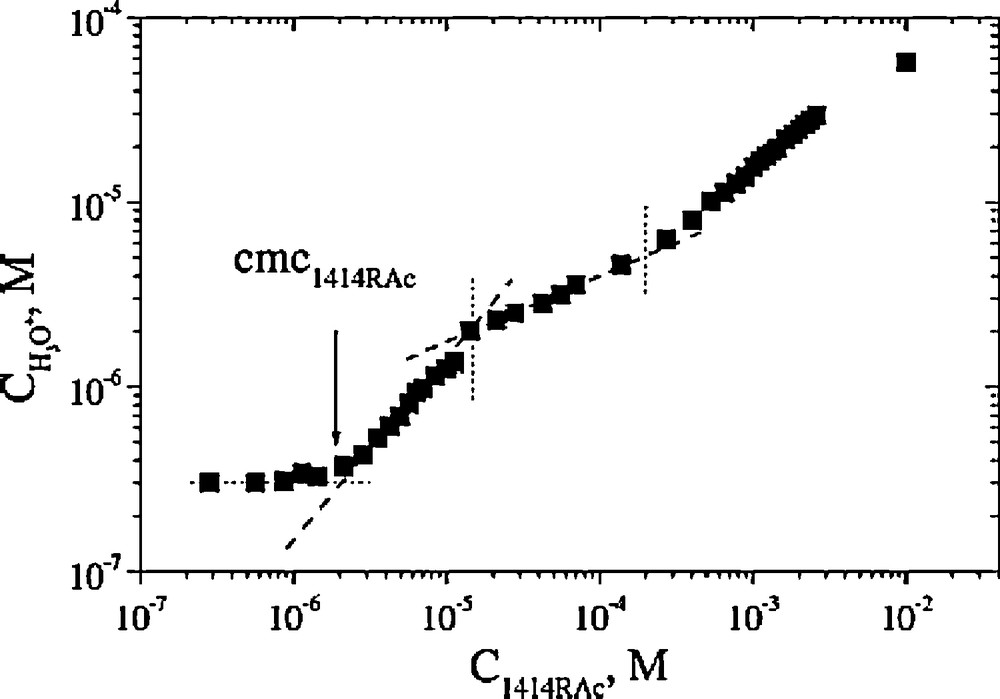
Proton concentration as a function of 1414RAc concentration measured at 25 °C in aqueous solution under nitrogen atmosphere. Dashed lines show different slope regions, and vertical dotted lines mark structural changes.
Reproduced from reference [21] with permission.
The diacyl glycerol surfactants 1212RAc and 1414RAc have been shown to form vesicles at low concentrations from cryo-TEM, SAXS and DLS results [22,23]. Structural changes as a function of concentration have been inferred from proton activity measurements [21]. Although no detailed structural changes with concentration and pH have been published yet [24], there is evidence of a parallel behaviour of the acetylated compounds compared to that of the dicationic counterparts with a reduction in size as a function of increasing concentration and decreasing pH. The same discrepancies between the apparent CMC obtained from conductivity (0.12 mM for 1212RAc and 0.09 mM for 1414RAc) [7] and surface tension show that there exist strong structural changes as a function of concentration.
Both 1212RAc and 1414RAc have been shown to stabilize, to some extend, both oil-in-water and water-in-oil droplets, this is implicit in their ability to produce multiple emulsions without the need of using surfactant mixtures [7]. This is shown in Table 2 where the properties of emulsions prepared with both surfactants are compared to those of the phospholipids DLPC and lecithin, which stabilize O/W and W/O emulsions, respectively.
Composition of the tested emulsions and morphological characteristics taken from reference [7] with permission.
Sample code | Water content (weight fraction) | Oil content (weight fraction) | Surfactant contenta (weight fraction) | Dominant droplet size (μm) | Emulsion type |
E1 | 0.5 | 0.5 decane | 0.00223, 1212RAc | 5/50b aggregates | W/O/W |
E2 | 0.5 | 0.5 decane | 0.00223, 1414RAc | 5/50b | W/O/W |
E3 | 0.09 | 0.91 decane | 0.00022, 1212RAc | 5/50c | O/W/O |
E4 | 0.5 | 0.5 decane | 0.0023, DLPC | 20 | O/W |
E5 | 0.77 | 0.23 squalane | 0.02 Span20 + 0.00045 1212RAc | 10/10 | O/W and W/O |
E6 | 0.77 | 0.23 squalane | 0.01, Span 20 | 10 | O/W |
E7 | 0.5 | 0.5 decane | 0.025, Lecithin | 10 aggregates | W/O |
a The rounded weight fraction sum more than unity.
b The first figure corresponds to water droplets within oil which droplet size corresponds to the second figure.
c The same as footnote b with oil and water roles reversed.
3.2 Concentrated phase behaviour
With increasing the concentrations of 1212RAc in water, two different bilayer structures are formed [23]. The diluted lamellar phase (Lam1) is a turbid dispersion of vesicles formed from 1.5 to 17 wt% 1212RAc. Above 42 wt%, a smectic lamellar phase (Lam2) exists (Fig. 4). Both phases are stable over a wide temperature interval (2–90 °C). Between both lamellar phases (17 < C < 42 wt%), a wide region of coexistence was observed. Below 1.5% a bluish solution, presumably formed by small, unilamellar vesicles, was observed.
