1 Introduction
Methamphetamines are psychostimulant and sympathomimetic drugs. They are used recreationally, but also as medications for medical conditions and for weight loss. From 1983 to 2005, many laws were passed that made it a crime to possess equipment for methamphetamine production and chemicals that can be used to synthesize them. In order to circumvent these laws, illicit or clandestine laboratories were used, where cold medications serve as precursors to methamphetamine. The Patriot Act includes a section called the Combat Methamphetamine Epidemic Act of 2005, a law which regulates the sale of these medications and limits how much non-prescription cold medicine can be bought at a time.
There are several methods of producing methamphetamines. One of the many dangers involved in this production, is the release of harmful chemicals such as phosphine and methylamine, which are dangerous to those in the surrounding area, by causing harm to the skin, eyes, and respiratory tract. These chemicals are also very harmful to the environment, as for every pound of methamphetamine produced, there are more than five pounds of toxic waste that go into rivers or the sewer system.
The chemicals used in methamphetamine production can also lead to dangerous explosions. About 15% of methamphetamine labs are found out because of a fire or explosion.
There have been 7347 incidents of methamphetamine clandestine laboratories in the United States in 2006. The greatest numbers were found in Missouri, Illinois, and Indiana.
Methamphetamines are also produced in other countries, for instance, the Netherlands, Belgium, and Australia [1]. One of the clandestine laboratories in Australia utilized Wacker oxidation of safrole to 3,4-methylenedioxyphenyl-2-propanone (MDP-2P) using palladium chloride, p-benzoquinone and methanol.
A number of studies discuss methods of synthesizing MDMA (a designer drug known also as “ecstasy” or “XTC”) from the point of view of identifying intermediates and by-products which would facilitate the recognition of illegal MDMA production in the suspected laboratory, as well as provide information about the synthetic route employed [2].
Salouros et al. [2] describe the synthesis reaction, with the obtaining of the by-product N-cyanomethyl-N-methyl–1-(3,4-methylenedioxyphenyl)-2-propylamine. This work applies quantum chemical methods to the study of the energetics of the synthesis reactions, as well as of the properties of possible intermediates and by-products. Those properties include infrared frequencies, knowledge which might help in the identifications of different structures. Indeed, Salouros et al. [2] isolated the compound identified tentatively by Swist et al. [3] as 2-dimethylamino-2-methyl-3-(3′,4′-methylenedioxyphenyl)-2-propanenitrile. Salouros et al. [2] elucidated the structure of this compound, using NMR, as N-cyanomethyl-N-methyl-1-(3′,4′-methylenedioxyphenyl)-2-propylamine. Experiments performed by Salouros et al. [2] support Swist's conclusion that this compound is a marker for the cyanoborohydride route of synthesis.
This work investigates both possible structures of the marker via the calculations of infrared frequencies. The present work also investigates 3′,4′–methylenedioxy-methamphetamines and related compounds with quantum chemical calculations.
Other methods of identification for 3′,4′-methylenedioxy-methamphetamines and related compounds include: thin layer chromatography/fluorescence [4], gas chromatography with mass spectrometry (GC-MS) [5], liquid chromatography with fluorescence detection [6], liquid chromatography with mass spectrometry (LC-MS) [7], and immunoassay [8]. Recently, MDMA has also been identified in the urine of users via NMR spectroscopy [9].
2 Methods and results
Density Functional Theory (DFT) was used to perform quantum chemical calculations at B3LYP level, with the 6-31G** basis set, as implemented by the Spartan computer program [10]. In order to investigate the necessity of diffuse functions, the reaction (1), was also investigated with the addition of diffuse functions. The results were found to be within 2.5 Kcals/mol from the results without diffuse functions. Therefore, it was deemed that it is not necessary to use diffuse functions. The entities studied are: the reaction of synthesis of MDMA, and a number of analogs of methamphetamine. These analogs have been chosen from the experimental report of Kato et al. [4].
The energetics of the reaction:
(1) |
Synthesis of MDMA: energies of the reactant, 3,4-methylenedioxyphenyl-2-propanone (1); the intermediate, N-methyl-1-(3,4-methylenedioxyphenyl)-2-propylimine (2); the product, 3,4-methylenedioxymethamphetamine MDMA (3) and by products (4) and (5).
