1 Introduction
The development of hybrid organic-inorganic frameworks due to replacing toxic metal catalysts with degradable organic compounds has emerged as one of the most potentially significant fields of investigation in modern chemistry [1]. Recently, hybrids of ionic liquids with polyoxometalates (POMs) have grown tremendously, not just as novel inorganic materials [2–4], but also as green catalysts and anti-tumor agents [5], have been increasingly known. For example, Tandon et al. [6] reported a new catalyst consisting of imidazolium and tungstate ions—tris(imidazolium) tetrakis-(diperoxotungsto)phosphate—for the oxidation of alcohols. Kumar et al. [7] reported that 1-methyl-3-butylimidazolium decatungstate catalyzed the selective oxidation of benzylic and secondary alcohols with hydrogen peroxide. Zhao et al. [8] utilized Keggin POMs [bmim]3[PW12O40] to catalyze olefin epoxidation. Very recently, Hou et al. synthesized a new POM-based ionic liquid and employed it as catalyst for the esterification of alcohols [9]. However, a limited number of these compounds have been investigated catalytically in heterocyclic synthesis [4]. Considering the number of structures discovered, it must be realized that the studying of reactions using organic cations bonded with POMs as Keggin anions as heterogeneous catalysts especially in ultrasonic-assisted organic synthesis (UAOS), is still in an immature state and being one of the underdeveloped areas research.
In recent years, solvent-free reactions in order to meet environmental demands have become one of the most important aspects in organic chemistry and great efforts have been and still are being made to find and develop new ones [10]. Moreover, the strategy of one-pot condensation reactions leading to interesting heterocyclic scaffolds is particularly useful for the creation of diverse drug-like molecules for biological screening [11]. One of the most attractive one-pot condensation reactions is the Erlenmeyer-Plöchl reaction that has been used for the synthesis of 4-arylidene-2-phenyl-5(4)-oxazolone derivatives [12] which occupy an important place in the realm of biological and pharmaceutical sciences [13–17]. Recently, almazolone as an alkaloid bearing azlactone unit, was isolated from the Senegalese alga Haraldiophyllum sp. and synthesized experimentally [18]. These systems also play a crucial role for the synthesis of hydrogel supports for in vivo cell growth [19]. Hence, synthesis of this heterocyclic nucleus is very important. Different methods have been reported for the synthesis of azlactones [20–26]. However, most of them require refluxing for hours in toxic organic solvents, the use of expensive or corrosive catalysts and a tedious work-up. As a consequence, implementation of ecofriendly and straightforward chemical methods remains actually a particularly interesting task.
As increasing environmental consciousness in chemical research and industry, the challenge for a sustainable environment calls for clean procedures. UAOS, as a green synthetic approach, is a powerful technique that is being used more and more to accelerate organic reactions [27]. UAOS can be extremely efficient and it is applicable to a broad range of practical syntheses. The notable features of the ultrasound approach are enhanced reaction rates, formation of purer products in high yields, easier manipulation and it is considered a processing aid in terms of energy conservation and waste minimization compared with traditional methods. So, this technique is more convenient, taking green chemistry concepts into account [28].
2 Results and discussion
According to the reports of Armstrong's group, germinal dicationic ionic liquids (DILs) showed high thermal stability in high temperature organic reactions [29,30]. Then, we decided to utilize this kind of cation to make the catalyst stable enough during reaction. Moreover, to our knowledge, almost all of the so-called “ionic liquids” based on POMs needed to be dissolved in organic solvents or another ionic liquid for catalytic applications. So, to expand the application of new POMs in the synthesis of heterocyclic compounds and also in continuation of our study on developing new and ecofriendly methodologies for the preparation of fine chemicals [31–34], herein we would like to report [C6(MIm)2]2W10O32. 2H2O as a new hybrid of dicationic ionic liquid-polyoxometalate which incorporates 1,6-bis(3-methylimidazolium-1-yl)hexane as cation and W10O32−4 as anion. Next, the novel POM-based DIL served as an active catalyst for room temperature synthesis of 4-arylidene-2-phenyl-5(4)-oxazolones under solvent-free and ultrasonic irradiation (US) conditions (Scheme 1).

Ultrasound-assisted one-pot synthesis of azlactones catalyzed by [C6(MIm)2]2W10O32. 2H2O.
This synthetic strategy combining the merits of solvent-free, green heterogeneous catalyst and US realized the environmentally friendly synthesis of a series of azlactones. To our knowledge, it is the first catalytic application of a hybrid of dicationic ionic liquid-polyoxometalate under ultrasonic condition for the synthesis of heterocycles.