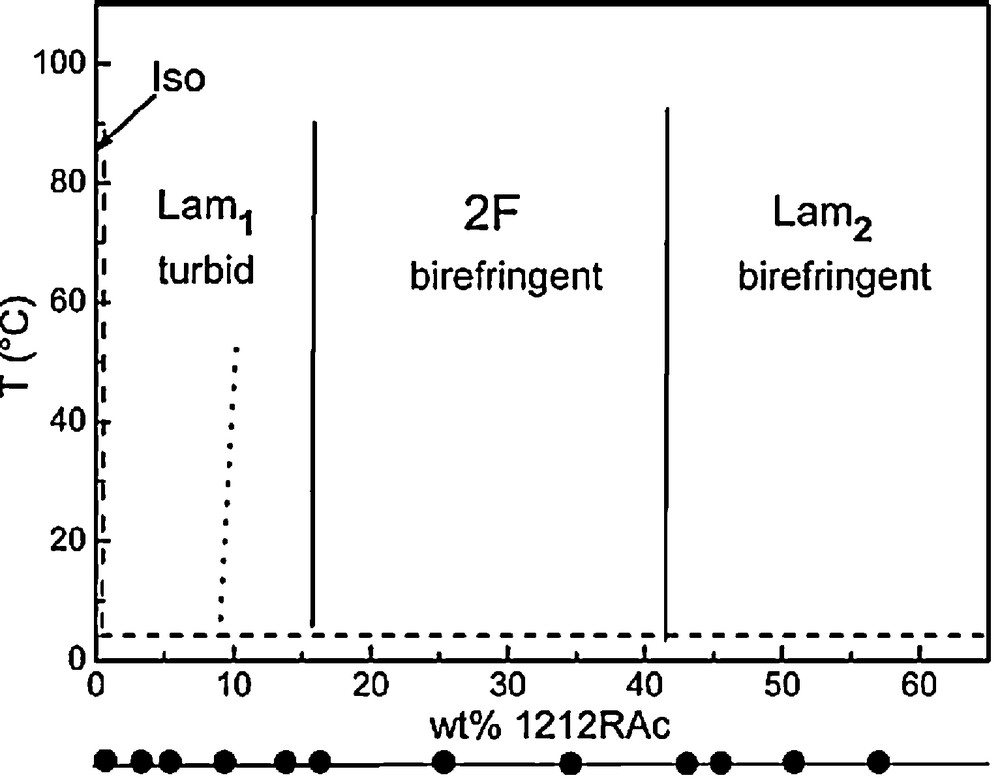
Partial phase diagram of 1212RAc/2H2O system, Iso, transparent solution. Lam1 and Lam2 indicate a dilute and concentrated lamellar phase, respectively. 2F is a two-phase birefringent region.
Reproduced with permission from reference [23].
At high concentrations, both 1212RAc and 1414RAc form lamellar liquid crystals over a wide temperature concentration [7,25], the latter forms a gel phase at room temperature that around 45 °C develops in a lamellar Lα phase. This lamellar phase disperses in water at lower concentrations to produce the vesicles observed at low concentrations. Interestingly, mielinic structures are also observed when contacting the dry product with water in the diluted side, Fig. 5.
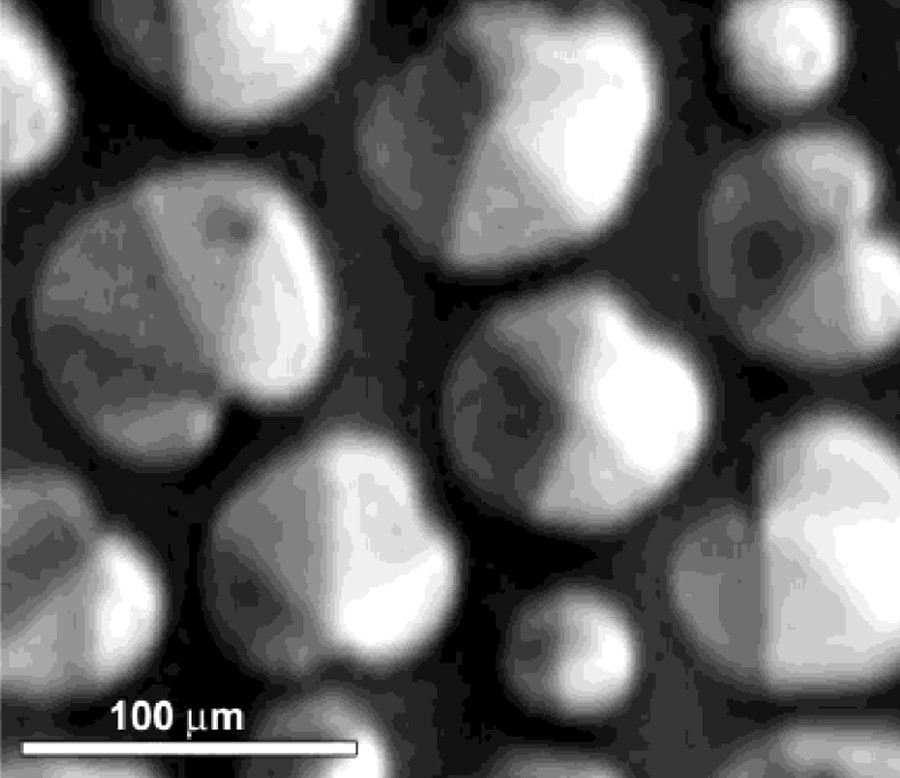
Monolayer of 1414RAc in the LC-LE coexistence region at T = 18 °C and π = 8 mN/m after 3 h of isothermal relaxation at a constant area. Six sectors can be observed in the BAM image.
