1 Introduction
Heteropoly acids (HPAs) [1,2] and their acidic salts [3,4] have been of interest as the environmentally benign reaction media in organic synthesis because of their unique properties like noncorrosive, ease of handling, recovery and regeneration. A variety of heteropoly compounds have been demonstrated as efficient catalysts in both academic and industrial processes such as hydrogenation, Mannich reaction and direct reductive amination [5–7]. Syntheses of antioxidants, vitamins and the other biologically active substances, for instance, have been reported and some are already applied in practice [8,9].
The carbon–carbon, carbon–sulfur and carbon–nitrogen bond forming processes according to Michael addition reaction are normally carried out in the presence of Lewis acids [10–14]. In these cases, several inorganic salts have been used, such as potassium phosphate [15], bismuth nitrate [16], bromodimethylsulfonium bromide [17] and bis(trifluoromethanesulfon)imide [18]. Some problems caused limitations in applying these catalysts; for example, nonrecoverability, excess amount of catalysts and unexpected side reactions like polymerization.
The HPAs-based catalysts, with the strong Brønsted and Lewis acid nature, have many advantages over the other catalysts [19–22]. For example, Keggin-type HPAs are well-known [23–28] which among them Cs2.5H0.5PW12O40 (CsPW) with a high specific surface acidity has been applied as an effective acid catalyst [29].
In this work, the CsPW heterogeneous nano-catalyst was prepared ultrasonically, for the first time. Then, aza-Michael addition reactions to α,β-unsaturated carbonyl compounds (chalcone, p-methyl chalcone and p-chloro chalcone) were performed by using the nucleophile amines (aniline, p-toluidine, p-anisidine and p-bromoaniline) in the presence of nano-catalyst CsPW (Scheme 1). The reactions were carried out under mild conditions with high yields and products characterized by spectroscopy methods. Moreover, X-ray crystallography was performed for structure determination of one of the new compounds, 3-(4-bromophenylamino)-1-phenyl-3-p-tolylpropan-1-one.
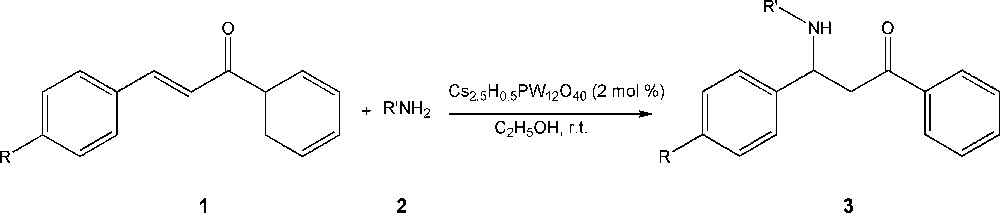
Synthesis of β-amino ketones catalyzed by nanoparticles Cs2.5H0.5PW12O40.
2 Experimental
2.1 Materials and methods
All chemicals were purchased from supplier (Aldrich, Fluka and Merck) and used without further purification. Infrared spectra were obtained by Buck 500 Scientific and Shimadzu 4300 spectrometers as KBr pellets. 1H (100, 500 MHz) and 13C NMR (125 MHz) spectra were recorded in CDCl3 on Bruker instruments. Ultraviolet spectra were recorded on an Agilent 8453 spectrophotometer at room temperature in the range of 200–400 nm by using EtOH as solvent. Elemental analyses were performed on a Thermo Finnigan Flash1112EA elemental analyzer. Diffraction data for single crystal X-ray determination were collected on an Enraf-Nonius Kappa CCD diffractometer with graphite monochromated Mo Kα (λ = 0.71073 Å) at 293 K.
2.2 Synthesis and characterization of nano-catalyst CsPW
Acidic cesium salt of 12-tungstophosphoric acid was prepared by stoichiometric ratio according to published method [29]. A 0.0125 M aqueous solution of Cs2CO3 (20 ml) was added dropwise (rate of about l ml.min−1) to a 0.01 M aqueous solution of H3PW12O40.nH2O (20 ml) at room temperature, under ultrasound irradiation for 30 min in a beaker. The resultant milky suspension was centrifuged and the solid was dried at 323 K.
