1 Introduction
1,3,4-Oxodiazole derivatives are highly attractive compounds for the development of materials for organic electroluminescent (EL) devices since they possess high electron-accepting properties and display strong fluorescence with high quantum yield [1]. This behavior is exemplified by 2,5-diphenyl-1,3,4-oxodiazole and 2,5-di-2-naphthyl-1,3,4-oxodiazole, for which quantum yields of 0.80 and 0.85 in cyclohexane solution, respectively, were reported [2]. Thus, compounds involving 1, 3,4-oxadiazole rings have been used as electron transporting materials and emitters in organic EL devices [3–5]. Recently 1,3,4-oxodiazole derivatives have aroused considerable interest in the area of organic light-emitting diodes (OLEDs) [6–9]. Oxadiazole fragments have also been connected to classical chelating ligands (such as bipyridines) in luminescent complexes, to obtain multifunctional (emitting and charge transporting) molecular species [10–17]. Furthermore, it has been reported that substituted 1, 3,4-oxadiazole derivatives show a broad spectrum of biological activity including anticancer effects [18,19]. In recent years, the application of 1,3,4-oxodiazole consisting of five membered heterocyclic ring have been described [20–24].
On the other hand, coumarins and structurally related compounds have been shown to inhibit replication of HIV and thus exhibit a therapeutic potential [25]. A large number of structurally novel coumarin derivatives have been reported to show substantial cytotoxic and anti-HIV activity in vitro and in vivo [26,27]. A variety of synthetic coumarins have unique mechanisms of action referring to the different stages of HIV replication [28]. Thus, coumarins are important lead compounds for the development of antiviral and/or virucidal drugs against HIV [29–31].
Keeping in view of the properties of 1,3,4 oxodiazole derivatives and in prolongation of our research on biologically active molecules [32,33], we have carried out the present study to describe new convenient and general procedures to afford novel 2-[(coumarin-4-oxy)methyl]-4-acetyl-5-substitued–1,3,4-oxodiazole 5 containing 4-hydroxycoumarine moieties and investigate both their electrochemistry and antimicrobial properties against Staphylococcus aureus (CIP 7625), S. aureus, Escherichia coli ATCC 25922, Klebsiella pneumonia CIP 104727 and Pseudomonas aeruginosa 27853 (CIP 76110) using the agar disk diffusion assay. The antioxidant properties of these compounds have been studied using two different test methods, namely 2,2-diphenyl-1-picrylhydrazyl and ABTS radicals, respectively. The differences of radical scavenging and antioxidant properties of 2-[(coumarin-4-oxy) methyl]-4-acetyl-5-substitued–1,3,4-oxodiazole were compared with similar doses of Trolox, a standard antioxidant commonly used in food and pharmaceutical industries.
2 Results and discussion
Compound 2 was prepared by reaction of 4-hydroxycoumarine with ethylbromoacetate in the presence of anhydrous potassium carbonate in dry acetone, followed by refluxing with hydrazine hydrate in absolute ethanol, generating a colorless crystalline product for which structure 3 was assigned.
The resulting of carbohydrazide 3 was reacted with arylaldehydes to give rise the (E)2-(coumarin-4-oxyacetic)-N-benzylideneacetohydrazide 4, which precipitated by mixing the carbohydrazide 3 and the corresponding ArCHO in hot ethanol.
The obtained compounds 4 were then refluxed with acetic anhydride to give the corresponding 4-(4-acetyl-5- substituted -4, 5-dihydro-1,3,4-oxodiazol-2-yl)methoxy)-2H-chromen-2-ones 5 in good yields according to Scheme 1.
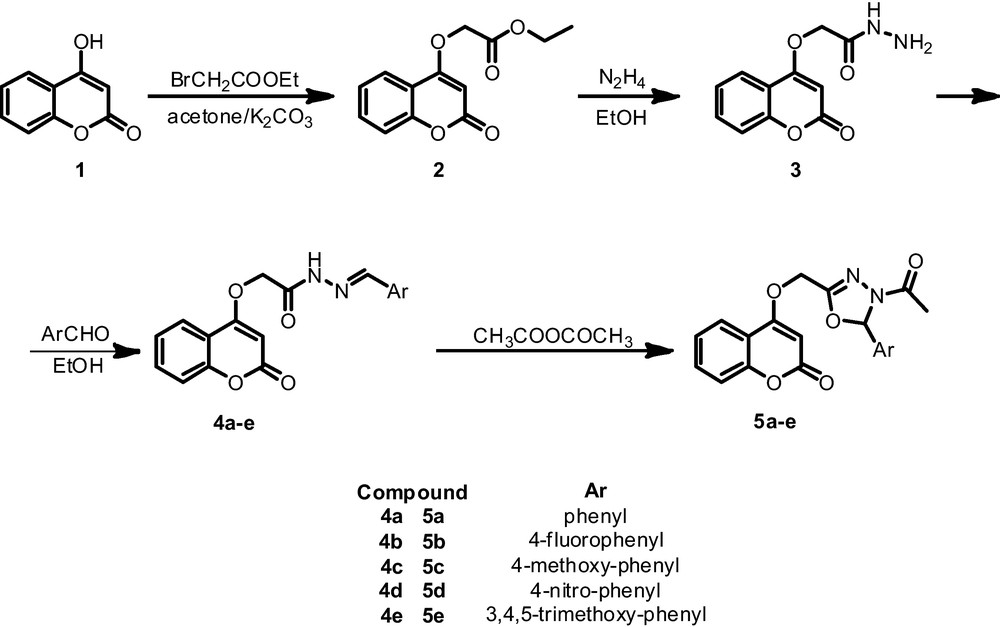
Synthesis of 4-(4-acetyl-5- substituted -4, 5-dihydro-1, 3,4-oxodiazol-2-yl)methoxy)-2H-chromen-2-ones5.