To evaluate the catalytic activity of [C6(MIm)2]2W10O32. 2H2O, this POM-DIL was first put to use in the condensation reaction of 4-chlorobenzaldehyde (2a), hippuric acid (b) and acetic anhydride (c) at room temperature under US, as a standard model reaction. Under optimum reaction conditions, it was found that high catalytic activity for this transformation was obtained and an excellent conversion rate and satisfactory yield (92%) in the presence of only 5 mol% of the catalyst affording the desired product (Table 1).
Synthesis of 4-(4’-chlorobenzylidene)-2-phenyl-5(4)-oxazolone as a model catalyzed by ([C6(MIm)2]2W10O32. 2H2O) under different conditionsa.
Entry | Amount of catalyst (mol%) | Solventb | Method | Temperature (°C) | Yield (%)c |
1 | 3 | Solvent-free | Ultrasound | 25–35 | 60 |
2 | 4 | Solvent-free | Ultrasound | 25–35 | 75 |
3 | 5 | Solvent-free | Ultrasound | 25–35 | 92 |
4 | 6 | Solvent-free | Ultrasound | 25–35 | 91 |
5 | 0 | Solvent-free | Ultrasound | 25–35 | < 5 |
6 | 5 | CH3CN | Ultrasound | 25–35 | 20 |
7 | 5 | CHCl3 | Ultrasound | 25–35 | 25 |
8 | 5 | Toluene | Ultrasound | 25–35 | 40 |
9 | 5 | Solvent-free | Stirring | 25–35 | < 10 |
10 | 5 | Solvent-free | Stirring | 80 | 60 |
a After 18 min.
b Using 2 ml.
c Isolated Yields.
The result revealed that, in the presence of 3, 4 and 6 mol% of the catalyst, it gave lower yields of the product even after prolonged reaction times (Table 1, entries 1, 2 and 4). Moreover, the catalyst is essential and in the absence of it, the reaction progressed sluggishly (Table 1, entry 5). We have also investigated the effect of solvents and revealed that under the solvent-free condition, the excellent yield of the product was obtained (Table 1, entry 3).
To demonstrate the effect of sonication, the synthesis of 2c as a model was investigated with and without US. When the reaction was carried out in the absence of US with stirring at room temperature, the yield of 2c was very low (< 5%, Table 1, entry 9), while at the same temperature under the influence of US, it gives excellent yield of the corresponding product in a short reaction time (92%, Table 1, entry 3). Moreover, under heating condition (at 80 °C) and in the absence of US, the corresponding product was obtained only in 60% yield (Table 1, entry 10). The reason may be the phenomenon of cavitations produced by ultrasound.
To further optimize the reaction, the procedure was carried out at different output power of the ultrasound irradiation. It was found that, the yield reached a plateau at 140 W power. Using the optimized reaction conditions, we synthesized a series of 4-arylidene-2-phenyl-5(4)-oxazolones in the presence of [C6(MIm)2]2W10O32. 2H2O under US. These compounds were synthesized in excellent yields with various aromatic/heteroaromatic aldehydes containing electron donating or electron withdrawing functional groups at different positions but it did not show any remarkable differences in the yields of the products and reaction times (Table 2).
Synthesis of 4-arylidene-2-phenyl-5(4)-oxazolones catalyzed by ([C6(MIm)2]2W10O32. 2H2O) under ultrasound irradiation at room temperature.
Entry | Ar | Product | Time (min) | Yield (%)a | |
1 | C6H5 | 3a | 20 | 80 | |
2 | 4-ClC6H4 | 3b | 18 | 92 | |
3 | 2-ClC6H4 | 3c | 12 | 88 | |
4 | 2,4-Cl2C6H3 | 3d | 18 | 89 | |
5 | 4-BrC6H4 | 3e | 15 | 91 | |
6 | 2-BrC6H4 | 3f | 14 | 94 | |
7 | 3-BrC6H4 | 3g | 15 | 89 | |
8 | 4-FC6H4 | 3h | 10 | 92 | |
9 | 4-O2NC6H4 | 3i | 24 | 85 | |
10 | 3-O2NC6H4 | 3j | 25 | 87 | |
11 | 4-NCC6H4 | 3k | 24 | 85 | |
12 | 4-CH3OC6H4 | 3l | 8 | 93 | |
13 | 4-(CH3)2NC6H4 | 3m | 8 | 92 | |
14 | 4-PhCH2OC6H4 | 3n | 20 | 86 | |
15 | 3,5-(CH3)2-4-(OH)C6H2 | 3o | 10 | 90 | |
16 | 1,4-(CHO)2C6H4 | 3p | 22 | 88 | |
17 | 1-naphthyl | 3q | 8 | 91 | |
18 | 3-indolyl | 3r | 13 | 89 | |
19 | 5-CH3-2-thiophenyl | 3s | 12 | 93 |
a Yields refer to isolated pure products.