A multiwave ultrasonic generator (Misonix Sonicator 4000 (S-4000), Qsonica, LLC, Newtown, CT, USA; equipped with a generator, converter, converter cable, a 1/2-inch replaceable tip horn, operating frequency at 20 kHz with a maximum power output of 600 W) was used for the ultrasound irradiation. The wave amplitude in this experiment was 40 and ultrasonic energy dissipated was set at 2.92 W, through the calorimetric method [30]. The structure and morphology of nanoparticles Cs2.5H0.5PW12O40 have been studied by scanning electron microscope (SEM), a Leo 1450 VP with accelerate voltage 35 kV and resolution 2.5 nm. The energy dispersive X-ray spectroscopic (EDX) was measured with an Oxford 7353. XRD patterns were recorded on a Bruker, D8 Advance using Ni-filtered, Cu Kα radiation (λ = 1.5406 Å). The BET surface area was determined via nitrogen adsorption at 77 K using Quantachrome Corporation. The sample was outgassed at 140 °C for 10 h before the measurement. Thermo gravimetric analysis (TGA) was performed under air with a Shimadzu TGA-50 system at a heating rate of 10 °C.min−1.
2.3 General procedure for aza-Michael addition reactions
To a magnetically stirred mixture of chalcone derivative (1.0 mmol) and CsPW (0.02 mmol) in ethanol (5 ml), amine (1.2 mmol) was added at room temperature. The reaction completion was monitored by thin layer chromatography (TLC). The nano-catalyst CsPW was collected by centrifugation. The reaction mixture was extracted with distilled water and ether (2 × 10 ml). The combined organic layer was evaporated to obtain crude product which was washed with hexane to give pure product.
2.3.1 1,3-Diphenyl-3-(phenylamino)propan-1-one (3a)
IR, ν (KBr): 3384, 1669, 1603, 1506, 1297 cm−1. 1H NMR (100 MHz, CDCl3), δ: 3.38–3.49 (m, 2H), 4.45 (brs, 1H), 5.0 (t, 1H), 6.51–6.68 (m, 3H), 7.24–7.48 (m, 10H), 7.82–7.97 (m, 2H). 13C NMR (125 MHz, CDCl3), δ: 46.3, 54.8, 113.8, 117.8, 126.3, 127.3, 128.2, 128.6, 128.8, 129.1, 133.3, 136.8, 143.0, 147.0, 198.2. UV, λmax (EtOH): 250, 298 nm. Anal. calcd for C21H19NO: C, 83.69; H, 6.35; N, 4.65. Found: C, 84.92; H, 6.36; N, 4.29.
2.3.2 3-(p-Tolylamino)-1,3-diphenylpropan-1-one (3b)
IR, ν (KBr): 3400, 1680, 1620, 1527, 1298 cm−1. 1H NMR (100 MHz, CDCl3), δ: 2.21 (s, 3H), 3.48–3.52 (m, 2H), 4.31 (brs, 1H), 5.01 (t, 1H), 6.51–6.97 (m, 2H), 7.12–7.68 (m, 10H), 7.79–8.09 (m, 2H). 13C NMR (125 MHz, CDCl3), δ: 20.3, 46.3, 55.1, 114.0, 122.2, 126.4, 127.3, 128.2, 128.6, 128.8, 129.6, 133.3, 136.8, 143.2, 144.7, 198.3. UV, λmax (EtOH): 247, 277 nm. Anal. calcd for C22H21NO: C, 83.78; H, 6.71; N, 4.44. Found: C, 83.69; H, 6.46; N, 4.46.
2.3.3 3-(4-Methoxyphenylamino)-1,3-diphenylpropan-1-one (3c)
IR, ν (KBr): 3380, 1664, 1598, 1518, 1281, 1040 cm−1. 1H NMR (100 MHz, CDCl3), δ: 3.49–3.52 (m, 2H), 3.66 (s, 3H), 4.35 (brs, 1H), 4.95 (t, 1H), 6.52–6.72 (m, 2H), 7.25–7.61 (m, 10H), 7.85–8.05 (m, 2H). 13C NMR (125 MHz, CDCl3), δ: 46.4, 55.72, 55.77, 114.7, 115.4, 126.4, 127.3, 128.2, 128.5, 128.8, 133.3, 136.8, 141.2, 143.2, 152.4, 198.3. UV, λmax (EtOH): 246, 279, 310 nm. Anal. calcd for C22H21NO2: C, 79.73; H, 6.39; N, 4.23. Found: C, 79.91; H, 6.43; N, 3.65.