All the new 4-(4-acetyl-5- substituted -4, 5-dihydro-1,3,4-oxodiazol-2-yl)methoxy)-2H-chromen-2-ones 5, have been characterized by UV, IR, 1H, 13C-NMR spectra as well as by NOESY and HMBC NMR experiments to elucidate their structures and assign completely the structural network of both protons and carbons. The obtained spectral data were in accordance with the proposed structures.
As example, the IR spectra of compound 5c showed the characteristic absorption bands for 1612(CC), 1472(NN) and 1289(COC). In addition, the detection of a strong CN stretching band at 1555 cm−1 evidenced the formation of the 1,3,4-oxodiazol ring. The 1H NMR spectra of 5c displays a signal at δ 6.07 ppm that ascribable to the proton H-3 from the coumarine moiety. A characteristic singlet proton signal at δ 8.53 ppm was assigned to H-5’ proton from the oxodiazole fragment. In addition, the aromatic protons (both coumarinic and oxodiazolinic) are observed between δ 7.08 and δ 7.91 ppm (see experimental), and the expected singlet for methylenic moiety is observed at δ 5.47 ppm. The methyl protons of the acyl group arose at δ 2.39 ppm. The singlet at 3.84 ppm was assigned to the three protons of the methoxy group. Full assignment of the 1H NMR spectra of 5c was deduced from the NOESY spectrum. An observed NOE cross peak between both H-5’ and methylic proton CH3 placed these two units on the same side of the oxodiazole ring while H-3 and CH2 are, therefore, on the other side. The 13C{1H} NMR spectrum of 5c in DMSO-d6 showed two downfield signals at δ 166.7 ppm (C2’) and δ 162.0 ppm (lactone C2O) as well as a signal to up field at δ 55.9 ppm (OCH3). The signals at δ 26.0, δ 91.9 and δ 168.1 ppm were assigned to the COCH3, the C3 and C5’, respectively.
The structure of 5c was finally elucidated through the analysis of the 1H, 13C HMBC spectrum (Fig. 1), which indicates that the methylenic protons at δ 5.47 ppm correlate with C2’ (δ 166.7 ppm) and C4 (δ 164.8 ppm). The hydrogen atom H-5’, at 8.53 ppm, correlate with Ca (δ 125.5 ppm). Moreover, the proton H-3 (δ 6.07 ppm) correlates with C4 (δ 164.8 ppm). Furthermore, methylic protons CH3CO (δ 2.39 ppm) and the CO carbon signal (δ 171.2 ppm) are also correlated.

1H 13C HMBC spectrum of compound 5c.
Assignment of the protons of 2-[(coumarin-4-oxy) methyl]-4-acetyl-5-substitued–1,3,4-oxodiazole 5 was deduced from their NOESY spectra. An observed nOe cross peak between H5’ and methylic proton CH3 places these two units on the same side of the average oxadiazoline ring plane. H3 and Hb are therefore on the other side of this plane.
A mechanistic rationalization for this reaction is provided in Scheme 2.
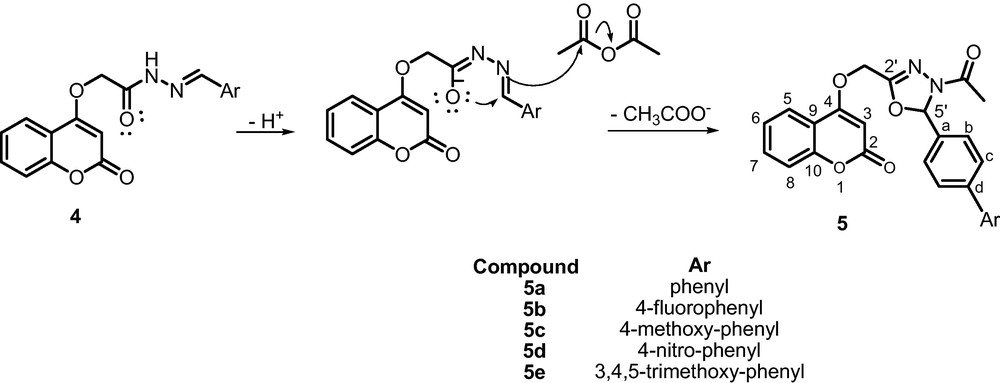
Proposed mechanism for the synthesis of 5a-e.
The formation of the 4-(4-acetyl-5- substituted -4, 5-dihydro-1,3,4-oxodiazol-2-yl)methoxy)-2H-chromen-2-ones 5 occurred via two steps: the carbohydrazide 3 initially formed rearranges to generate a negative oxygen which then attacks on the CN bond, followed by attack of the nitrogen atom to the CO bond of the acetic anhydride accompanied by the loss of acetic acid yielding the 4-(4-acetyl-5- substituted -4, 5-dihydro-1,3,4-oxodiazol-2-yl)methoxy)-2H-chromen-2-ones 5.
3 Absorption spectral characteristics of the compounds 5a–e
The UV spectra of the compounds 5 measured in methanol solutions are shown in Fig. 2. As shown in this figure compounds 5 display similar absorptions ranging from 200 to 350 nm that are attributed to π- π* transition of conjugate system.
