1 Introduction
Biomass is a readily available raw material and has the advantage of being renewable. With the growing awareness of environmental issues, its non-food value is of interest. The use of pentoses from agricultural resources for the synthesis of green surfactants fits this perspective.
Since recently, the non-food valorization of pentoses extracted from the hemicellulose fraction of wheat has been the subject of several studies [1]. Their transformation into surface-active components is one of the most promising one.
Surfactants are molecules with specific properties (emulsifying, detergent, wetting, foaming) due to their amphiphilic structure and have many industrial applications, particularly in detergent formulations and cosmetics [2].
Our previous studies have shown the possibility of synthesizing pentosides having an octadienyl chain, with a double bond at the terminal position, from the Pd-catalyzed telomerisation of butadiene with pentoses [3]. 1-O-Alkenoyl- and alkenyl d-xylosides were shown to exhibit interesting surface-active properties [4].
Bolaamphiphilic molecules are another class of surfactants. They are composed of two identical hydrophilic heads connected by an hydrophobic segment. In aqueous media, these compounds have the ability to form aggregates which can constitute micro reservoirs for hydrophilic and/or lipophilic compounds [5]. Bolaamphiphiles can be used as therapeutic or cosmetic agents for encapsulation and/or vectorisation [6], for example, for transport and delivery of active ingredients in the body. Comparatively to most of bolaamphiphiles, sugar-based ones have a higher hydrosolubility and a reduced cytotoxicity [7]. In the literature, examples of non-ionic, symmetrical or asymmetrical sugar-based bolaforms exist but they are mainly composed of hexoses or disaccharides [8–16], but very few of pentoses [17].
In aqueous media, bolaform molecules organize themselves in aggregates that may differ depending on the polar head and the length and flexibility of the spacer, and also the presence of foreign substances like salt, sugar, etc. in solution [4,5,18]. The bolaform within the aggregates can adopt a U bent or a stretched geometry [19]. Measurement of compression isotherms at an air–water interface is one of the methods that can provide information about the conformation adopted by a bolaform at an interface [19,20].
Recently, we have prepared d-xylose-based bolaamphiphiles (Scheme 1) and the properties (aggregation behavior and membrane interaction) of 1′,18′-bis-octadec-9′-enyl-α-d-xylopyranoside (1, C18unsat) (Fig. 1) have been studied [17].
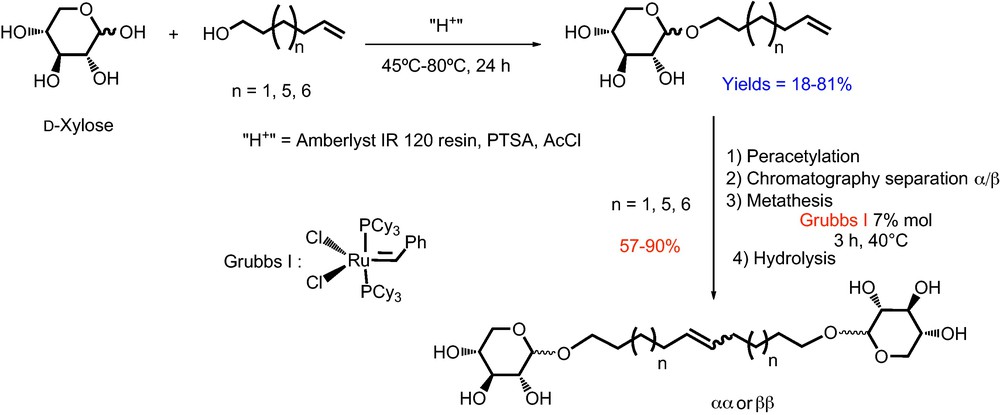
Synthesis of monocatenar and bolaform surfactants from d-xylose.
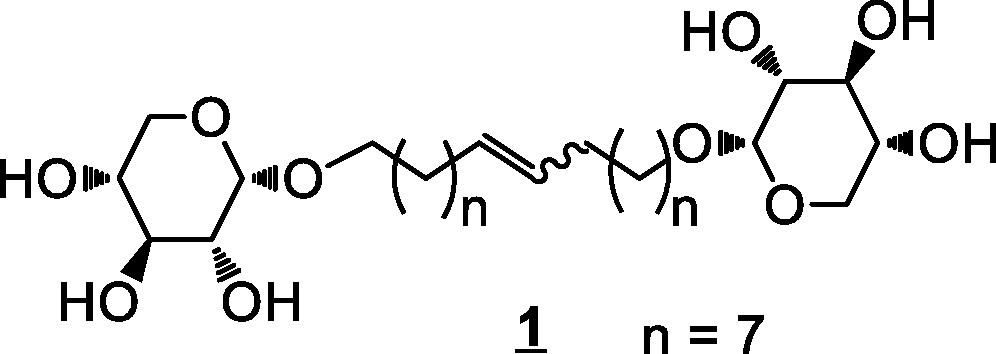
Bolaamphiphile 1 (C18unsat).