Compound | Energy (au) | Zero-point energy (Kcal/mol) | Energy of solvation (Kcal/mol) |
1 | −612.74721 | 113.9 | −8.8 |
2 | −632.17683 | 139.1 | −9.0 |
3 | −633.39527 | 154.7 | −6.0 |
4 | −764.93053 | 171.5 | −8.6 |
5 | −764.93036 | 171.3 | −7.9 |
CH3NH2 | −95.86137 | 40.3 | −6.3 |
H2O | −76.42257 | 13.1 | |
H2 | −1.17850 | 6.4 | |
ClCH2CN | −592.33926 | 23.18 | −4.5 |
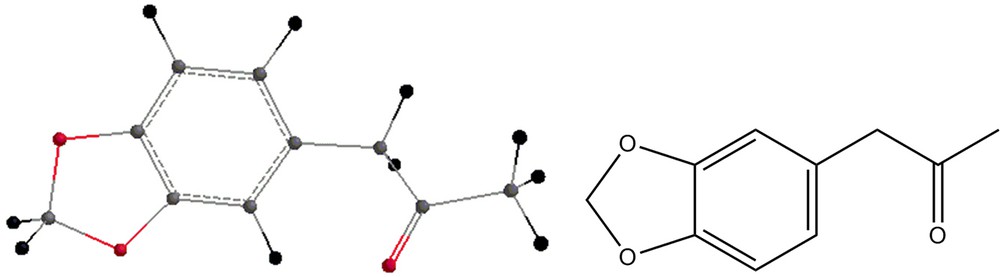


Some by-products of the reaction are shown in Figs. 4–7.
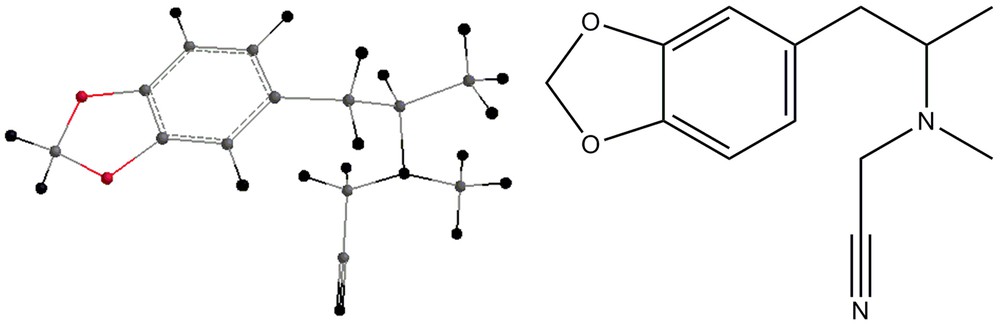

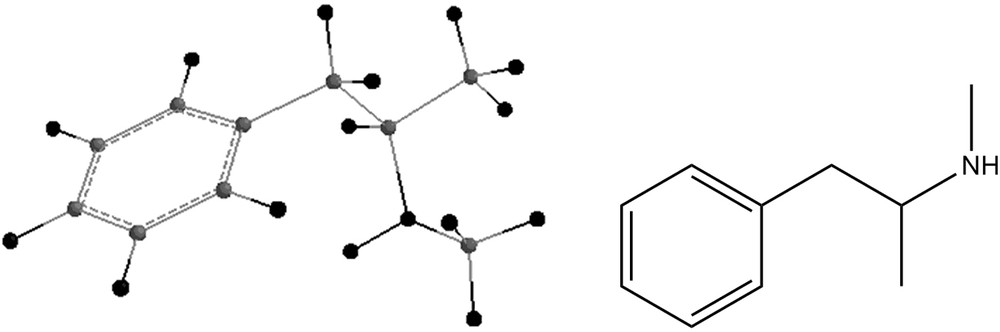
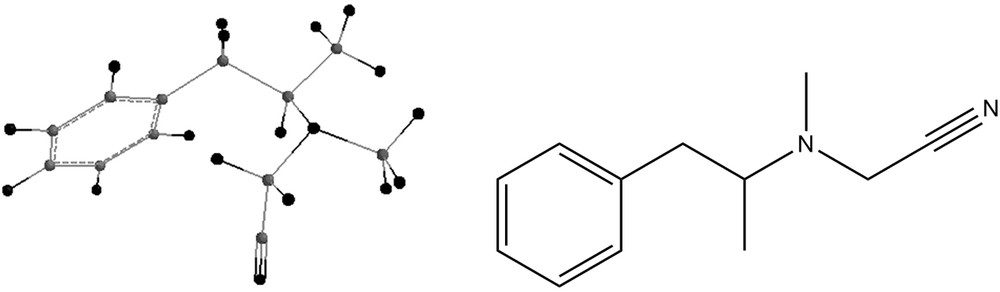
The energies of the reactions of formation of compounds 4 and 7 were also investigated and their energies were calculated:
(2) |
(3) |
The methamphetamine analogs shown in Figs. 8–11 have also been geometry-optimized and their energies are displayed in Table 2. Tables 1 and 2 display also zero-point energies and solvation energies, the latter being calculated by the semi-empirical methods [11]. Infrared vibrational frequencies were calculated for all the structures mentioned above. In order to examine the necessity of scaling the