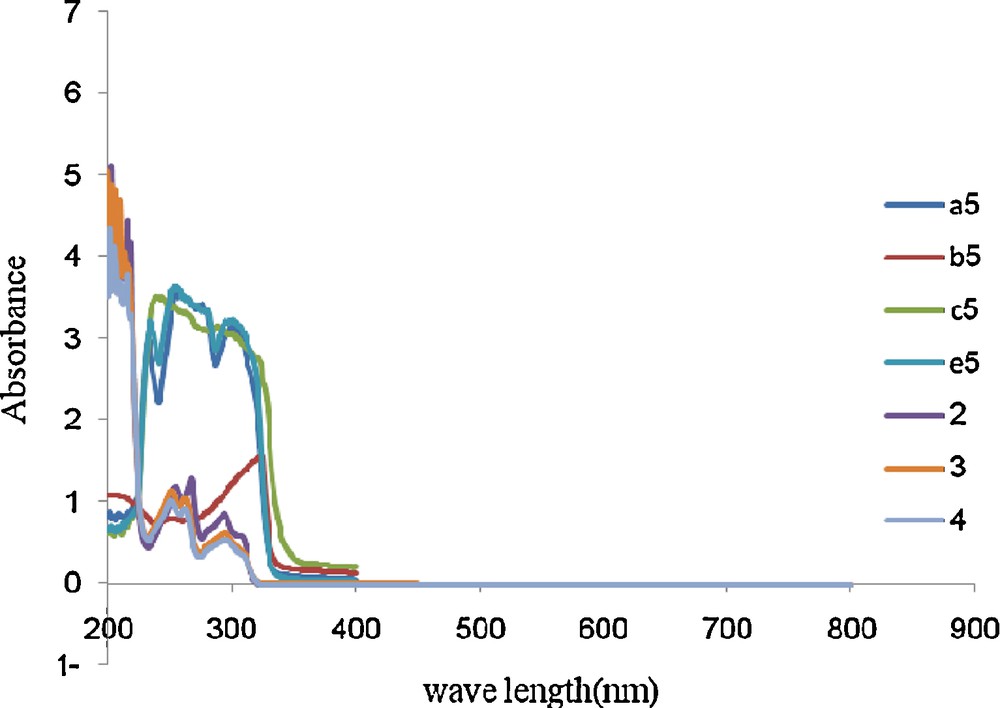
The UV spectra of the compounds 5a–d and 2–4 in methanol (2 10−5 M).
The lowest energy absorption bands are from the π- π* transitions by virtue of their large molar extinction coefficients. Two absorption bands were observed for 5a and 5e respectively, while only one was observed for 5b.
Comparing compounds 5b and 5e, electron-withdrawing group (F) result in a blue shift (6 nm) with contrast to an electron-donating group (3.4.5 MeO). Compounds 5 have almost same maximum absorption (200–350 nm) that means substituent R in para position does not influence the absorption.
4 Electrochemistry
Electrochemical studies of 4-(4-acetyl-5- substituted -4, 5-dihydro-1, 3,4-oxodiazol-2-yl)methoxy)-2H-chromen-2-ones 5 are of interest due to the electron-deficient nature of the oxadiazole unit.
Hence, the electrochemical properties of compounds 5a–e, were determined by cyclic voltammetry in CH3CN (1 × 10−3 M) solutions, using 0.1 M tetrabutylammonium bromide (C4H12BrN) as the supporting electrolyte. Both platinum and gold were used as working electrodes, Ag/AgCl (0.1 M) as the reference electrode, and platinum as the counter electrode. Under these electrochemical conditions, 5 show a quasi-reversible behavior for the first reduction process. This can be deduced from the fact that the cathodic–anodic peak separations (Epc–Epa) are ca. 100 mV. The ratio of the peak current intensity for the cathodic and anodic processes is about 0.5–0.7. As expected, the reduction peak potential of the 2-[(coumarin-4-oxy) methyl]-4-acetyl-5-substitued–1,3,4-oxodiazole 5 are strongly influenced by the para substitutent on the phenylene ring. Compared to the unsubstituted compound 5, the methoxy group, having a slight electron donor behavior, shifts the reduction peak potential of 5 to more negative values. The redox behaviors of all the 1,3,4-oxadiazolines are summarized in Fig. 3. All the compounds exhibited one quasireversible redox processes.
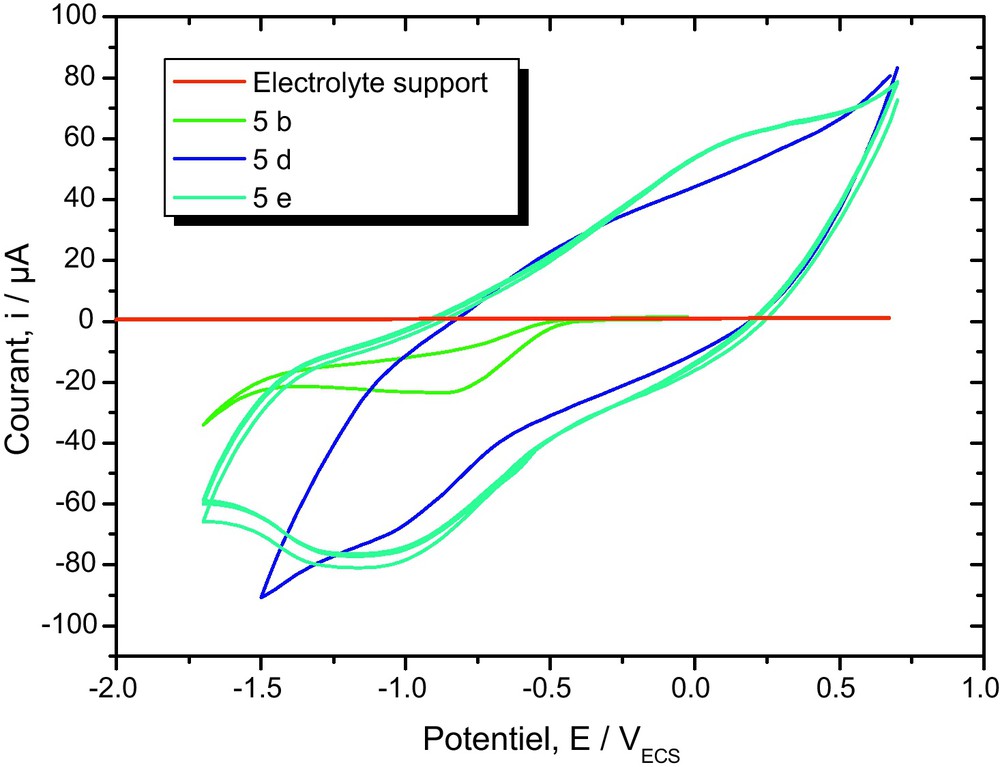
Cyclic voltammograms of compounds 5d, 5b and 5e (1 × 10−3 M) in CH3CN, scanned at 100 mVs−1.