Its critical micellar concentration value (∼0.05 mM) is low, compared to those of conventional surfactants and thermodynamic analysis (surface tension measurements and isothermal titration calorimetry (ITC)) has revealed that the aggregation phenomenon is mainly a driven entropy process. It has been also shown that 1 (C18unsat) is able to interact with dipalmitoylphosphatidylcholine (DPPC) monolayers, in particular if their surface pressure is ≥ 15 mN/m, highlighting the potential of this bolaamphiphile as a surfactant and membrane interacting agent.
This article reports the synthesis and the physico-chemical studies (monolayer properties, adsorption behaviour and membrane destabilization) of the xylose-based bolaamphiphile 4 (C18sat) and the saturated analogue of 1 (C18unsat), in order to focus on the importance of the nature of the spacer chain. Results provide some insights about their potential valorizations.
2 Results and discussion
2.1 Synthesis of 1′,18′-bis-octadecyl-α-d-xylopyranoside 4 (C18 sat)
The hydrogenation reaction of 1′,18′-bis-octadec-9′-enyl-(2,3,4-tri-O-acetyl)-α-d-xylopyranoside (compound 2) issued from the metathesis of peracetylated xyloside α was conducted in the presence of Pd/C in ethyl acetate [21]. After 24 h under a hydrogen atmosphere, 1′,18′-bis-octadecyl-(2,3,4-tri-O-acetyl)-α-d-xylopyranoside (compound 3) was obtained as a colorless oil with a yield of 84% (Scheme 2). The characterization of 3 by 13C NMR shows the disappearance of signals at 130.3 and 130.7 ppm, relative to the carbon atoms of the double bond.
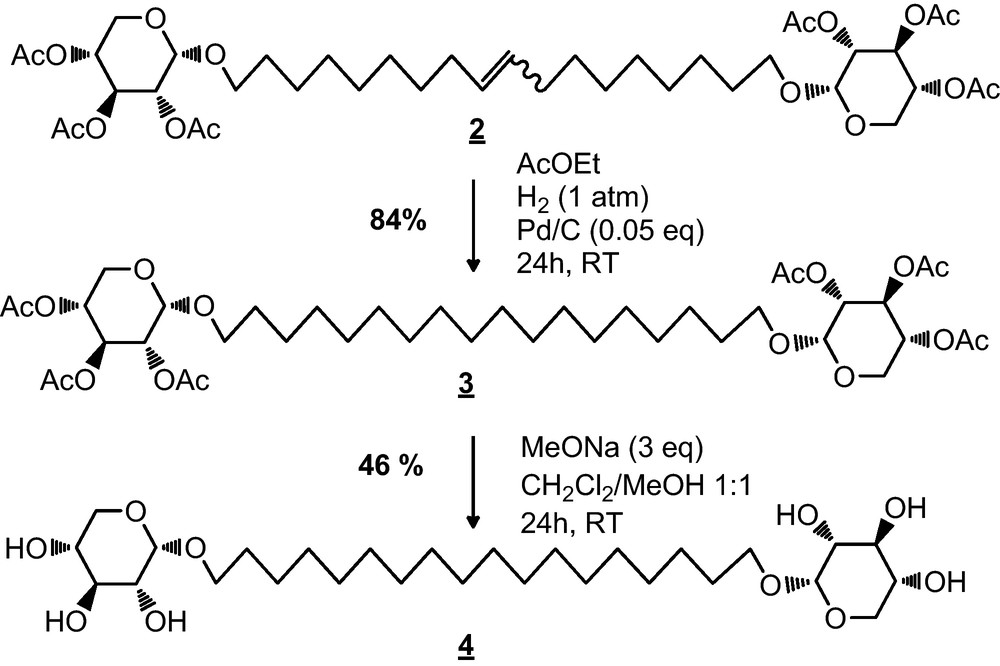
Synthesis of bolaamphiphile 4 (C18 sat).
Next, the deacetylation of compound 3 was carried out in the presence of sodium methoxide in a dichloromethane/methanol mixture (1/1 v/v) [22] with a yield of 46% (Scheme 2). 1H and 13C NMR analysis of 4 (C18 sat) showed the complete disappearance of signals corresponding to acetyl groups. The moderate yield of the deacetylation step could be explained by C18sat purification method, which consists of a recrystallization from a minimum of hot methanol.
2.2 Physico-chemical studies
2.2.1 Behaviour at an air–water interface
The organisation of C18sat and C18unsat at an air–water interface was studied using the Langmuir trough technique. The compression isotherms of their monolayers at an air–water interface at 25 °C are shown in Fig. 2.