See reference [26] for further details.
At the limit of high concentration, i.e. the dry product, the thermotropic phase behaviour has been studied [8]. Because of the possible effects of positional isomers on the phase behaviour of these compounds, isomerically pure compounds were separated. The reaction mixture yields a 70/30 mixture of 1,2/1,3 regioisomers. The 1,2 regioisomer could be separated to high purity while the 1,3 regiosiomer could only be enriched to a 50% content, corresponding the remaining to the 1,2 regioisomer. The SAXS curves showed the coexistence of two orders of lamellar spacing in the first heating scan. This coexistence was not observed in thermalised samples, suggesting a strong influence of lyophilisation on the crystalline property of these compounds with the induction of different domains. In the case of the pure 1,2 compound, these different domains may be caused by the presence of both diastereoisomers while in the mixtures, segregation of regioisomers might also be possible. For the pure 1,2-compound, the interlamellar distances were compatible with fully extended alkyl chains at low temperature and this length progressively decreased with increasing temperature showing the melting of the hydrocarbon chains. The regioisomer mixtures pack with increasing tilted chains as a consequence of difficulties in packing.
3.3 Monolayer formation and surfactant monolayer interaction
Because of their structural resemblance with cell membrane phospholipids, their interactions are one of the key elements for the prospective uses of these derivatives. To this end, spread monolayers of the diacyl glycerol arginine compounds 1212RAc and 1414RAc were studied alone [26] and in their mixtures with the 1,2,-dimiristoyl-phosphatidyl choline (DMPC) and 1,2,-Dipalmitoyl-phosphatidyl choline (DPPC) [27]. The use of BAM image analysis of the inner textures has revealed that condensed phases of isomerically pure 1,2 dimyristoyl glycerol compounds exhibit hexatic order, as it can be observed in Fig. 6. It was found that the headgroup charge did not change significantly the monolayer behaviour, and that the differences could be attributed rather to the differences in volume between the dicationic and monocationic compounds (non acetylated and acetylated compounds, respectively). Indications concerning the easier packing of 1,2 derivatives as compared with the 1,3 derivatives was also obtained using glutamine analogues.
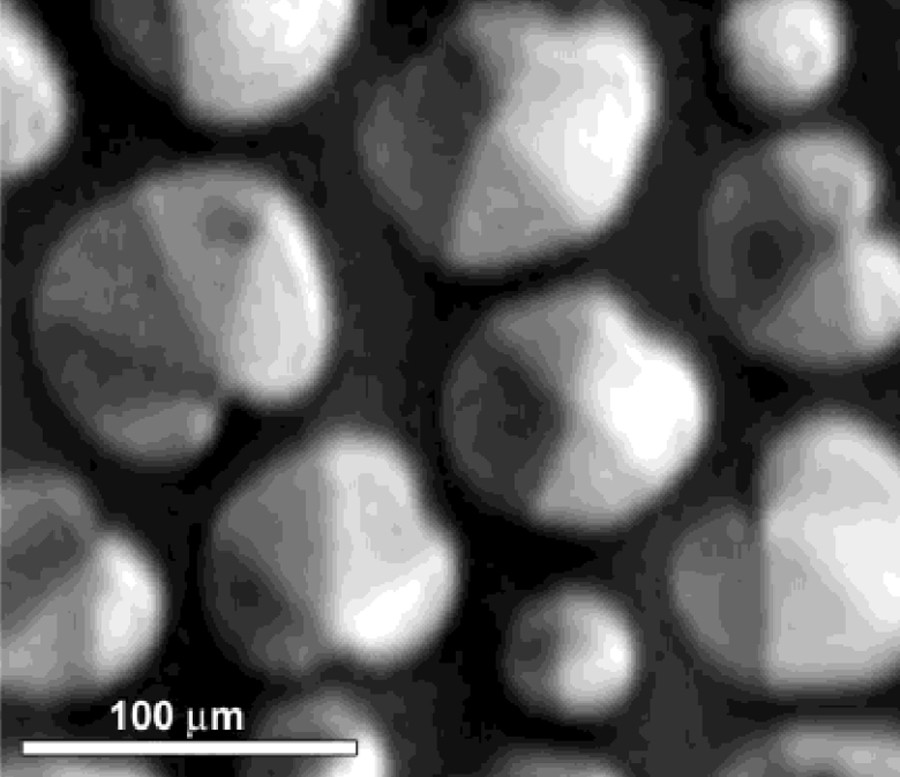
Monolayer of 1414RAc in the liquid condensate-expanded (LC-LE) coexistence region at T = 18 °C and π = 8 mN/m after 3 h of isothermal relaxation at a constant area.
Reproduced from reference [26] with permission.