For example, compounds 5d showed one quasireversible reduction at −0.8 and −1.15 V, respectively. We assume that the curve at lower reduction potential may be due to the more electron-deficient dications in the ring system, and the curve at higher reduction potential can be attributed to the redox behavior of the oxadiazole unit.
5 Antibacterial and antioxidant studies
5.1 Free radical scavenging activity assay
The free radical scavenging activity of the new 4-(4-acetyl-5- substituted -4, 5-dihydro-1,3,4-oxodiazol-2-yl)methoxy)-2H-chromen-2-ones 5 was tested by utilizing DPPH scavenging [34].
DPPH is a free radical and accepts one electron or one-hydrogen radical to become a stable diamagnetic molecule [35]. The reduction capability of DPPH radical was determined by the decrease in absorbance induced by 4-(4-acetyl-5- substituted -4, 5-dihydro-1,3,4-oxodiazol-2-yl)methoxy)-2H-chromen-2-ones 5. Briefly, 1.5 ml ethanolic solution of the synthesized compounds (0.2 mM) was added to 1.5 ml (0.2 mM) solution of DPPH radical in ethanol (final concentration of DPPH and synthesized compounds was 0.1 mM). The mixture was shaken vigorously and allowed to stand for 30 min. After this, the absorbance at 534 nm was determined and the percentage of scavenging activity was calculated using the following formula:
Ab: absorbance of 0.1 mM ethanolic solution of DPPH at 534 nm, As: absorbance of 0.1 mM ethanolic solution of test compound at 534 nm, Am: absorbance of ethanolic mixture of the drug and DPPH at 534 nm.
Trolox was used as reference compound. All tests and analyses were undertaken and averaged on three samples. The results are given in Scheme 3.
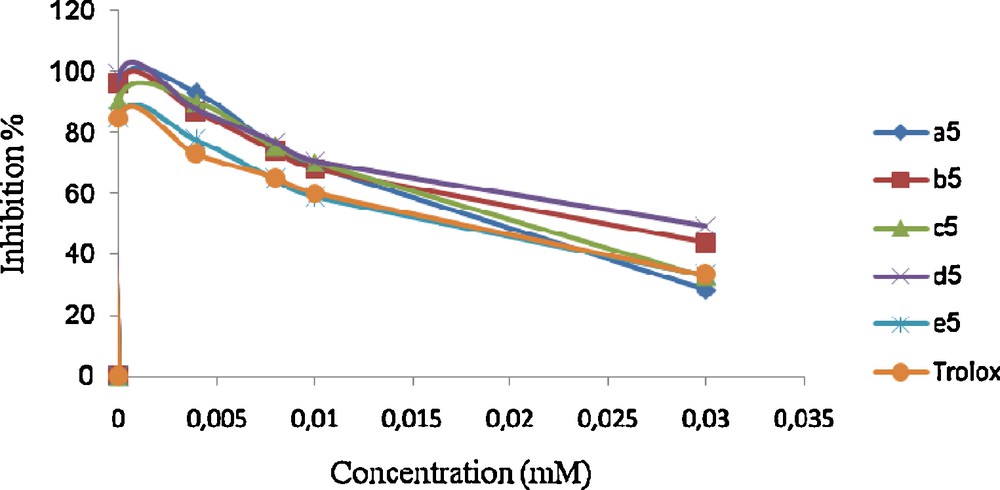
Scavenging effect on 1,1-diphenyl-2-picrylhydrazyl (DPPH) radical of compounds 5.
Among the compounds from the 4-(4-acetyl-5- substituted -4, 5-dihydro-1,3,4-oxodiazol-2-yl)methoxy)-2H-chromen-2-ones 5 series 5b showed moderate antioxidant activity.
The activity exhibited by the compound 5e was the highest. In addition the experimental data show that compound 5a has a stronger effect of scavenging free radical than Trolox.
According to the experimental results, we can note that any increase in the concentration of the obtained 1,3,4 oxodiazole in the medium involves an attenuation of percentage of inhibition. Indeed, this last can reach 90% for a concentration about 1 μM for all the synthesized products. Thus, we can conclude that substituent's on the aryl group does not have an influence on the anti-oxidant activity.
One parameter that has been introduced recently for the interpretation of the results from the DPPH method is the efficient concentration or EC50 value (otherwise called the IC50 value); this is defined as the concentration of substrate that causes 50% loss of the DPPH activity (color), and it is corresponding to the endpoint of the titration. It should be noted that in all cases, any residual (yellow) color from the reduced form or any non specific absorbance from the sample has to be taken into account in defining the “endpoint” of the titration, or the 50% point, this IC50 parameter also has the drawback that the higher the antioxidant activity, the lower is the value of EC50.