frequencies, the experimental spectrum of dimethylmethamphetamine was compared with the spectra calculated using both HF/6-31G** and B3LYP/6-31G** methods. While the HF results showed clearly the necessity of scaling, the frequencies being larger than the experimental ones, that was not the case with the B3LYP results, where the experimental frequencies and the calculated ones were very close (within the first decimal). Table 3 shows the frequencies and the intensities of the infrared peaks of Compound 2. In addition, the calculated drawn spectrum is shown. Table 4 shows the frequencies and intensities of the by-product 4, while those of compound 5 are shown in Table 5. Both spectra of the compounds shown are drawn. Table 6 shows the frequencies and intensities of the methamphetamine analogs depicted in Figs. 8 and 9, and Table 7 shows the infrared frequencies and intensities of compounds 10 and 11. Table 8 shows the frequencies and intensities of compound 7.
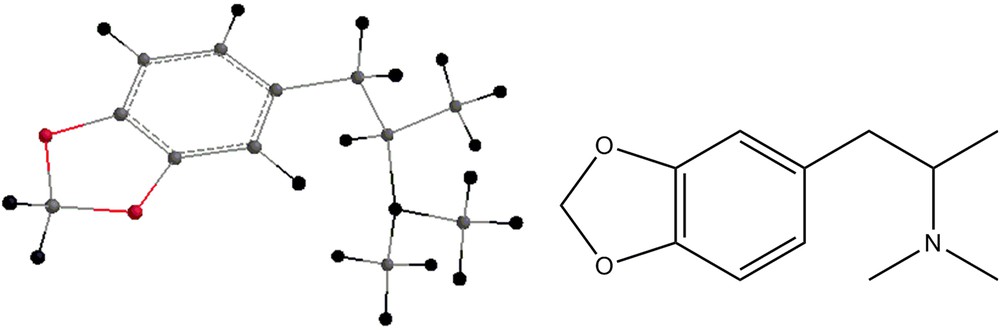
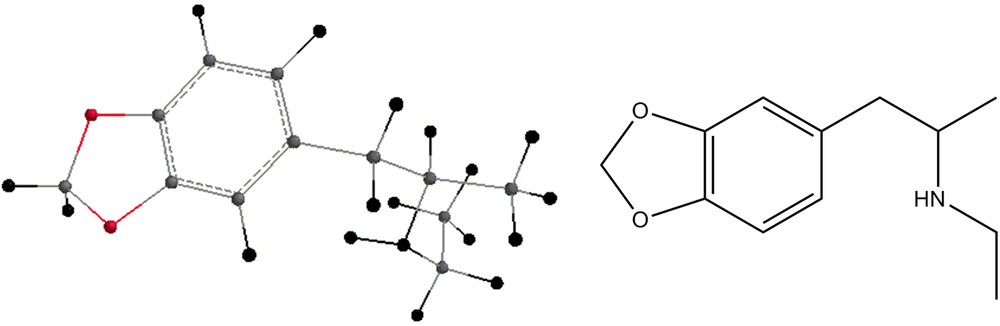
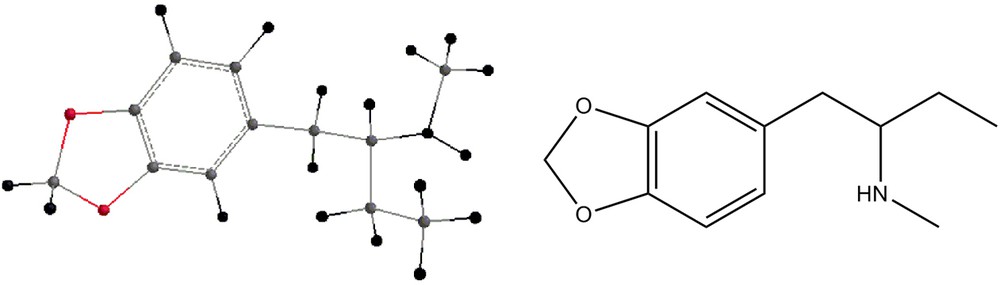

Energies of compounds 6, 7, 8, 9, 10 and 11.