In Fig. 7, the Langmuir isotherms corresponding to the 1212RAc and 1414RAc derivatives at 25 °C are shown. Amphoteric phosphatidyl choline derivatives as a function of chain length (DPPC, DMPC and DLPC) are also shown for comparison. 1414RAc shows all possible states of the monolayer as a function of increasing pressure: gas, expanded liquid, condensed liquid, solid and collapse. In this sense, the behaviour is similar to that of DPPC. The 1212RAc compound and the phospholipids DMPC and DLPC have similar behaviour. These monolayers collapse at a pressure well below that of 72 mN/m, and only show gas and expanded liquid states.
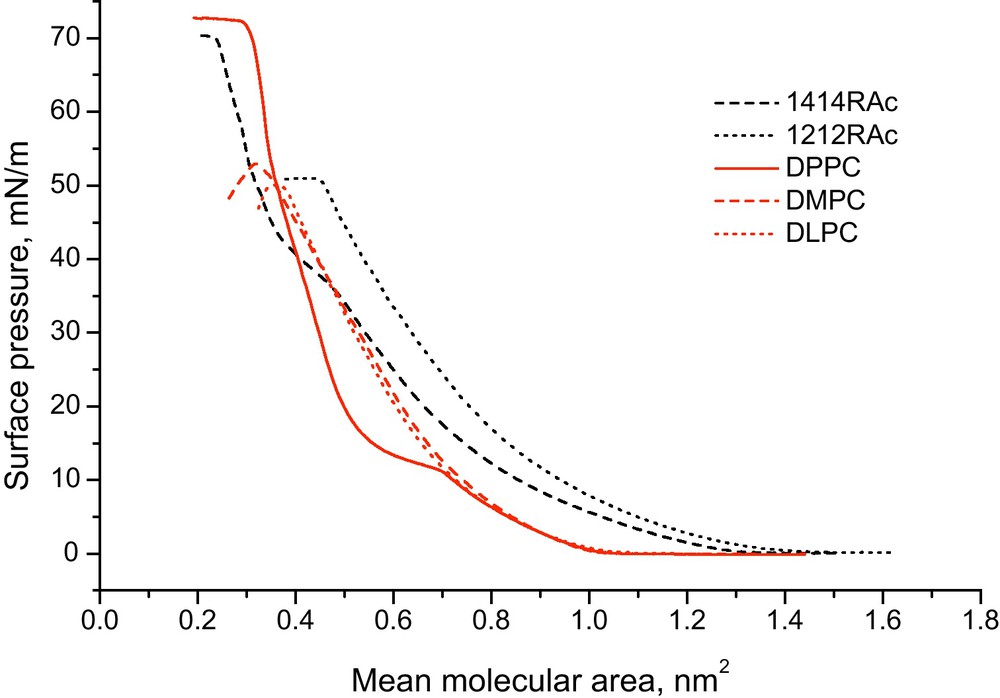
Langmuir isotherms of 1212RAc and 1414RAc compounds at 25 °C. Amphoteric phosphatidyl choline derivatives as a function of chain length: dilauryl, (DLPC), dimiristoyl (DMPC) and dipalmitoyl (DPPC) are also shown for comparison.
Reproduced from reference [27] with permission.
From the pressure-molecular area isotherms of 1414RAc and 1212RAc and mixtures with phospholipids at 25 °C, the mixing properties of these monolayers were determined (Fig. 8) [28]. Interactions between components in mixed monolayers have been studied from the point of view of miscibility. All mixtures present a partial miscibility between the two components except for the mixture 1212RAc/DMPC, which showed positive deviation from the additively rule at phospholipids’ molar fractions of 0.3 and 0.7. For all the mixtures, the monolayer is thermodynamically favoured except for that same mixture, which exhibits positive values of excess Gibbs energy of mixing [27].
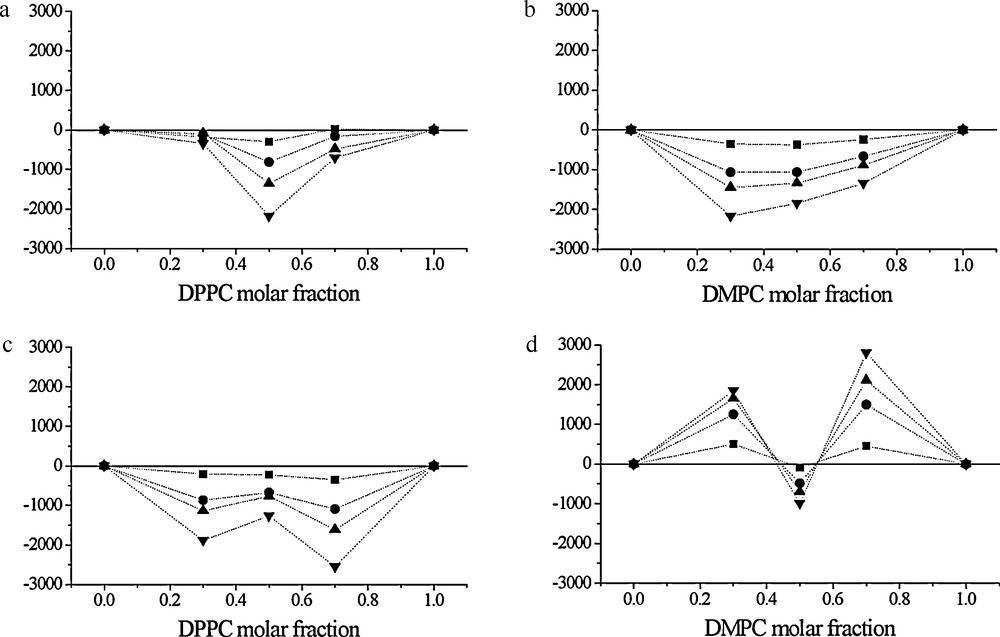
Excess free energy of mixing as a function of the phospholipid molar fraction for different surface pressure values (■:, 5 mN/m; •: 20 mN/m; ▴:30 mN/m; ▾: 45 mN/m) for (a) 1414RAc/DPPC mixtures; (b) 1414RAc/DMPC mixtures; (c) 1212RAc/DPPC mixtures and (d) 1212RAc/DMPC mixtures.