The EC50 values exhibited by 4-(4-acetyl-5- substituted -4, 5-dihydro-1,3,4-oxodiazol-2-yl)methoxy)-2H-chromen-2-ones 5 are summarized in Table 1.
The EC50 values exhibited by 4-(4-acetyl-5- substituted -4, 5-dihydro-1, 3, 4-oxodiazol-2-yl)methoxy)-2H-chromen-2-ones 5.
Compounds 5a–e | EC50 (mmol.l−1) |
5a | 0.00521 |
5b | 0.005217 |
5c | 0.00551 |
5d | 0.00506 |
5e | 0.00591 |
Trolox | 0.005879 |
It was found that oxodiazoles 5a–d possess higher activity than Trolox with EC50 value of 0.00521, 0.005217, 0.00551and 0. 00506 mmol.l-1 respectively followed by 5e with EC50 value of 0.00591. The low activity of the compound 5e is attributed to the methoxy groups.
5.2 ABTS radical cation decolorization Assay
The potential of 4-(4-acetyl-5- substituted -4, 5-dihydro-1,3,4-oxodiazol-2-yl)methoxy)-2H-chromen-2-ones 5 to scavenge free radicals was also assessed by their ability to quench ABTS+. Scheme 4 depicts the concentration-dependent decolourization of ABTS+.
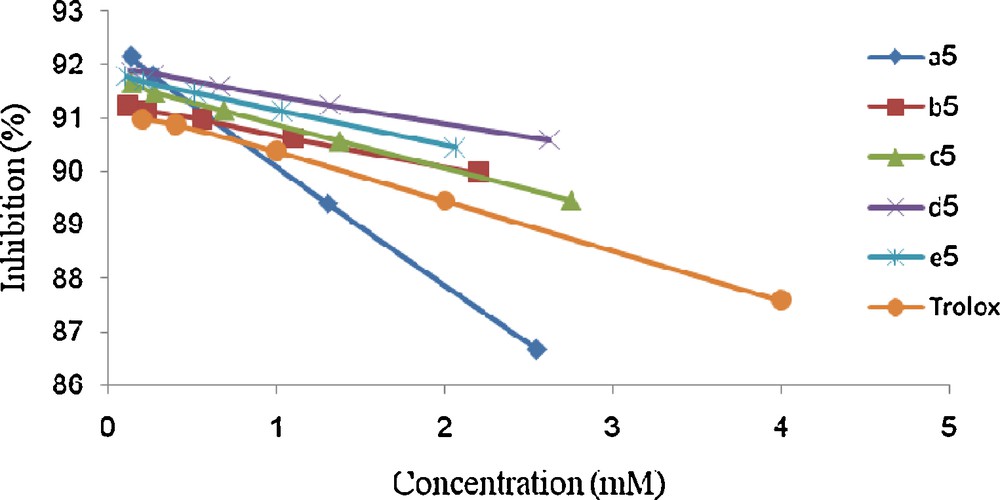
Scavenging ability on ABTS radical of compounds 5.
ABTS radical-scavenging activity of 4-(4-acetyl-5- substituted -4, 5-dihydro-1,3,4-oxodiazol-2-yl)methoxy)-2H-chromen-2-ones 5 was determined according to Re et al. [30]. The ABTS+.. Cation radical was produced by the reaction between 5 ml of 14 mM ABTS solution and 5 ml of 4.9 mM potassium persulfate (K2S2O8) solution, stored in the dark at room temperature for 16 h. Before use, this solution was diluted with ethanol to get an absorbance of 0.700 ± 0.020 at 734 nm. In a final volume of 1 ml, the reaction mixture comprised 950 μl of ABTS± solution and 50 μl of the 1,3,4 oxadiazoles 5 at various concentrations. The reaction mixture was homogenized and its absorbance was recorded at 734 nm. Ethanol blanks were run in each assay, and all measurements were done after at least 6 min. Similarly, the reaction mixture of standard group was obtained by mixing 950 ll of ABTS+ solution and 50 μl of Trolox. As for the antiradical activity, ABTS scavenging ability was expressed as EC50 (<mu> g/ml). The inhibition percentage of ABTS radical was calculated using the following formula:
As shown for DPPH scavenging, these data indicate the higher capacity of 4-(4-acetyl-5- substituted -4,5-dihydro-1,3,4-oxodiazol-2-yl)methoxy)-2H-chromen-2-ones 5 to quench ABTS+ as compared to the synthetic antioxidant Trolox.
The variation of the percentage of inhibition (PI) is almost constant starting from a value of the concentration equal to 1.34 mM. In addition, the synthesized products 5 have an antioxidant activity better than Trolox. Indeed, the antioxydant capacity seems to be attenuated when the concentration increases in the medium. This can be explained by the existence of the peroxides sites, which are susceptible for oxidizing when the concentration increases. We have just shown that the synthesized oxdiazole derivatives 5a–e have a good antioxidant activity under weak concentration, but it proves to be necessary to determine the reaction time necessary to highlight the antioxidant effect to be able to use these derivatives in pharmay.
The EC50 values exhibited by 4-(4-acetyl-5- substituted -4, 5-dihydro-1,3,4-oxodiazol-2-yl) methoxy)-2H-chromen-2-ones 5 are summarized in Table 2.
The EC50 values exhibited by 4-(4-acetyl-5- substituted -4, 5-dihydro-1, 3, 4-oxodiazol-2-yl) methoxy)-2H-chromen-2-ones 5.