On the other hand, bis-azalctone 3p was obtained in excellent yield while using 1,4-phthalaldehyde (Scheme 2).

Synthesis of (4Z)-4-{4-[(7Z)-(5-Oxo-2-phenyloxazol-4(5H)-ylidene)methyl]benzylidene}-2-phenyloxazol-5(4H)-one.
The present method is convincingly superior to the reported methods with respect to yield, reaction time, simplicity and safety. Also, to the best of our knowledge, no report is available in the literature using a combination of polyoxometalate as catalyst and ultrasound irradiation for heterocyclic synthesis.
Generally, the reaction is experimentally excellent and proceeded under US at room temperature without using any toxic organic reagents/solvents and generates virtually no byproducts. Equally important is the high selectivity, and nearly quantitative yields of this transformation, which lead to significant structural diversity in the products. All the synthesized compounds were characterized by spectral data (IR, NMR, and MS) and compared with authentic samples.
Another advantage of this catalyst is its recycleability. Thus [C6(MIm)2]2W10O32. 2H2O can be separated by filtration and washing with hot ethanol, dried at 80 °C under reduced pressure and reused in at least five runs without any loss of its activity (Table 3).
Results obtained using recycled [C6(MIm)2]2W10O32. 2H2Oa.
Entry | Cycle no. | Time (min) | Yield (%)b |
1 | 1 | 18 | 92 |
2 | 2 | 18 | 92 |
3 | 3 | 18 | 90 |
4 | 4 | 18 | 86 |
5 | 5 | 18 | 84 |
a Based on synthesis of 4-(4’-chlorobenzylidene)-2-phenyl-5(4)-oxazolone.
b Isolated yield.
Although the detailed mechanism of the reaction is not clear, this condensation most probably proceeded via two step reactions (Scheme 3).

Plausible mechanism.
In the first step, [C6(MIm)2]2W10O32. 2H2O through activation of acetic anhydride, engages hippuric acid to produce intermediate A. In the next step, tautomerization of A in the presence of the catalyst and the following condensation reaction produces the corresponding product. It seems that US affects the stability of the intermediates and therefore, accelerates the reaction accordingly.
3 Experimental
3.1 General
All chemicals purchased from Aldrich-Sigma and Merck chemical companies and used as received. 1,6-bis(3-methylimidazolium-1-yl)hexane chloride [C6(MIm)2Cl2] was synthesised according to the literature [35]. All known organic products were identified by comparison of their physical and spectral data with those of authentic samples. Thin layer chromatography (TLC) was performed on UV-active aluminum-backed plates of silica gel (TLC Silica gel 60 F254). 1H, and 13C NMR spectra were measured on a Bruker DPX 400 MHz spectrometer in DMSO-d6 with chemical shift (δ) given in ppm relative to TMS as internal standard. Coupling constants are given in Hz. Low-resolution mass spectra (LRMS) were recorded on a Bell and Howell 21–490 spectrometer. IR spectra were taken on a FT-IR-Tensor 27 spectrometer in KBr pellets and reported in cm−1. Melting points were determined using Stuart Scientific SMP2 apparatus. Sonication was performed in a UP 400S ultrasonic processor equipped with a 3 mm wide and 140 mm long probe, which was immersed directly into the reaction mixture. The operating frequency was 24 kHz and the output power was 0–400 W through manual adjustment. Thermogravimetric analysis (TG) was carried out with a Seiko TG-DTA 6200-S under atmospheric condition. This measurement was performed with a temperature ramp of 10 °C/min between 25 and 600 °C.
3.2 Preparation and characterization of [C6(MIm)2]2W10O32. 2H2O
To a solution of Na2WO4.2H2O (5 mmol) in H2O (10 ml) was added aqueous HCl (9 ml, 3 M) and boiled until a clear yellow solution was obtained. A solution of 1,6-bis(3-methylimidazolium-1-yl)hexane chloride [C6(MIm)2Cl2] (10 mmol; dried at 80 °C) was added to this solution and the precipitate was filtered, washed with water and dried overnight at 80 °C in vacuum. Tungsten content in the catalyst was confirmed by ICP-AES analysis (Shimadz, ICP-1000 IV). The particular characteristics of the POM-based DIL catalyst during the reaction have been examined in detail. In the FT-IR spectrum of POM-based DIL, the well-known characteristic bands at 891and 796 cm−1, which resulted from the W = O and W–O–W vibrations, respectively, proved the presence of the core POM clusters. Sharp bands at 3435, 3076, 2933, 1566 and 1167 cm−1, arising from the dicationic lionic liquid, can also be seen clearly (Fig. 1).