Reproduced from reference [28] with permission.
The properties of these pure and mixed monolayers were also studied using Infrared Reflectance Absorption Spectroscopy (IRRAS) combined with pulsating bubble tensiometry [29]. The results confirm the synergism observed for spread monolayers using the Langmuir balance and further show that both the cationic surfactants and the phospholipid pack more efficiently when mixed both as spread monolayers or as adsorbed monolayers.
The rheological properties of 1414RAc/DPPC spread monolayers have also been investigated [21]. The binary systems are mostly elastic at low frequencies and show more viscous behaviour as the frequency increases. The elastic component is dominated by the value reached by the diacyl glycero arginine surfactant (Fig. 9). This has been interpreted as the signal of a softer type of intermolecular potential for the arginine derivative than for the phospholipid.
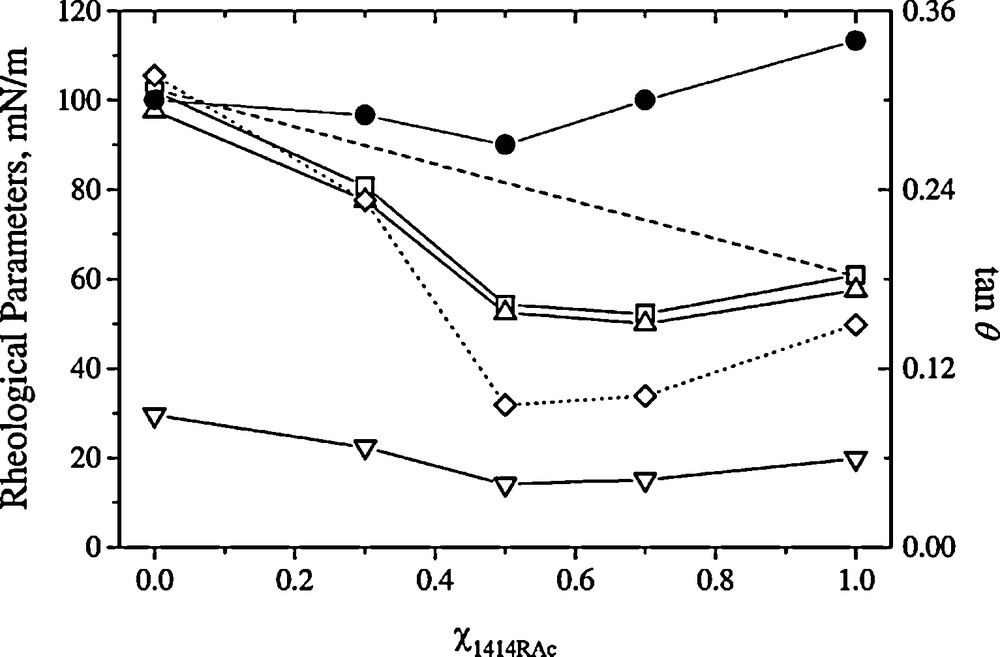
Effect of χ1414RAc on rheological parameters (□: E, surface dilational modulus; ▵: Er, elastic component; ▿: Ei, viscous component and •: tan θ, loss angle tangent) for 1414RAc/DPPC mixed films on the saline subphase (0.1 M sodium chloride) at 25 °C. Surface tension, 47 mN/m; amplitude, 5%; frequency, 50 mHz. Dashed line indicates the ideal behaviour. Comparative with the static elasticity (◊ [Es]) for 1414RAc/DPPC spread films on the saline subphase.
Reproduced from reference [21] with permission.
The rheological parameters of pure and mixed monolayers as a function of interfacial composition for the binary system 1414RAc/DPPC (47 mN/m surface tension, 5% amplitude, 50 mHz frequency) are shown in Fig. 9. The surface dilational modulus (E) of the ideal mixture between 1414RAc and DPPC is shown as a dashed line; the experimental results systematically lie below that curve. The maximum deviation was observed at equimolarity. Both the dilational modulus and elastic component are very close to the values of static elasticity, which were measured previously [21] and are redrawn in the same figure.
This observation could be related to the previous results obtained for the evaluation of the extent of component miscibility using the excess Gibbs energy [21]. Although the 1414RAc monolayers are not completely insoluble (CMC of 2 μM as shown above), at the experimental compression rates they behave as insoluble monolayers, that is, they present a predominantly elastic component. The elastic component of mixtures at χ1414RAc of 0.3 is similar to that of ideal mixing while above this fraction it is similar to that of the pure surfactant. This behavior can be explained in the basis of a similar form of the interaction potential of 1414RAc-DPPC and 1414RAc-1414RAc, which would be softer than the DPPC-DPPC interaction potential. The viscous component remains small for all compositions with a shallow minimum at equimolarity.