2.3.4 3-(4-Bromophenylamino)-1,3-diphenylpropan-1-one (3d)
IR, ν (KBr): 3374, 1668, 1592, 1501, 1292, 1073 cm−1. 1H NMR (100 MHz, CDCl3), δ: 3.39–3.51 (m, 2H), 4.55 (brs, 1H), 4.95 (t, 1H), 6.35–6.50 (m, 2H), 7.11–7.62 (m, 10H), 7.85–8.11 (m, 2H). 13C NMR (125 MHz, CDCl3), δ: 46.1, 54.8, 109.5, 115.4, 126.2, 127.5, 128.1, 128.7, 128.9, 131.8, 133.5, 136.6, 142.4, 146.0, 198.1. UV, λmax (EtOH): 256, 315 nm. Anal. calcd for C21H18BrNO: C, 66.33; H, 4.77; N, 3.68. Found: C, 67.06; H, 4.80; N, 4.64.
2.3.5 3-(4-Chlorophenyl)-1-phenyl-3-(phenylamino)propan-1-one (3f)
IR, ν (KBr): 3390, 1664, 1600, 1503, 1292, 1085 cm−1. 1H NMR (100 MHz, CDCl3), δ: 3.40–3.52 (m, 2H), 4.51 (brs, 1H), 4.98 (t, 1H), 6.48–6.75 (m, 3H), 7.12–7.58 (m, 9H), 7.81–7.98 (m, 2H). 13C NMR (125 MHz, CDCl3), δ: 46.1, 54.2, 113.8, 118.0, 127.8, 128.1, 128.5, 128.7, 129.6, 132.9, 133.4, 136.6, 143.3, 146.7, 197.9. UV, λmax (EtOH): 250, 298 nm. Anal. calcd for C21H18ClNO: C, 75.11; H, 5.40; N, 4.17. Found: C, 76.49; H, 5.52; N, 4.58.
2.3.6 3-(p-Tolylamino)-3-(4-chlorophenyl)-1-phenylpropan-1-one (3g)
IR, ν (KBr): 3360, 1660, 1605, 1220, 1018 cm−1. 1H NMR (500 MHz, CDCl3), δ: 2.19 (s, 3H), 3.38–3.48 (m, 2H), 4.44 (brs, 1H), 4.94 (t, 1H), 6.45–6.91 (m, 2H), 7.26–7.62 (m, 9H), 7.75–8.03 (m, 2H). 13C NMR (125 MHz, CDCl3), δ: 20.3, 46.1, 54.4, 114.0, 127.8, 128.1, 128.5, 128.7, 128.9, 129.6, 132.9, 133.5, 136.6, 141.7, 144.4, 197.9. UV, λmax (EtOH): 236, 315 nm. Anal. calcd for C22H20ClNO: C, 75.53; H, 5.76; N, 4.00. Found: C, 75.10; H, 5.87; N, 3.99.
2.3.7 3-(4-Bromophenylamino)-3-(4-chlorophenyl)-1-phenylpropan-1-one (3h)
IR, ν (KBr): 3384, 1671, 1594, 1500, 1303, 1083 cm−1. 1H NMR (100 MHz, CDCl3), δ: 3.51–3.68 (m, 2H), 4.56 (brs, 1H), 5.11 (t, 1H), 6.48–6.78 (m, 2H), 7.21–7.85 (m, 9H), 8.03–8.19 (m, 2H). 13C NMR (125 MHz, CDCl3), δ: 45.9, 54.2, 109.8, 115.5, 127.7, 128.1, 128.8, 129.0, 131.8, 133.2, 133.6, 136.5, 140.9, 145.7, 197.8. UV, λmax (EtOH): 255, 300 nm. Anal. calcd for C21H17BrClNO: C, 60.82; H, 4.13; N, 3.38. Found: C, 60.57; H, 4.06; N, 2.99.