Compound | Energy (au) | Zero-point Energy | Solvation Energy |
6 | −444.86572 | 145.1 | −2.8 |
7 | −576.40626 | 161.7 | −3.4 |
8 | −672.70090 | 172.2 | −4.5 |
9 | −672.71270 | 172.7 | −5.1 |
10 | −672.70880 | 165.3 | −6.5 |
11 | −672.70792 | 172.7 | −6.5 |
HCl | −460.80080 | 4.2 | −0.7 |
Frequencies and intensities of the infrared peaks of compound 2.
Frequencies (cm−1) | Intensities |
3106.2 | 54.3 |
3160.8 | 12.7 |
3088.2 | 21.5 |
3070.8 | 52.5 |
3008.2 | 139.8 |
2998.8 | 54.2 |
1754.6 | 92.7 |
1537.3 | 276.3 |
1487.1 | 40.0 |
1325.3 | 22.6 |
1294.0 | 235.3 |
1265.1 | 58.6 |
1193.0 | 23.3 |
1070.0 | 118.6 |
960.2 | 23.8 |
947.8 | 24.8 |
Frequencies and intensities of the infrared peaks of by-product 4.
Frequencies (cm−1) | Intensities |
3114.8 | 34.4 |
3097.5 | 59.8 |
3010.8 | 136.1 |
2957.4 | 91.7 |
2945.8 | 43.9 |
2371.7 | 2.0 |
1538.7 | 229.0 |
1506.1 | 23.0 |
1487.5 | 55.6 |
1304.4 | 64.2 |
1296.7 | 207.1 |
1223.4 | 29.4 |
1154.9 | 24.7 |
1072.1 | 131.6 |
978.8 | 29.2 |
821.9 | 26.9 |
Frequencies and intensities of the infrared peaks of by-product 5.
Frequencies (cm−1) | Intensities |
3152.3 | 32.8 |
3012.0 | 132.9 |
2983.8 | 58.6 |
2975.1 | 36.3 |
2335.0 | 2.7 |
1560.0 | 10.6 |
1519.8 | 15.2 |
1487.4 | 57.0 |
1261.5 | 26.4 |
1224.3 | 22.7 |
1138.8 | 42.9 |
1070.6 | 122.6 |
1000.4 | 35.4 |
956.5 | 31.6 |
Frequencies and intensities of the infrared peaks of by-products 8 and 9.
Compound 8 | Compound 9 | ||
Frequencies (cm−1) | Intensities | Frequencies (cm−1) | Intensities |
3141.8 | 30.6 | 3136.2 | 30.0 |
3129 | 32.1 | 3123.7 | 32.1 |
3049 | 29.5 | 3108.6 | 43.8 |
3009 | 141.6 | 3100.2 | 53.4 |
2936.3 | 116.8 | 3008.4 | 151.3 |
2923.8 | 55.9 | 2925.5 | 112.4 |
2898.1 | 42 | 1539.4 | 236.5 |
1536 | 253.5 | 1487.1 | 67.0 |
1492 | 30.7 | 1304.4 | 70.3 |
1372.2 | 32.5 | 1210.0 | 36.8 |
1292.3 | 130.6 | 1164.2 | 40.9 |
1291.7 | 155.5 | 1086.1 | 43.2 |
1073.1 | 82.8 | 1069.9 | 101.8 |
1067.1 | 37.8 | 971.4 | 33.5 |
974.6 | 36.5 |
Frequencies and intensities of the infrared peaks of by-products 10 and 11.