Important for the application in living systems are also the kinetics of adsorption from dispersion. The velocities of adsorption of insoluble phospholipids at surfaces are slow and depend of their state of dispersion. It has been shown that small vesicles produce a faster surface tension reduction than bigger and multilayered structures. A strong synergy of adsorption has been found for the addition of small amounts of 1414RAc to DPPC.
This synergy can be clearly observed in Fig. 10. The deep minima for the surface tension of 1414RAc mixed with DPPC observed around 6 μmol/L (open symbols in Fig. 10) shows a strong cooperativity of the mixture with respect to both DPPC alone and to 1414RAc (closed symbols in Fig. 10) at the same concentration. DPPC dispersions do not reduce significantly the surface tension of water when injected in the subphase within the lapse of hours at those concentrations. This has been interpreted as the surfactant helping DPPC to adsorb at the surface, being DPPC the main responsible for the decreased surface tension [21,29]. Note that the surface tension of 1414RAc solutions have a very slow adsorption around 2 μmol/L and above.
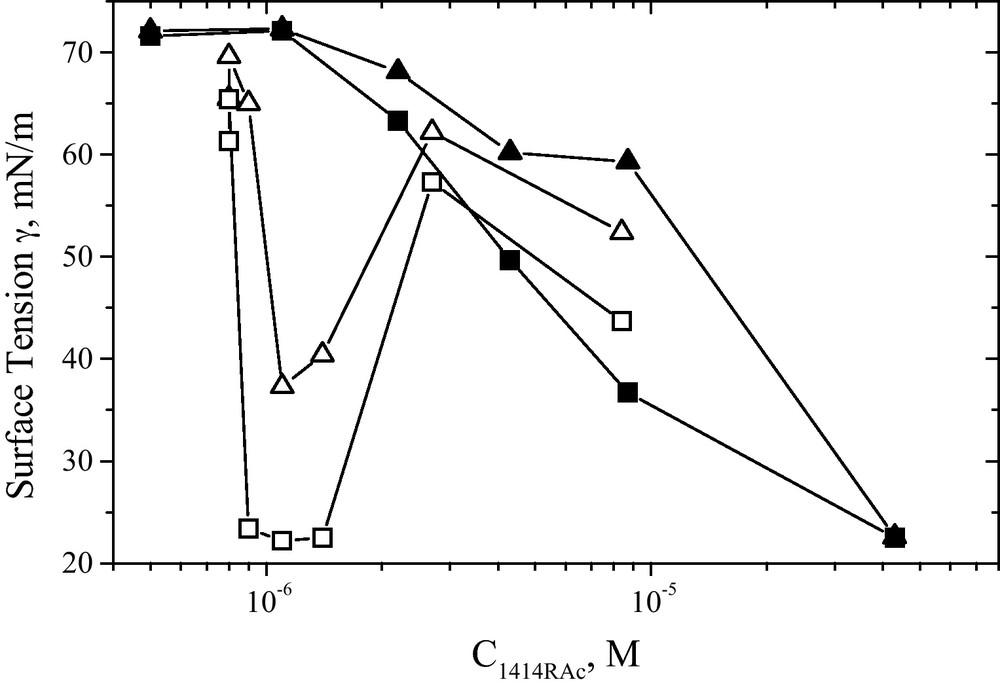
Surface tension of pure 1414RAc (solid symbols) and 1414RAc/DPPC mixtures (open symbols) as a function of surfactant concentration. The total concentration of the 1414RAc/DPPC mixtures is 43 μmol/L. Data taken at 40 min (up-triangles) and 60 min (squares) after the solutions were injected in the saline subphase (0.1 M sodium chloride) at 25 °C.
Reproduced from reference [21] with permission.
3.4 Formation of vesicles
As it has been briefly stated under the aqueous phase behaviour, both the dicationic and acetylated species have a strong tendency to forming vesicles at low concentration in water. These vesicles form either spontaneously or under mild conditions. At this point, the question whether these vesicles constitute a thermodynamic equilibrium state or a kinetically arrested state has been largely debated, see for instance [30]. Their history dependent vesicle size clearly points to the kinetic stability of the system although only limited shear is needed to disperse the system. One interesting possible use of these vesicle forming surfactants is their incorporation in catanionic mixtures, which can be formulated both as anionic or cationic vesicles depending on the proportion of their components [22]. This formulation strategy has been put forward as a method to reduce adverse haemolytic effects while preserving some antimicrobial activity of these surfactants [31]. An interesting aspect of the formation of these catanionic vesicles is the increase Z potential in absolute values for high mixing ratios. This corresponds to the seemingly contradictory finding that adding a small amount of the cationic surfactant to a phospholipid anionic vesicle results in an increase of the anionic net charge of the vesicle or that the addition of an anionic phospholipid to a cationic vesicle of 1414RAc increases the cationic net charge. The origin of this peculiar behaviour may be due to the multiple possibility of the bulky glycero arginine polar head to stabilize net charge by the formation of inter- and intramolecular hydrogen bonding, which allows for a large release of the non surface active counterions from the bilayers.