Overlap of FT-IR Spectrums.
TGA-DTG analysis (Fig. 2) showed about 1.32% weight loss below 150 °C, suggesting that 1 mol of POM-DIL still contained about 2 mol of H2O. In addition, the TGA trace POM-DIL showed an obvious weight loss of nearly 15.3% between 300 and 425 °C, which corresponded to the proportion of the DIL.
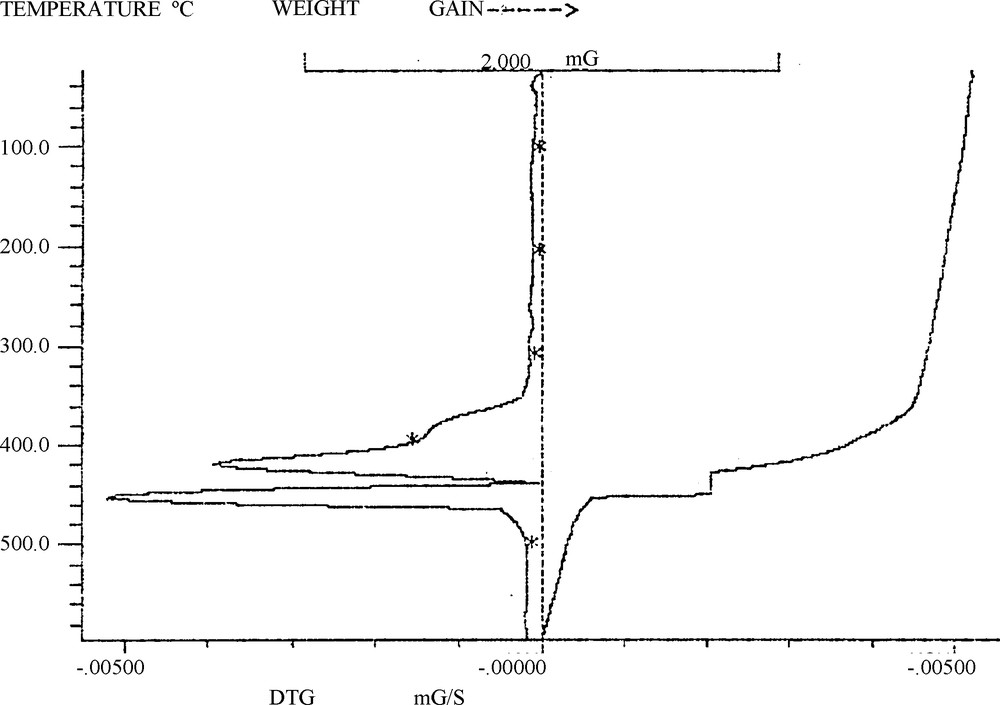
TG-DTG diagrams of [(C14H24N4)2W10O32]. 2H2O.
So we concluded that this loss resulted from the decomposition of 1,6-bis(3-methylimidazolium-1-yl)hexane ion. At around 450 °C, another obvious weight loss was detected, corresponding to the onset of the thermal decomposition of W10O32−4 (Scheme 4).

[C6(MIm)2]2W10O32. 2H2O.
3.3 General procedure for the synthesis of 4-arylidene-2-phenyl-5(4)-oxazolones
A mixture of arylaldehyde (1 mmol), hippuric acid (1.2 mmol) and acetic anhydride (5 mmol) in the presence of [(C6(MIm)2)2W10O32]. 2H2O (0.05 mmol) was exposed to US at room temperature for 5–25 min. The output power of 140 W was chosen after optimization. After completion of the reaction as indicated by TLC, ethanol (5 ml) was added to it and stirred for 10 min until a yellow solid precipitated. The mixture was allowed to stand overnight, and then it was cooled in an ice bath. An aqueous solution (20%) of NaHCO3 (10 ml) was added, the solid products and the catalyst were filtered. The solid materials were dissolved in Et2O to remove the catalyst. The solvent was evaporated and the pure products were recrystallized from ethanol (10)/water (5).
The structures of all the products were unambiguously established on the basis of their spectral analysis (FT-IR, 1H NMR, 13C NMR and mass spectra).