2.3.8 1-Phenyl-3-(phenylamino)-3-p-tolylpropan-1-one (3j)
IR, ν (KBr): 3381, 1666, 1602, 1510, 1292 cm−1. 1H NMR (100 MHz, CDCl3), δ: 2.47 (s, 3H), 3.39–3.59 (m, 2H), 4.67 (brs, 1H), 5.15 (t, 1H), 6.67–6.83 (m, 3H), 7.18–7.73 (m, 9H), 8.05–8.12 (m, 2H). 13C NMR (125 MHz, CDCl3), δ: 21.0, 46.3, 54.5, 113.8, 117.7, 126.3, 128.2, 128.5, 128.7, 129.1, 129.5, 133.3, 136.9, 140.0, 147.1, 198.3. UV, λmax (EtOH): 250, 298 nm. Anal. calcd for C22H21NO: C, 83.78; H, 6.71; N, 4.44. Found: C, 85.65; H, 6.86; N, 5.44.
2.3.9 3-(p-Tolylamino)-1-phenyl-3-p-tolylpropan-1-one (3k)
IR, ν (KBr): 3390, 1658, 1600, 1288 cm−1. 1H NMR (500 MHz, CDCl3), δ: 2.23 (s, 3H), 2.40 (s, 3H), 3.37–3.51 (m, 2H), 4.40 (brs, 1H), 4.95 (t, 1H), 6.48–6.91 (m, 2H), 7.12–7.82 (m, 9H), 7.91–8.03 (m, 2H). 13C NMR (125 MHz, CDCl3), δ: 20.3, 21.5, 46.4, 54.8, 114.0, 126.3, 126.9, 128.2, 128.4, 128.6, 129.7, 133.3, 136.8, 138.4, 141.0, 144.9, 198.4. UV, λmax (EtOH): 234, 324 nm. Anal. calcd for C23H23NO: C, 83.86; H, 7.04; N, 4.25. Found: C, 83.44; H, 7.61; N, 4.04.
2.3.10 3-(4-Bromophenylamino)-1-phenyl-3-p-tolylpropan-1-one (3l)
IR, ν (KBr): 3396, 1669, 1597, 1501, 1297, 1072 cm−1. 1H NMR (100 MHz, CDCl3), δ: 2.44 (s, 3H), 3.49–3.61 (m, 2H), 4.48 (brs, 1H), 4.95 (t, 1H), 6.51–6.62 (m, 2H), 7.15–7.75 (m, 9H), 8.01–8.12 (m, 2H). 13C NMR (125 MHz, CDCl3), δ: 21.0, 46.2, 54.6, 109.5, 115.4, 126.2, 128.2, 128.7, 129.6, 131.8, 133.4, 136.7, 137.1, 139.4, 146.1, 198.2. UV, λmax (EtOH): 256, 300 nm. Anal. calcd for C22H20BrNO: C, 67.01; H, 5.11; N, 3.55. Found: C, 67.69; H, 5.21; N, 4.60.
3 Result and discussion
3.1 Synthesis and characterization of nano-catalyst CsPW
The catalytic properties of HPAs are modified by using of some large cations like Cs+ as counter-cation [31,32]. This modification is done via lowering of the solubility of catalyst which overcomes the separation problems in catalysis processes. In this work, we used ultrasound irradiation in synthesis of CsPW to achieve a unique catalyst in nano-scale. The structure and morphology of nano-catalyst CsPW were studied by IR, SEM, EDX, XRD, BET and TGA that are presented in the next section.
3.1.1 Study on structure and morphology of nano-catalyst CsPW
The IR spectrum of nano-powder shows the characteristic bands of [PW12O40]3− anion at 800 cm−1 (W-Oc-W), 890 cm−1 (W-Ob-W), 985 cm−1 (W-Od) and 1080 cm−1 (P-Oa) (Supplementary data, Fig. S1). The Oa, Ob, Oc and Od atoms are the oxygen atoms which bonded to P and W, the corner-shared oxygen atoms of WO6 octahedra, the edge-shared oxygen atoms of WO6 octahedra and the terminal oxygen atoms, respectively. SEM images indicate that the particles are fairly spherical. The existence of cesium, tungsten and oxygen in CsPW was confirmed by SEM-EDX analysis (Fig. 1). The XRD pattern of nanoparticles CsPW was recorded in the range of 5 to 50° for 2θ (Fig. 2). The average particles size (D) was calculated by using the Debye-Scherrer equation [33], D = 0.89λ/(βcosθ), where λ is the wavelength of the radiation, β is the full-width at half-maximum and θ is the diffraction angle. The calculated average particles size is 22.2 nm. Specific surface area was calculated by the Brunauer-Emmett-Teller (BET) method from the data in a P/P0 range between 0 and 0.3 (Supplementary data, Fig. S2). BET results showed that the average surface area is 49.16 m2g−1. The thermal stability of nano-catalyst CsPW was studied by its TGA from 23 to 600 °C (Supplementary data, Fig. S3). The TGA weight loss of 1.624 wt.% between 23 and 193 °C is attributed to the removal of 3 moles of crystallization water per mole of CsPW.