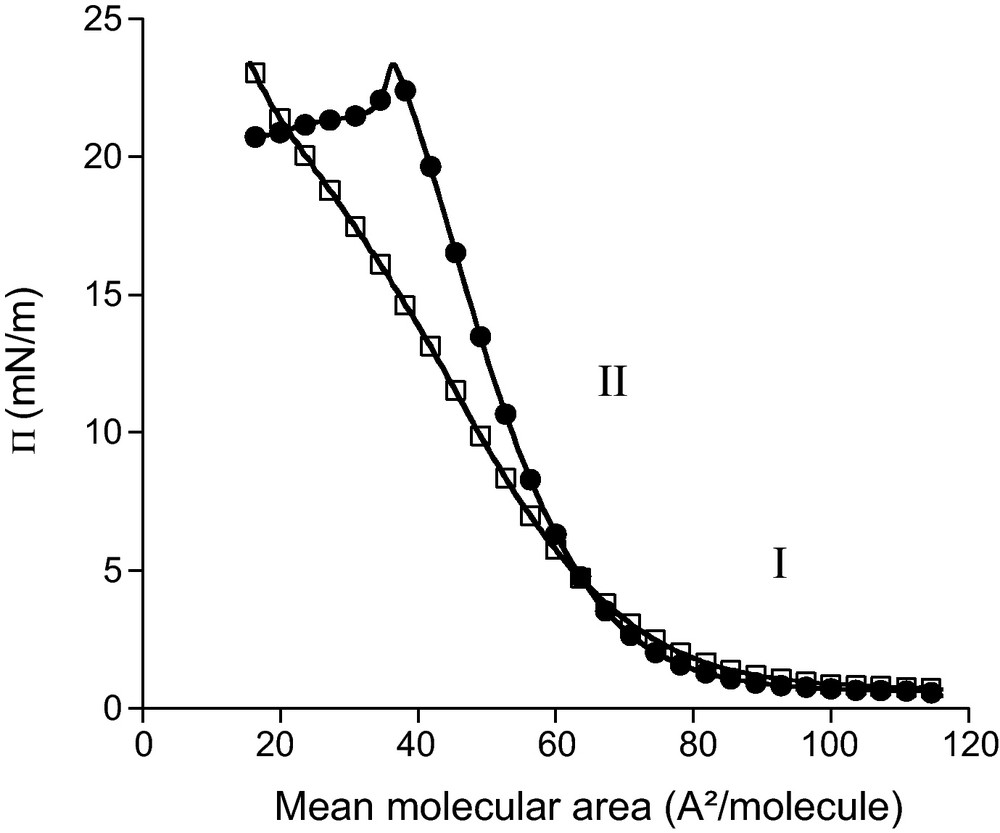
Surface pressure (Π)–molecular area of pure C18sat (●) and C18unsat (□) at an air–water interface at 25 °C.
Both curves present two parts (I and II) which are characteristic of the physical state of the monolayers. Part I of the isotherm corresponds to the gaseous state. The molecules at the interface are so far apart that there is little, if any, interaction between them [23]. Part II is characteristic of a liquid-expanded film in which a certain degree of cooperative interaction occurs between the molecules at the interface [24]. The intersection of the tangent to this part of the curve with the mean molecular area axis defines the initial interfacial molecular area (A0), which corresponds to the cross-sectional area of the molecules when they begin to adopt a particular orientation without being closely packed. For both molecules, A0 is 75 Å2. This value is similar to the one determined in static conditions for C18unsat (81 Å2) from the maximum excess concentration calculated by the Gibbs equation [17]. In an unpacked state, C18sat and C18unsat occupy thus the same area at the interface. This indicates that the presence of the double bond in the spacer comprising 18 carbon atoms does not impair its flexibility. As observed for other bolaforms [19], it can be suggested that C18sat and C18unsat adopt a loop structure at the interface with both xylose heads close to each other and immersed in water.
In the liquid-expanded state (part II), both curves are superimposed until 7 mN/m. At higher surface pressure (Π), C18unsat curve exhibits a smaller slope than the C18sat one suggesting that C18sat monolayer has a lower compressibility than C18unsat. However, the presence of a kink around 15 mN/m in the C18unsat curve, corresponding to a mean area of 35 Å2/molecule, implies some changes in the molecular organization or behaviour of the film. At the same mean molecular area, an abrupt of slope is observed for the C18sat curve. As this molecular area is similar to the one determined for simple alkyl glucosides [25], it could be suggested that at this stage, one of the polar heads of the bolaform (C18sat and C18unsat) is displaced from the interface into the subphase.
Consequently, the interfacial area is reduced by two, compared to the A0 where both polar heads are at the interface. The surface pressure at which the organization change occurs for the C18sat monolayer is much higher (around 23 mN/m) than for C18unsat. The monolayer formed by C18sat molecules is thus more stable than that of the unsaturated analogue.
For both components, no sharp increase in surface pressure is observed, even at very low areas per molecule, indicating that C18sat and C18unsat monolayers cannot adopt a liquid-condensed state [26].