Compounds 5a–e | CI50 (mmol.l−1) |
5a | 0.073 |
5b | 0.072 |
5c | 0.07 |
5d | 0.056 |
5e | 0.06 |
Trolox | 0.109 |
The 4-(4-acetyl-5- substituted -4, 5-dihydro-1,3,4-oxodiazol-2-yl)methoxy)-2H-chromen-2-ones 5 were shown to be efficient antioxidants. They showed higher free radical scavenging activity than Trolox scavenging activities.
These compounds have a remarkable capacity oxidizing which explains their susceptibility to fix free radicals DPPH and ABTS+.
5.3 Antibacterial activity
The antibacterial activity of 4-(4-acetyl-5- substituted -4, 5-dihydro-1,3,4-oxodiazol-2-yl)methoxy)-2H-chromen-2-ones 5 was assessed by the agar disk diffusion assay [36] against five human pathogenic bacteria: Gram-positive including S. aureus (CIP 7625), S. aureus and Gram-negative bacteria including E. coli (ATCC 25922), P. aeruginosa (ATCC 27853)(CIP 76110) and K. pneumonia CIP 104727. The bacterial strains were first grown on Muller Hinton medium at 37 °C for 24 h prior to seeding onto the nutrient agar. The antibacterial activity was assessed by measuring the zone of growth inhibition surrounding the discs and compared with the known antibiotic gentamycin. Standard discs of gentamycin (10 UI) served as positive antibiotic controls according to CASFM 2005 guidelines. Discs with 10 μl of pure methanol were used as negative controls. The results are given in Table 3.
Antibacterial activity spectrum of compounds 5a–e.
Indicator organism | Inhibition zone (mm) | Compounds |
Staphylococcus aureus (CIP 7625) | 34 | 5a |
27 | 5b | |
28 | 5c | |
33 | 5d | |
34 | 5e | |
24–28 | Gentamycin | |
Staphylococcus aureus* | 26 | 5a |
28 | 5b | |
27 | 5c | |
33 | 5d | |
34 | 5e | |
24 | Gentamycin | |
Escherichia coli ATCC 25922 | 30 | 5a |
27 | 5b | |
35 | 5c | |
32 | 5d | |
31 | 5e | |
22–26 | Gentamycin | |
Klebsiella pneumonia CIP 104727 | 25 | 5a |
27 | 5b | |
31 | 5c | |
30 | 5d | |
28 | 5e | |
21 | Gentamycin | |
Pseudomonas aeruginosa 27853 (CIP 76110) | 30 | 5a |
26 | 5b | |
34 | 5c | |
33 | 5d | |
32 | 5e | |
15–22 | Gentamycin |
5.3.1 Methicillin-resistant clinical isolates
An examination of the data reveals that all the compounds showed antibacterial activity ranging from 25 to 100 μg ml−1. The compounds 5a and 5e were highly active against all the five organisms employed. Compound 5c was highly active against E. coli. From the screened results, it is observed that the presence of methoxy/NO2 group at the phenyl ring increases the antibacterial activity. The activity is maximum in a compound with a methoxy group at 4th position.
6 Conclusions
A new versatile synthetic route to 4-(4-acetyl-5- substituted -4, 5-dihydro-1, 3,4-oxodiazol-2-yl)methoxy)-2H-chromen-2-ones 5 by the treatment of 4- hydroxycoumarine with different reagents is described. The method is easy; rapid and produced the title compounds 5 in good yields: the structures were verified by, UV/IR| 1D| 2D NMR. All the newly synthesized compounds were screened for their antibacterial and antioxidant activities. Among the screened samples, compounds 5a and 5b showed excellent antibacterial activity against E. coli. Compounds with 4-phenyl, 4-methoxyphenyl, 4-fluorophenyl and 4-nitrophenyl substituents in the 1,3,4-oxodiazole ring exhibited highest antioxidant activity than Trolox and 1,3,4- oxodiazole bearing 3.4.5 trimethoxysubstituent in the phenyl ring exhibited enhanced antioxidant activity from the respective series. Absorption spectral characteristics of the compounds were investigated in methanol by UV absorption and they were correlated with substituent on benzene rings.
7 Experimental
7.1 General
All reactions were magnetically stirred. Commercially available reagents were used without further purification. All chemicals were supplied from Aldrich, Merck and Fluka Co. Melting points were determined by open capillary method and were uncorrected.
All reactions were monitored by thin layer chromatography (TLC). Compounds were visualized with UV light at 254 and 365 nm. Melting points were measured on a WRX-1S instrument. Infrared (IR) spectra were recorded with a Perkin-Elmer spectrum one B spectrometer. 1H NMR spectra were recorded on a Varian-Unity spectrometer at 300 MHz using tetramethylsilane (TMS) as an internal standard. UV absorption spectra were recorded on a Lambda 20 UV spectrometer Perkin Elmer. Cyclic voltammetry (CV) was performed on a BAS 100BW electrochemical workstation. All CV measurements were carried out in tetrabutylammonium bromide (C4H12BrN) as a supporting electrolyte, purging with nitrogen prior to conduct the experiment. Platinum wire (MF-2013) was used as a working electrode, Ag/AgCl as a reference electrode, and another platinum wire (MF-1032) as a counter electrode.
7.2 Preparation of compounds 2–5
7.2.1 Preparation of ethyl (coumarin-4-oxy) acetate 2
4-hydroxycoumarin (16.2 g, 0.1 mol), anhydrous potassium carbonate (13.8 g, 0.1 mol), ethylbromoacetate (11.1 ml, 0.1 mol) are mixed in round bottomed flask. The latter is stirred at reflux in dry acetone for 10 h. The reaction mixture was filtered, while hot and the residue washed with boiling acetone. Yield (80%); mp 245 °C. IR spectrum, ν cm–1: 1732 (CO); 1525 (O–CO lactone). 1H NMR spectrum (DMSO-d6). δ ppm: 1.23(t,3H,CH3); 4.23(q,2H,CH2); 5.1(s,2H,OCH2); 5.93 (s,1H,H3); 7.36-7.82(m,4H, Harom). 13CNMR spectrum (DMSO-d6). δ ppm: 14.2(CH3); 61.4(COOCH2); 91.7(C3), 65.7(OCH2); 115.2–153.0(Carom); 161.7(C2); 164.3(C4); 167.3(CO).