3.4 Spectral data for selected compounds
(4Z)-4-(4-Hydroxy-3,5-dimethoxybenzylidene)-2-phenyloxazol-5(4H)-one (3o): yellow solid, mp 217–219 °C. FTIR(KBr) υ max (cm−1): > 3000, 2922, 2848, 1791, 1761, 1654, 1595, 1201, 1130; 1HNMR (400 MHz, acetone-d6), δ (ppm): 3.91 (s, 1H), 3.95 (s, 6H), 7.28 (s, 1H), 7.64 (t, J = 8 Hz, 2H), 7.73 (t, J = 8 Hz, 1H), 7.83 (s, 2H), 8.22 (d, 2H); 13CNMR (100 MHz, acetone-d6), δ(ppm): 55.79, 109.12, 116.48, 125.57, 128.12, 129.28, 130.58, 131.77, 133.64, 136.84, 152.64, 166.82, 174.66; MS(EI): m/z (%) = 325.44, 201.25, 105.11, 77.05, 57.18.
(4Z)-4-{4-[(7Z)-(5-Oxo-2-phenyloxazol-4(5H)-ylidene)methyl]benzylidene}-2-phenyloxazol-5(4H)-one(3p): yellow orange solid, mp: 280–282 °C. FTIR(KBr) υ max (cm−1): > 3000, 2922, 2852, 1788, 1762, 1653, 1548, 1327, 1159. 1HNMR (400 MHz, CDCl3), δ (ppm): 7.59 (m, 5H), 7.68 (m, 2H), 8.25 (m, 4H), 8.32 (s, 5H). 13CNMR (100 MHz, CDCl3), δ(ppm): 125.41, 128.60, 129.06, 130.08, 132.68, 133.72, 134.64, 135.86, 164.28, 167.38. MS (EI): m/z (%) = 420.18, 287.78, 104.89, 76.88.
(4Z)-4-[(1H-Indol-3-yl)methylene]-2-phenyloxazol-5(4H)-one (3r): orange solid, mp 209–211 °C. 1HNMR (400 MHz, acetone-d6), δ (ppm): 7.36 (m, 2H), 7.56 (m, 4H), 7.72 (s, 1H), 8.05 (bs, 1H), 8.18 (d, J = 8 Hz, 2H), 8.65 (s, 1H), 8.86 (bs, 1H). 13CNMR (100 MHz, acetone-d6), δ(ppm): 112.23, 112.40, 119.62, 121.63, 123.24, 125.65, 126.45, 127.08, 127.52, 127.55, 129.13, 132.60, 134.48, 137.02, 160.03, 166.99. FTIR(KBr) υ max (cm−1): 3250, > 3000, 2922, 2852, 1726, 1639, 1230, 1132. MS (EI): m/z (%) = 288.04, 154.87, 104.88, 76.87, 56.99.
(4Z)-4-[(5-Methylthiophen-2-yl)methylene]-2-phenyloxazol-5(4H)-one (3 s): yellow solid; melting point: 145–147 °C. FTIR(KBr) υ max (cm−1): > 3000, 2920, 2852, 1788, 1645, 1548, 1325, 1151.1HNMR (400 MHz, CDCl3), δ (ppm): 2.63(s, 3H), 6.897(s, 1H), 7.44(m, 2H), 7.54(m, 2H), 7.62(m, 1H), 8.17(m, 2H). 13CNMR (100 MHz, CDCl3), δ(ppm): 16.20, 125.55, 125.89, 126.86, 127.60, 128.22, 128.91, 133.00, 135.95, 136.35, 151.58, 160.56, 167.27. MS (EI): m/z (%) = 269.12, 207.11, 105.14, 77.08, 51.14.
4 Conclusions
In conclusion, we have developed a novel, highly effective and entirely green procedure for the synthesis of 4-arylidene-2-phenyl-5(4)-oxazolones at room temperature under solvent-free and US conditions in the presence of [C6(MIm)2]2W10O32. 2H2O. To the best of our knowledge, this is the first application of hybrid of organic-inorganic catalyst in heterocyclic synthesis under sonication condition that bear dicationic ionic liquid and polyoxometalate. Moreover, other noteworthy features of this approach as follows: (i) the catalyst could be easy synthesysed, (ii) [C6(MIm)2]2W10O32 could be reused for five times after simple treatment and (iii) the corresponding azlactone can be separated by easy work-up. The mild reaction conditions, clean reaction profiles, operational and experimental simplicity, enhanced reaction rates, and with options of further transformations of the resulting 4-arylidene-2-phenyl-5(4)-oxazolones into synthetically interesting biologically active compounds, this synthetic methodology ideally suited for automated applications in organic synthesis.
Acknowledgments
The authors are grateful to the Center of Excellence of Chemistry of University of Isfahan (CECUI) and also the Research Council of the University of Isfahan for financial support of this work.