4 Antimicrobial activity
Classic cationic surfactants, derived from quaternary ammonium, are compounds that have good antimicrobial action [32]. However, their use is seriously questioned from an environmental point of view as they are not readily biodegradable and toxic to aquatic organisms. Furthermore, the growth of resistance to antimicrobial products such as quaternary ammonium compounds (QAC) [33] makes necessary the development of new antimicrobial agents. Cationic surfactants from arginine have good antimicrobial properties as well as high biodegradation levels, so that these compounds can be a good alternative to the QAC in some applications.
Table 3 shows the MIC of the 1212RAc and 1414RAc compounds against some Gram-negative and Gram-positive microorganisms [7]. This table also contains the MIC values obtained for the 88R, 1212R and 1414R surfactants, these compounds are similar to the first ones, the only difference is that they have the α-NH2 group of the arginine as hydrochloride and consequently two positive charges in the molecule [7].
Minimum inhibitory concentration (μg/mL) for diacyl glicerolipid conjugates from acetyl-arginine (1212RAc and 1414RAc) and diacyl glicerolipid conjugates from non-acetylated arginine homologues (88R, 1212R and 1414R).
Microorganisms | 1212RAc | 1414RAc | 88R | 1212R | 1414R |
Gram-positives | |||||
Bacillus cereus var. mycoide ATCC 11778 | 4 | >256 | 64 | >256 | >256 |
Bacillus subtillis ATCC 6633 | 32 | >256 | 64 | >256 | >256 |
Staphylococcus aereus ATCC 6538 | 32 | 32 | 4 | >256 | 256 |
Staphylococcus epidermidis ATCC 12228 | >256 | 8 | 8 | >256 | 16 |
Micrococcus luteus ATCC 9341 | 2 | 4 | 16 | 4 | 1 |
Candida albicans ATCC 10231 | >256 | 8 | 16 | 64 | >256 |
Gram-negatives | |||||
Salmonella typhimurium ATCC 14028 | 32 | >256 | 16 | >256 | >256 |
Pseudomonas aeruginosa ATCC 9027 | >256 | >256 | 64 | >256 | >256 |
Escherichia coli ATCC 8793 | >256 | >256 | 8 | >256 | >256 |
Arthrobacter oxidans ATCC 8010 | 16 | >256 | 32 | >256 | >256 |
Streptoccocus faecalis ATCC 19434 | 1 | 8 | 8 | 2 | 0.5 |
Bortedella bronchiseptica ATCC 4617 | >256 | >256 | 0.25 | 0.25 | 0.25 |
Citrobacter freundii ATCC 22636 | >256 | >256 | 32 | >256 | 256 |
Alcaligenes faecalis ATCC 8750 | 16 | 2 | 8 | >256 | 256 |
Enterobacter aerogenes CECT 689 | 32 | 32 | 32 | >256 | 16 |
Klebsiella pneumoniae v. preumonial CIP 104216 | 8 | 1 | 8 | >256 | 4 |
As expected, the acetylated surfactants show lower antimicrobial activity against the Gram-negative microorganisms, because the different cell wall composition between these two types of bacteria. Given this behaviour and the presence of ester linkages in the molecules, these compounds are easily biodegradable in the environment [34].
It can be observed that the number of cationic charges in the polar head does not affect significantly the antimicrobial activity of this type of surfactants. On the other hand, the alkyl chain length, and consequently the solubility, plays an important role in this property. Given that the natural habitat of microorganisms is the aqueous phase, they must be an effective concentration in this phase to achieve an antimicrobial activity. Because that, the antimicrobial activity of the 88R is much higher that that of the compounds 1212RAc, 1414RAc, 1212R and 1414R. The 88R show low MIC values against all microorganisms tested, being his activity similar to those reported for the HTAB [35], a known biocide product. It should also be noted that the 1212RAc and 1414RAc surfactants are only active against the microorganisms that need concentrations lower than 64 μg/mL to produce the inhibitory effect of the growth. The CMC values of these two surfactants as observed from conductivity are 72.2 and 62.3 μg/mL [7]. This suggests that the species that interact with the cells are the surfactant monomers not the aggregates. However, because the values of CMC strongly depend on the technique used we should be very cautious using these values. The same occurs with the 1212R and 1414R and has also been described for surfactants from arginine [36].
5 Toxicity and environmental issues
Amino acid-based surfactants have attracted much interest as environmentally friendly surfactants because of their biodegradability, low aquatic toxicity and low haemolytic activity. Furthermore, they are prepared from renewable sources of raw materials. Due to the potential application of 1212RAc and homologues in biological field, it is necessary to evaluate their potential adverse toxic, topical irritation and phototoxicity. Vinardell's group has carried out a great investigation in the development of useful toxicological methods to search new amino acid-based surfactants with low irritant and photoirritant action [10,37–40]. A biologically relevant target for skin irritants are fibroblasts and keratinocytes [41,42]. Series of different in vitro models for phototoxicity, included to validate NRU 3T3 phototoxicity assay, are useful screening tools. The phototoxic effects of a novel family of glycerol amino acid-based surfactant compounds were examined via these assays. Human red blood cells and two immortalised cell lines, murine fibroblast cell line 3T3 and one human keratinocyte cell line, HaCaT, were the in vitro models employed to predict potential photoirritation. The phototoxic end-points assessed were hemolysis (human red blood cell test) and resazurin transformation to resorufin and NRU in cell culture methods. It has been studied the toxicity effect of 1212RAc in comparison with its homologue with one alkyl chain, i.e. mono lauroyl glyceride acetyl arginine derivative. Results indicate that the two chains surfactant (1212RAc) are less cytotoxic than the corresponding one chain surfactant as measured with the 3T3 NRU and resazurin assay but increase phototoxicity in the 3T3 cell line and no effects are observed in the HaCaT cell line [37].