In the case of C18unsat, no collapse point is observed. As 20 Å2 is considered as the minimal area occupied by a simple surfactant with one aliphatic chain [23], it can be suggested that the C18unsat monolayer is overcompressed below this area value and that some molecules are pushed from the interface into the subphase and/or form multilayers at the interface.
Another important property of surfactants is their ability to adsorb at an interface as well as the kinetic of this process. In this work, the adsorption ability of C18sat and C18unsat from a pure water subphase to the air–water interface is studied by monitoring the surface pressure increase (ΔΠ) as a function of time (Fig. 3).
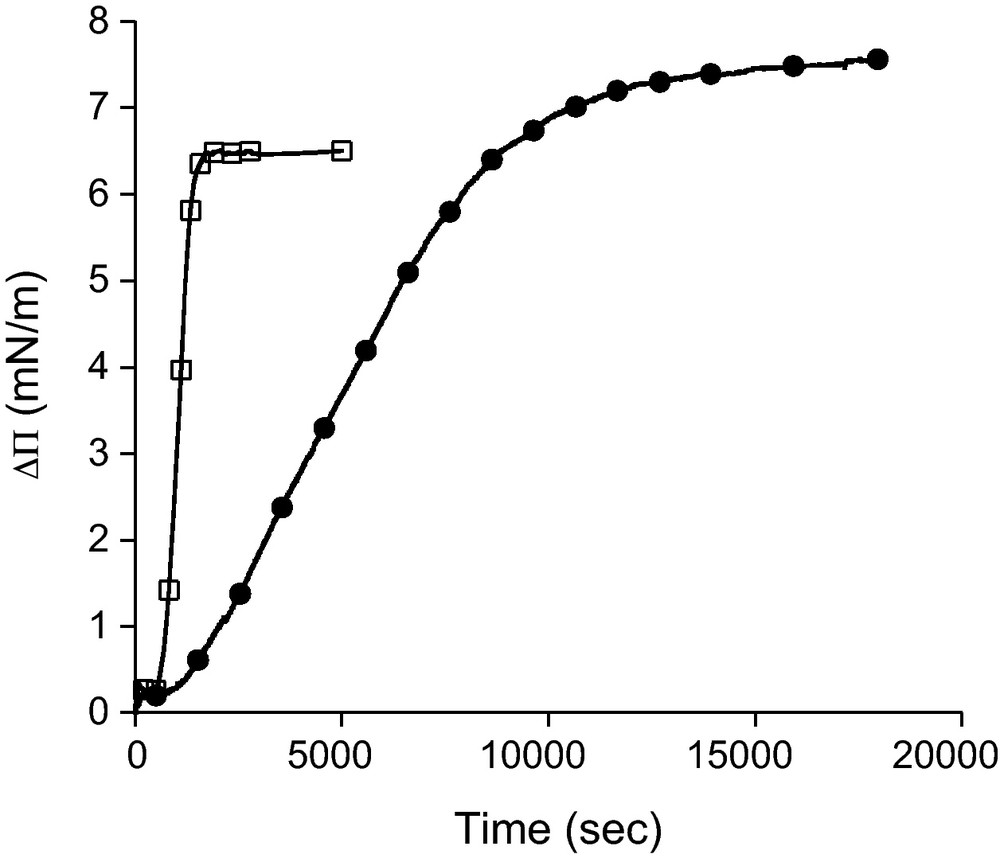
Surface pressure increase (ΔΠ) as a function of the time elapsed after injection of C18sat (●) and C18unsat (□) bolaform into the subphase (ultrapure water at 25 °C). Final concentration in the subphase is 2.34 ± 0.09 μM.
For both components, an increase of surface pressure is observed, indicating that these molecules are sufficiently amphiphilic to diffuse and adsorb at the interface. The presence of a double bond has a slight effect on the equilibrium surface pressure (7.5 mN/m for C18sat and 6.5 mN/m for C18unsat) while it has a large impact on the kinetics of the adsorption. Indeed, the ΔΠ plateau is reached after a much longer time for C18sat (∼12 000 s) than for C18unsat (∼1500 s). This indicates that the absence of double bond increases greatly the arrangement step of the molecule at the interface. On the contrary, the diffusion step seems not to be influenced by the unsaturation as the time before observing an increase in ΔΠ is the same for both bolaforms (delay of ∼600 s after the injection).
2.2.2 Membrane destabilizing property
C18unsat has been shown to be able to interact with a DPPC monolayer which was taken as a simplified biological membrane model [17]. In the present work, its interaction with biological membranes is further explored by using a more realistic membrane model consisting of vesicles composed by a mixture of lipid representative of eucaryote membranes. The influence of the double bond within the hydrophobic spacer is investigated by comparing the results obtained for C18sat and C18unsat. Their membrane destabilizing capacity is evaluated by studying their ability to permeabilize lipid bilayers and their capacity to cause the fusion of lipid vesicles.