Compound 10 | Compound 11 | ||
Frequencies (cm−1) | Intensities | Frequencies (cm−1) | Intensities |
3128.4 | 31.0 | 3134.0 | 27.8 |
3110.1 | 38.2 | 3117.9 | 35.2 |
3096.6 | 57.1 | 3105.4 | 41.9 |
3080.2 | 50.2 | 3102.0 | 57.5 |
3009.7 | 145.1 | 3095.5 | 37.2 |
2942.8 | 100.1 | 3077.9 | 38.7 |
2900.9 | 56.6 | 3040.8 | 36.6 |
1539.3 | 244.1 | 3004.9 | 142 |
1486.5 | 43.0 | 2945.3 | 97.3 |
1346.8 | 26.5 | 2896.4 | 62.5 |
1310.1 | 67.1 | 1537.8 | 245.8 |
1282.3 | 157.9 | 1486.4 | 49.5 |
1145.0 | 31.0 | 1402.8 | 28.0 |
1071.6 | 126.1 | 1298.8 | 59.8 |
971.0 | 39.3 | 1295.5 | 142.7 |
1284.2 | 64.5 | ||
1220.6 | 41.0 | ||
1169.2 | 39.4 | ||
1071.2 | 70.0 | ||
1069.1 | 52.0 | ||
973.6.0 | 31.2 | ||
774.2 | 68.1 |
Frequencies and intensities of the infrared peaks of by-product 7.
Frequencies (cm−1) | Intensities |
3047.7 | 35.9 |
2955.2 | 89.7 |
2929.7 | 49.4 |
1403.6 | 20.4 |
1172.4 | 30.0 |
1055.2 | 28.7 |
736.9 | 23.0 |
Calculated drawn spectrum for compound 2.
Calculated drawn spectrum for compound 4.
Calculated drawn spectrum for compound 5.
Calculated drawn spectrum for compound 7.
3 Discussion
The reaction of synthesis of metamphetamine, compound shown in Fig. 3, features two steps:
- 1. the reaction of MDP-2P (Fig. 1) with a reagent such as methylamine, leading to the formation of the intermediate shown in Fig. 2 and water;
- 2. the reduction of the intermediate by a hydrogen molecule, leading to the formation of methamphetamine.
The energetics of these reactions, calculated as shown in Methods with the DFT method (B3LYP/6-31G**) shows the first reaction to be endothermic, with an energy of 5.8 Kcal/mol and the second reaction to be exothermic, with an energy of −25.1 Kcal/mole. As such, the overall energy of the synthesis of metamphetamine is of −19.3 Kcal/mole. If the solvation energy is taken into account, since the solvation energy of MDMA is smaller by 2 Kcal/mol than the one of the reactant (compound 1), the total energy of the reaction is of −17 Kcals/mol. The differences in zero-point energies between reactant and products are small, therefore negligible.
Salouros et al. [2] propose as a by-product of these reactions the formation of N-cyanomethyl-N-methyl-1-(3′,4’methylenedioxyphenyl)-2-propylamine, as shown in Fig. 4. According to their NMR studies, the structure shown in Fig. 4 is the right one for this by-product, but this might be reinforced by examining the IR results. In order to confirm or infirm this possibility, this work calculated the infrared vibrational modes for this compound, after subjecting it to geometry optimization. Table 4 shows these values.
Swist et al. [3] propose as a by-product 2-dimethylamino-2-methyl-3-(3,4-methylenedioxyphenyl)-propanenitrile. This compound, as shown in Fig. 5 was also geometry-optimized and its infrared vibrational frequencies were calculated. These results are shown in Table 5. Examining Tables 4 and 5, one notices that in both compounds the nitril vibration shows a very low intensity. Both compounds show strong absoptions in the 3000 cm−1 region, as well as a strong peak at 1070 cm−1. Compound 4 shows a very strong absorption at 1538 cm−1, which seems to be absent in Compound 5. Another significant difference is the strong band at 1297 cm−1, present in 4 and absent in 5.
The reaction of synthesis of the compound 4 is found to be slightly endothermic, with a reaction energy of 2 Kcal/mol. Since the solvation energy difference between product and reactant is of −2 Kcal/mol, this reaction does not occur with a change in energy.
The reaction of synthesis of compound 7 is slightly exothermic, with a reaction energy of −1.32 Kcal/mol. The solvation energy in this reaction is of only 0.6 Kcals/mol.
Table 1 shows the energies of the investigated compounds and it is clear that all are minima in energy, that is, they are stable compounds. That can be seen from the fact that all the infrared frequencies are positive.
Tables 3–8 display the infrared frequencies of all the compounds discussed. The experimentalists could take the infrared spectrum of an unknown compound suspected to be a marker, a by-product or an intermediate in the synthetic route and compare the data with the quantum chemical obtained frequencies of the suspected structure in order to obtain some information about its structure.
In conclusion, the calculations can serve to help identify the structure of the investigated compounds.