The assessment of primary eye and skin irritants by in vitro cytotoxicity and phototoxicity models of compounds XXR with alkyl chain lengths in the range from eight to 14 carbon atoms were studied by Vinardell et al. and compared to 1212RAc and HTAB, a commercial surfactants [37,38].
In Tables 4 and 5, some data about ocular and dermal classification are showed. As expected, HTAB was far more toxic than 14,14R, 88R and 1010R, being 1212RAc and 1212R less toxic.
Haemolysis HC50 (μg/mL) and ocular irritation classification of XXR homologues and 1212RAc compared with a commercial cationic surfactant, the hexadecyl trimethyl ammonium bromide (HTAB) [37].
Compound | HC50 (μg/mL) | Ocular irritation classification |
88R | 24.0 | Middle irritant |
1010R | 20.5 | Middle irritant |
1212R | 60.1 | Middle irritant |
1212RAc | 75.1 | Middle irritant |
1414R | 64.3 | Middle irritant |
HTAB | 43.6 | High irritant |
Half maximal inhibitory concentration (IC50) and dermal irritation classification of XXR homologues and 1212RAc compared with HTAB on the uptake of neutral red by 3T6 mouse fibroblasts and provisional classification in reference to commercial surfactants irritancy valuations.
Compound | IC50 (μg/mL) | Dermal irritation classification |
88R | 60.5 | Middle irritant |
1010R | 74.8 | Middle irritant |
1212R | 345.2 | Slight irritant |
1212RAc | 294.6 | Slight irritant |
1414R | 63.7 | Middle irritant |
HTAB | 0.5 | High irritant |
The biodegradability and aquatic toxicity of these new arginine-based glycerolipidic cationic surfactants has also been studied and compared to conventional cationic surfactants [34]. Acute toxicity tests on freshwater crustaceans (Daphnia magna) as well as on saltwater bacteria (Photobacterium phosphoreum) were carried out to assess the aquatic toxicity of the XXR cationic surfactants. The result of both the D. magna 24 h immobilisation test (IC50) and P. phosphoreum 30 min luminiscent reduction test (EC50) for diacylglycerol arginine-based surfactants XXR and HTAB are summarised in Table 6.
Aquatic toxicity values of XXR series on Daphnia magna and Photobacterium phosphoreum in the aqueous media of the toxicity bioassays.
Compound | D. magna IC50 (μMa) | P. phosphoreum EC50 (μMb) |
88R | – | 1–9 |
1010R | 20–40 | 3–7 |
1212R | 30–50 | – |
1414R | 50–80 | – |
HTAB | 0.36 | 1.73 |
a Concentration values that cause 50% inhibition in the crustacean mobility after 24 h of exposure.
b Concentration values that cause 50% reduction in the light emitted by the bacteria after 30 min of exposure.
The data indicated that the compounds under study are less ecotoxic that the conventional surfactant HTAB and that the P. phosphoreum bacteria population is more sensitive to toxic effects of these arginine-based cationic surfactants than Daphnia, since the concentration of the cationic surfactants, able to reduce to 50% the light emitted by the bacteria, is one order of magnitude less than the concentration required to immobilise 50% of the crustacean population.
The biodegradability of the arginine-based glycerolipidic cationic surfactants XXR prepared in this study was evaluated by applying the Closed Bottle Test [43]. In this test, ultimate biodegradation or mineralisation of the surfactants, that is, the microbial transformation of the parent chemical into inorganic final products of the degradation process, such as carbon dioxide, water and assimilated biomass, was determined.
The biodegradation extent obtained for the diglycerolipidic cationic surfactants (1212R, 1414R), after a 28-day period surpassed 60% (82, 61, 71 and 98%, respectively) while 1010R underwent only a modest amount of biodegradation (20%). (Table 7) the excellent biodegradation of all these surfactants, with the exception of 1010R, allows them to be regarded as readily biodegradable compounds, which are consistent with the presence in the molecules of ester bonds susceptible to chemical or enzymatic hydrolysis that leads to the formation of easily biodegradable metabolites such as arginine, glycerol, acyl glycerol, etc. The poor degradation of 1010R could be attributed to its inhibitory effects on the bacterial population since, as reported elsewhere [44], this homologue possesses the highest antimicrobial activity of the XXR surfactant series.
Ultimate biodegradation of XXR surfactant series.
Compound | % Biodegradation Closed Bottle Test O2 consumption |
1010R | 20 |
1212R | 82 |
1414R | 61 |
HTAB | 0.36 |
Acknowledgment
This work was supported by the Spanish CYCIT, reference CTQ2009-14151-C02-01.