The measurement of membrane permeabilization is performed by monitoring the release of a fluorescent probe (HPTS) encapsulated in the aqueous compartment of the vesicles. Release of HPTS is measured after 15 min of incubation of liposomes with bolaform at two different bolaform/lipid ratios (R = 0.1 and 0.4) (Fig. 4). At both ratios, a very slight membrane permeabilization was induced by C18unsat. This suggests that C18unsat inserts only weakly within the bilayer or that its insertion has only a slight destabilizing effect, contrary to C18sat that exhibits a higher but limited (< 25%) effect. From a molecular point of view, the partial membrane permeabilization induced by membrane-interacting surfactants could result from two mechanisms. Either: (i) some vesicles in the population release all their aqueous contents, while the rest maintain the permeability barrier intact; or (ii) virtually all vesicles gradually release the probe and this process is followed by an annealing process that prevents further leakage [27]. The first mechanism is in accordance with permanent pores formation, while the second can be explained by a transient membrane destabilization arising from the penetration and rearrangement of the surfactant within the bilayer. The higher degree of freedom of C18sat hydrophobic spacer is in better agreement with the second hypothesis.
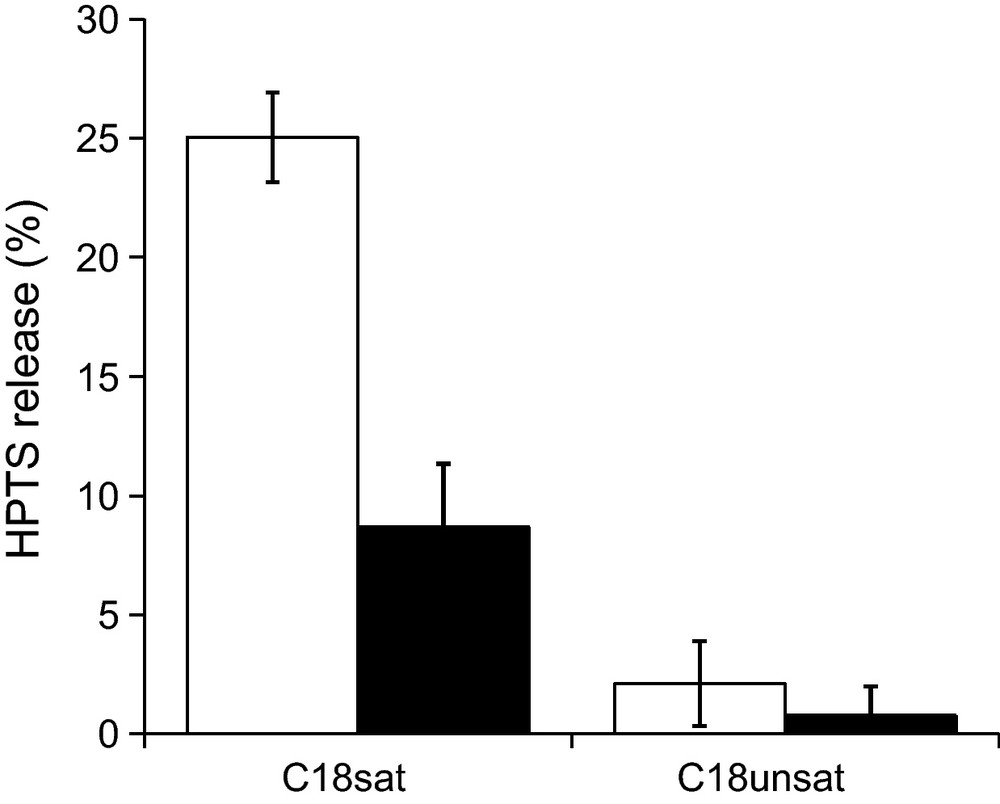
Release of 8-hydroxypyrene-1,3,6 trisulfonic acid (HTPS) from PC/PE/SM/CHOL (1/1/1/2.5 w/w/w/w) liposomes, upon addition of bolaform at two bolaform/lipid molar ratios (R = 0.4 and 0.1 for the white and black bars, respectively). The ordinate shows the amount of HTPS released after 15 min in the presence of the surfactant as a percentage of the total amount released by Triton X-100.
The ability of bolaforms to induce lipid fusion was investigated by recording the increase of fluorescence of octadecylrhodamine B chloride (R18) inserted into the hydrophobic core of the bilayer upon its dequenching by dilution due to the mixing of the lipid phases [28]. The effect of the double bond in the spacer is evaluated by comparing the fluorescence intensity obtained after 15 min of C18sat or C18unsat incubation with liposomes (Fig. 5).