7.2.2 Preparation of coumarin-4-oxyacetic hydrazide 3
Ethyl (coumarin-4-oxy) acetate (9.72 g, 0.037 mol) obtained in previous reaction and hydrazine hydrate (1.1 ml, 0.022 mol) in absolute ethanol. The reaction mixture was then stirred for 7 h at room temperature. The reaction is controlled by TLC (hexane: ethyl acetate 2:1) when the quantities of reagents are depleted, the stirring was stopped. The residue from the reaction mixture was filtered off and washed with ethanol to give a white precipitate of coumarin-4-oxyacetic hydrazide 3, which was crystallized to give colourless plates. Yield 80%; mp 265 °C. IR spectrum, ν cm–1: 1720(CO). 1715(O–CO lactone). 1H NMR spectrum (DMSO-d6). δ ppm: 5.71(s,3H,H3); 4.78 (s,2H,OCH2); 4.42(s,2H,NH2); 7.34–8.03(m,4H, Harom); 9.51(s,1H,NH). 13CNMR spectrum (DMSO-d6). δ ppm: 67.4(OCH2); 91.6 (C3); 115.3–133.2(Carom); 161.8(C2); 164.8(C4); 165.3(CO).
7.2.3 Preparation of (E)- N- arylidene coumarin-4-oxyacetic hydrazones 4
Coumarin-4-oxyacetic hydrazide 3 (2.34 g, 0.01 mol) and the substituted aromatic aldehyde (0.01 mol) were dissolved in a boiling methanol–water mixture (50:6 v/v) mL of methanol. The reaction mixture was refluxed for 5 h. Then concentrated and cooled. The solid product was filtered off, washed with the appropriate solvent.
7.2.4 (E)-N- benzylidene coumarin-4-oxyacetic hydrazone: 4a
Yield 75%. mp 244 °C. IR spectrum, ν cm–1: 1692 (CO); 1430 (CN); 3118 (NH).
1H NMR spectrum (DMSO-d6). δ ppm: 4.98(s,1H,H3); 5.47(s,2H,OCH2); 11.78(s,1H,NH); 7.38-8.03(m,8H, Harom); 7.89(s,1H, NCH). 13CNMR spectrum (DMSO-d6). δ ppm: 66.4(OCH2); 91.6 (C3); 144.6 (NC);115.6–134.2(Carom); 161.9(C2); 165.1(C4); 167.5(C1’).
7.2.5 (E)- N- (4-fluorobenzylidene) coumarin-4-oxyacetic hydrazone: 4b
Yield 72%; mp 234 °C. IR spectrum, ν cm–1: 1692 (C=O); 1430 (CN); 3118 (NH). 1H NMR spectrum (DMSO-d6). δ ppm: 4.98(s,1H,H3); 5.47(s,2H,OCH2); 11.79(s,1H,NH); 7.26–8.02(m,8H, Harom); 5.92(s,1H, NCH). 19FNMR (DMSO-d6) −110.55 ppm. 13CNMR spectrum (DMSO-d6). δ ppm: 66.4(OCH2); 91.6 (C3); 115.6-133.3(Carom); 147.5 (NC); 161.9(C2); 165.1(C4); 167.6(C1’).
7.2.6 (E)- N- (4-methoxybenzylidene) coumarin-4-oxyacetic hydrazone: 4c
Yield 73%; mp 265 °C. IR spectrum, ν cm–1: 1684 (CO); 1686 (CN); 3106 (NH).
1H NMR spectrum (DMSO-d6). δ ppm: 3.79 (s,3H, OCH3); 4.95(s,1H,H3); 5.44(s,2H,OCH2); 5.88(s,1H, NCH); 11.64(s,1H,NH); 6.98-8.24(m,8H, Harom). 13CNMR spectrum (DMSO-d6). δ ppm: 55.7(OCH3); 66.4(OCH2); 91.7 (C3); 114.6–133.3(Carom); 144.5 (NC); 162.5(C2); 165.1(C4); 167.3(C1’).
7.2.7 (E)- N- (4-nitrobenzylidene) coumarin-4-oxyacetic hydrazone: 4d
Yield75%; mp 248 °C. IR spectrum, ν cm–1: 1710 (CO); 1628 (CN); 2962 (NH). 1H NMR spectrum (DMSO-d6). δ ppm: 5.99(s,1H,H3); 5.48(s,2H,OCH2); 8.14(s,1H, NCH); 11.86(s,1H,NH); 7.38–8.27(m,8H, Harom). 13CNMR spectrum (DMSO-d6). δ ppm: 66.4(OCH2); 91.9 (C3); 115.2-133.3(Carom); 142.2 (NC); 161.9(C2); 165.0(C4); 168.0(C1’).
7.2.8 (E)- N- (3,4,5- trimethoxybenzylidene) coumarin-4-oxyacetic hydrazone: 4e
Yield75%; mp 216 °C. IR spectrum, ν cm–1: 1692 (CO); 141 0 (CN); 3118 (NH).