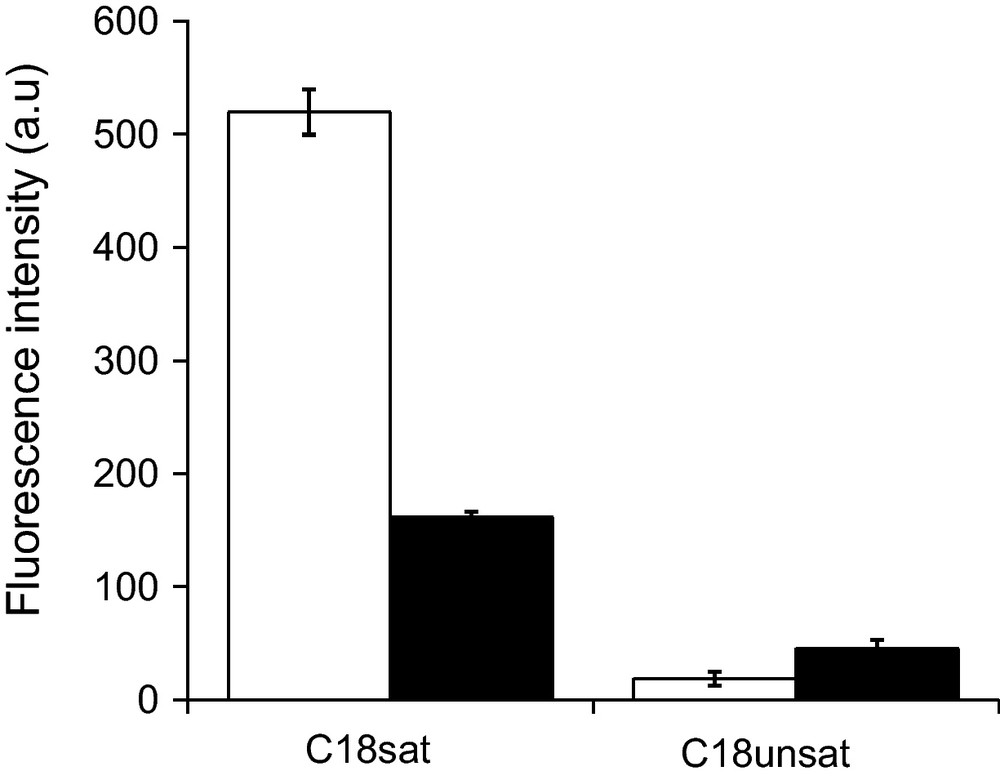
Fluorescence intensity as a measure of dequenching of octadecylrhodamine B chloride (R18) after addition of bolaform. Liposomes of PC/PE/SM/CHOL (1/1/1/2.5 w/w/w/w) were incubated for 15 min at room temperature in the presence of bolaform at two bolaform/lipid molar ratios (R = 0.4 and 0.1 for the white and black bars, respectively).
The much higher membrane destabilizing effect of C18sat compared to C18unsat is confirmed by its much greater lipid fusion capacity at both bolaform/lipid ratios investigated.
Destructuration of the vesicles by lipid phase fusion suggests that C18sat could promote the formation of highly curved “stalk” intermediates required for the fusion of membranes [29].
3 Conclusion
The presence of one double bond in the hydrophobic spacer of the xylose-based bolaamphiphile has no influence on its interfacial organization at low compression but impairs the stability of its monolayer at high compression. Saturated and unsaturated molecules are suggested to adopt a loop structure at the interface at low compression.
The higher degree of freedom of the saturated hydrophobic spacer does not affect the initial diffusion step of the bolaform from the subphase to the interface but greatly slows the arrangement step at the interface. However, once at the interface, their surface-active properties are similar.
The higher flexibility of the saturated analogue spacer also greatly increases its lipid vesicle destabilizing property. Its rearrangement within the lipid bilayer is in favour of the formation of inverted phases facilitating membrane fusion.
From an application point of view, the saturated bolaform could be more suitable for the formulation of drug delivery systems view the higher stability of the film it can form. However, the molecule could give rise to side effects due to its relatively high destabilizing effect observed on membrane models.
4 Experimental
4.1 Syntheses
All reagents were commercially available and used as received. CH2Cl2 was dried over CaH2 and distilled under argon before use. 1H and 13C NMR spectra were recorded on an AC 250 Bruker in CDCl3, DMSO-d6 with TMS as reference for 1H spectra and CDCl3 (δ 77.0), DMSO-d6 (δ 39.5) for 13C spectra. The infrared spectra were recorded with Spectrafile IRTM Plus MIDAC. C, H and N analyses were performed on a Perkin Elmer 2400 CHN equipment. Chromatography was carried out on SDS Silica 60 (40–63 μm), Art 2050044 (flash-chromatography) or Silica 60 F254 (TLC plates).
4.1.1 1′,18′-bis-octadecyl (2,3,4-tri-O-acetyl)-α-d-xylopyranoside
In a Schlenk tube under an inert atmosphere, compound 2 (1.178 g, 1.5 mmol, 1 eq) and Pd/C (319.2 mg, 0.075 mmol, 0.05 eq) are suspended in ethyl acetate (5 ml). The argon atmosphere is then replaced by a dihydrogen one. After stirring for 24 h at room temperature, the mixture is filtered through Celite and washed with ethyl acetate. After evaporation of the solvent under reduced pressure, the compound 3 is obtained as a colorless oil with a yield of 84%.
IR (KBr), cm−1: 2929, 2853, 1751, 1468, 1144, 1055.
1H NMR (CDCl3, 250 MHz), δ (ppm): 5.42–5.50 (2H, H3), 4.92–4.97 (m, 4H, H1α, H4), 4.73–4.79 (m, 2H, H2), 3.41–3.78 (m, 6H, H1’b, H18’b, H5), 3.31–3.38 (m, 2H, H1’a, H18’a), 2.01–2.04 (6 CH3), 1.54 (m, 4H, H2’, H17’), 1.24 (m, 28H, H3’, H4’, H5’, H6’, H7’, H8’, H9’, H10’, H11’, H12’, H13’, H14’, H15’, H16’).
13C NMR (CDCl3, 62.9 MHz), δ (ppm): 170.6 (6 C = O), 96.0 (C1α), 71.6 (C2), 70.1 (C3), 69.9 (C4), 68.9 (C1’, C18’), 58.6 (C5), 30.1 (C2’, C4’, C5’, C6’, C7’, C8’, C9’, C10’, C11’, C12’, C13’, C14’, C15’, C17’), 26.4 (C3’, C16’), 21.1 (6 CH3).
Elemental analysis: Calcd: C 59.83%; H 8.28%. Found: C 59.79%; H 8.59%.
4.1.2 1′,18′-bis-octadecyl-α-d-xylopyranoside
In a Schlenk tube under an inert atmosphere, compound 3 (1.014 g, 1.3 mmol, 1 eq) and sodium methoxide (211 mg, 3.9 mmol, 3 eq) have been dissolved in a mixture of CH2Cl2/MeOH: 1/1 (10 ml). After stirring overnight at room temperature, the mixture was neutralized with resin Amberlyst IR 120H, filtered and the solvent was evaporated to dryness. The residue was then recrystallized from hot methanol. After removal of supernatant, followed by filtration and evaporation to dryness, the compound 4 has been obtained as a white powder with a yield of 46%.
IR (KBr), cm−1: 3404, 2918, 2852, 1595, 1471, 1145, 1043.
1H NMR (250 MHz, DMSO-d6), δ (ppm): 4.55–4.56 (d, 2H, H1α, J 3.5 Hz), 3.89 (sl, 6 OH), 3.51–3.57 (m, 5H, H1’b, H5e, H18’), 3.23–3.48 (m, 7H, H3, H4, H1’a, H15’), 3.16–3.18 (m, 4H, H5a, H2), 1.48–1.51 (m, 4H, H2’, H17’), 1.23 (m, 28H, H3’, H4’, H5’, H6’, H7’, H8’, H9’, H10’, H11’, H12’, H13’, H14’, H15’, H16’).
13C NMR (62.9 MHz, DMSO-d6), δ (ppm): 99.3 (C1α), 73.7 (C3), 72.3 (C2), 70.3 (C4), 67.5 (C18’, C1’), 62.3 (C5), 29.5 (C2’, C4’, C5’, C6’, C7’, C8’, C9’, C10’, C11’, C12’, C13’, C14’, C15’, C17’), 26.1 (C3’, C16’),
Elemental analysis: Calcd: C 60.58%; H 9.83%. Found: C 60.43%; H 9.72%.
4.2 Physico-chemical studies
4.2.1 Monolayer studies
For isotherm experiments, 4 (C18sat) and 1 (C18unsat) monolayers were prepared at 25 ± 0.2 °C with an automated LB system (KSV minitrough, KSV instruments Ltd., Helsinki, Finland).
C18sat or C18unsat were dissolved in dichloromethane/methanol (9:1 v/v) at a concentration of 1.35 mM. Precise volumes of these solutions (between 20 and 30 μl) were spread on milliQ water using an Hamilton syringe. After a waiting time of 15 min allowing for solvent evaporation and spreading of the molecules, the monolayers were compressed at a rate of 3 mm/min. The surface pressure of the monolayer was measured with an accuracy of 0.1 mN/m using a platinum plate. The difference between the molecular areas of two independent sets of measurements was less than 10%.
For adsorption experiments, C18sat or C18unsat, solubilized in DMSO, was injected (20 μl) into the subphase (milliQ water) to a final concentration of 2.34 ± 0.09 μM. The injection was done using a Hamilton syringe and two homemade devices allowing the injection without disturbing the air–water interface. These devices were placed at two fixed positions on the trough to ensure a reproducible injection process. Furthermore, during the entire duration of the experiment, the subphase was stirred using two cylindrical micromagnetic rods (8 × 1.5 mm) and two electronic stirrer heads located beneath the trough (model 300, Rank Brothers, Bottisham, U.K.). An autoreversing mode with slow acceleration and a stirring speed of 100 rpm was selected. After the injection of bolaform, the increase in surface pressure was recorded. The difference between ΔΠ of two independent sets of measurements was less than 0.5 mN/m.