1H NMR spectrum (DMSO-d6). δ ppm: 3.69(s,3H, OCH3); 3.82(s,6H, OCH3); 5.50(s,2H,OCH2); 5.87(s,1H,H3); 7.02–7.94(m,6H, Harom); 7.97(s,1H, NCH); 11.80(s,1H,NH). 13CNMR spectrum (DMSO-d6). δ ppm: 56.3(OCH3); 60.5(OCH3); 66.5(OCH2); 91.6 (C3); 104.7–144.5(Carom); 144.5 (NC); 162.7(C2); 165.2(C4); 167.6(C1’).
7.2.9 Preparation of 2-[(coumarin-4-oxy) methyl]-4-acetyl-5-substitued – 1, 3,4-oxodiazoline 5
The appropriate 2-[(coumarin-4-oxy) methyl]-4-acetyl-5-substitued–1,3,4-oxadiazolines 5 (0.001 mol) were refluxed with acetic anhydride (10 ml) for 8 h, unreacted acetic anhydride was removed and the residue was solidified by trituration with ether and crystallized from the proper solvent.
7.2.10 4-(4-acetyl-5-phenyl-4,5-dihydro-1,3,4-oxodiazol-2-yl)methoxy)-2H-chromen-2-one 5a
Yield 65%. mp 218 °C. IR spectrum, ν cm–1: 1624 (CN); 1414 (NN); 1284 (COC).1H NMR spectrum (DMSO-d6). δ ppm: 1.89(s,3H, CH3); 4.91(s,2H,OCH2); 5.88(s,1H,H3); 7.35–7.97(m,9H, Harom); 7.99(s,1H,H5’). 13CNMR spectrum (DMSO-d6). δ ppm: 20.8(CH3); 65.8(OCH2); 91.6 (C3); 115.4 (C5’);116.7–133.2(Carom); 153.1(C2); 161.8(C4); 165.3(CO); 168.8(C2’).
7.2.11 4-(4-acetyl-5-(4-fluorophenyl)-4,5-dihydro-1,3,4-oxodiazol-2-yl)methoxy)-2H-chromen-2-one 5b
Yield 65%, mp 222 °C. IR spectrum, ν cm–1: 1630 (CN); 1404 (NN); 1246 (COC).1H NMR spectrum (DMSO-d6). δ ppm: 2.41(s,3H, CH3); 5.48(s,2H,OCH2); 6.0(s,1H,H3); 7.34-7.99(m,8H, Harom); 8.67(s,1H,H5’). 19F NMR (DMSO-d6) −107.13 ppm.13CNMR spectrum (DMSO-d6). δ ppm: 26.0(CH3); 69.3(OCH2); 91.9 (C3); 115.5 (C5’);116.4-133.2(Carom); 162.0(C2); 164.8(C4); 166.8(C=O); 171.2(C2’).
7.2.12 4-(4-acetyl-5-(4-methoxyphenyl)-4,5-dihydro-1,3,4-oxodiazol-2-yl)methoxy)-2H-chromen-2-one 5c
Yield 67%; mp 258 °C. IR spectrum, ν cm–1: 1624 (CN); 1388 (NN); 1246 (COC).1H NMR spectrum (DMSO-d6). δ ppm: 2.38(s,3H, CH3); 3.83(s,3H,OCH3); 5.46(s,2H,OCH2); 6.07(s,1H,H3); 7.07-7.85(m,8H, Harom); 8.53(s,1H,H5’).13CNMR spectrum (DMSO-d6). δ ppm: 25.9(CH3); 55.9(OCH3); 69.3(OCH2); 91.9 (C3); 114.9 (C5’);115.5–153.1(Carom); 162.0(C2); 164.8(C4); 168.0(CO); 171.2(C2’).
7.2.13 4-(4-acetyl-5-(4-nitrophenyl)-4,5-dihydro-1,3,4-oxodiazol-2-yl)methoxy)-2H-chromen-2-one 5d
Yield 70%. mp 216 °C. IR spectrum, ν cm–1: 1628 (CN); 1410 (NN); 1242 (COC).1H NMR spectrum (DMSO-d6). δ ppm: 2.18(s,3H, CH3); 5.40(s,2H,OCH2); 5.92(s,1H,H3); 7.18-8.29(m,8H, Harom); 7.80(s,1H,H5’). 13CNMR spectrum (DMSO-d6). δ ppm: 20.8(CH3); 66.4(OCH2); 91.9 (C3); 115.4 (C5’);115.4–153.1(Carom); 157.6(C2); 161.8(C4); 163.0(CO); 164.8(C2’).
7.2.14 (4-acetyl-5-(3,4,5-trimethoxyphenyl)-4,5-dihydro-1,3,4-oxodiazol-2-yl)methoxy)-2H-chromen-2-one 5e
Yield 72%; mp 248 °C. IR spectrum, ν cm–1: 1634 (CN); 1408 (NN); 1284 (CO–C).1H NMR spectrum (DMSO-d6). δ ppm: 2.41(s,3H, CH3); 3.75(s,3H,OCH3); 3.78(s,6H,OCH3); 4.99(s,2H,OCH2); 5.88(s,1H,H3); 7.21–7.67(m,6H, Harom); 8.43(s,1H,H5’).13CNMR spectrum (DMSO-d6). δ ppm: 20.8(CH3); 56.3(OCH3); 56.4(OCH3); 60.6(OCH2); 91.9 (C3); 116.8 (C5’);123.3–153.7(Carom); 162.0(C2); 164.8(C4); 172.4(CO); 192.2(C2’).
Acknowledgments
This work was carried out with financial aid of both the Tunisian Ministry of Higher Education and Scientific Research and Technology and the Spanish Agency of International Cooperation through projects (A/9549/07 and A/8302/07).
We are very grateful to Professor A. Romerosa, University of Almeria, Spain, for his help and for recording the 1D and 2D NMR spectra.