4.2.2 Preparation of lipid vesicles (LUV liposomes)
Large unilamellar vesicles (LUV) are prepared as described by Tyteca et al. [30]. LUV are made from a mixture of phosphatidylcholine (PC), phosphatidylethanolamine (PE), sphingomyelin (SM) and cholesterol (chol) (1/1/1/2.5 w/w/w/w) (Sigma, St. Louis, MO, USA). Lipids are dissolved in chloroform:methanol (2:1, v:v) in a round bottomed flask. The solvent is evaporated under vacuum (Rotavapor R Büchi RE-111, Büchi, Flawil, Switzerland) to obtain a thin film of lipids, which is dried overnight in a vacuum dessicator. The dry lipid films are hydrated for 1 h under nitrogen at 37 °C in a 10 mM Tris (Sigma, St. Louis, MO, USA), 150 mM NaCl (Merck, Darmstadt, Germany), 0.01% EDTA (Merck, Darmstadt, Germany) and 1 mM NaN3 (Sigma, St. Louis, MO, USA) buffer at pH 7.4. The suspension is submitted to five cycles of freezing/thawing to obtain multilamellar vesicles. These are further extruded to produce LUV [31] via 10 passages on a Lipex Biomembranes Extruder (Vancouver, Canada) composed of one pre-filter and two stacked polycarbonate membranes with a pore size of 0.1 μm (Polycarbonate filter Lipex Biomembranes, Vancouver, Canada). The actual phospholipid content of each preparation is determined by phosphorous assay [32].
4.2.3 Membrane permeabilization
Membrane permeabilization was followed as described by Van Bambeke et al. [33]. Release of 8-hydroxypyrene-1,3,6 trisulfonic acid (HTPS) coentrapped with and quenched by p-xylene-bis-pyridinium bromide (DPX) from liposomes can be monitored by the fluorescence increase upon dilution following their leakage from the vesicles. The dried lipid films were hydrated with a solution of HTPS and DPX in a 40 mM glycine-NaOH mixture adjusted to pH 11 at a concentration of 31.8 and 35 mM, respectively. After formation of LUV, the unencapsulated dye was eliminated by passage on a sephadex G75 column. The liposomes were diluted to a final lipid concentration of 25 μM in Tris 10 mM, NaCl 150 mM buffer pH 7.4. C18sat or C18unsat was added and fluorescence intensities were immediately recorded. The percentage of HTPS released was defined as [(Ft − Fcontr)/(Ftot − Fcontr)] × 100, where Ft is the fluorescence signal measured after 15 min in the presence of C18sat or C18unsat, Fcontr is the fluorescence signal measured at the same time for control liposomes, and Ftot is the total fluorescence signal obtained after complete disruption of the liposomes by 0.05% Triton X-100. All fluorescence determinations were performed at room temperature on a Perkin Elmer LS-50B Fluorescence Spectrophotometer (Perkin-Elmer Ltd., Norwalk, CT, USA.) using λexc of 450 nm and a λem of 512 nm.
4.2.4 Fusion of lipid phases
The fusion of lipid phases was determined by measuring the dequenching of the fluorescence of octadecylrhodamine B chloride (R18) according to Hoekstra et al.’s method [28]. The fluorescence of this lipid-soluble probe is self-quenched as a function of its membranous concentration and any decrease of its surface density is therefore associated with a commensurate increase of the fluorescence intensity of the preparation. Labeled LUV liposomes were obtained by incorporating R18 in the dry lipid film at a molar ratio of 5.7% w with respect to the total lipids. The liposomes were diluted to a concentration of 25 μM in Tris 10 mM, NaCl 150 mM buffer pH 7.4. These labeled liposomes were mixed with unlabeled LUV liposomes (adjusted to the same concentration) at a ratio of 1:4. C18sat (or C18unsat) was added and the fluorescence was then followed at room temperature during 15 min, using a λexc of 560 nm and a λem of 590 nm (Perkin-Elmer LS-50B, Perkin-Elmer Ltd, Norwalk, CT, USA). Results were expressed as the fluorescence signal recorded for samples incubated with C18sat (or C18unsat) after 15 min from which the fluorescence signal, recorded at the same time for a control (without bolaform), was subtracted.
Acknowledgements
This work was supported by the CPER Program “Pentoraffinerie”. We are grateful to the public authorities of Champagne-Ardenne and the FEDER for material funds. M.D. and L.L. thank the F.R.S.-F.N.R.S. (National Funds for Scientific Research, Belgium) for their research associate and senior research associate positions, respectively. K.N. thanks the Superzym ARC grant, financed by the French Community of Belgium.