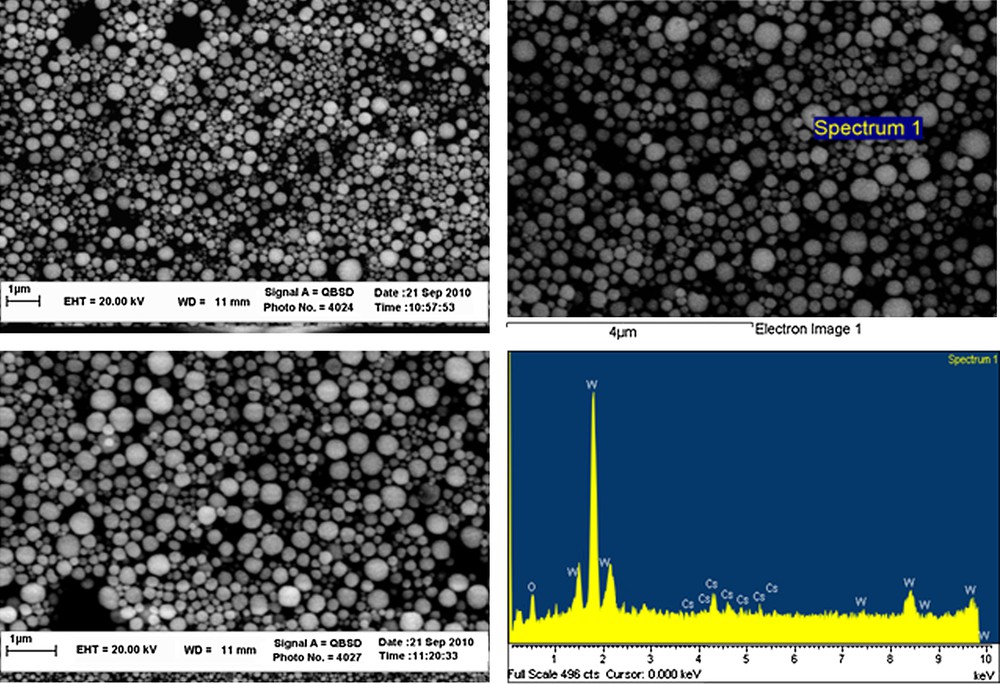
SEM-EDX images of nanoparticles Cs2.5H0.5PW12O40.

XRD pattern of nanoparticles Cs2.5H0.5PW12O40.
3.2 Aza-Michael addition reactions catalyzed by nanoparticles CsPW
We have observed that the heterogeneous nano-catalyst CsPW efficiently catalyzes the conjugate addition between a variety of Michael acceptors and nitrogen nucleophiles. The advantage of this method is the use of catalytic amounts of CsPW (2 mol %) and its ease of handling in comparison to other catalysts which should be used in more than a molar ratio with highly corrosive and toxic effects.
In fact, the nano-catalyst CsPW, having more active sites, is really more efficient than the conventional one. It means that the reaction time was longer using the conventional catalyst CsPW (2 mol %) in aza-Michael addition reactions; in some cases, the reactions were completed by heating or reflux conditions (as monitored by TLC). The reaction of chalcone and aniline in the presence of 10 mol % conventional catalyst at room temperature results in a lower yield in comparison to use of 2 mol % nano-catalyst CsPW.
The electrophilic activation of α,β-unsaturated ketones occurs by the acidic sites of nano-catalyst CsPW via the polarization of carbonyl's oxygen atom. In this case, the β-carbon atom is more susceptible to nucleophilic attack (Scheme 2). We have selected some chalcone and amine derivatives with both electron withdrawing and electron donating substituents on their aromatic rings. An excellent yield was obtained for the reaction between chalcone and aniline (Table 1, 3a). When aniline and p-bromoaniline have been used as nucleophiles, chalcone with electron withdrawing group Cl leads to better yields (Table 1, 77.5% 3f & 73.3% 3h) relative to cases involving chalcone with electron donating group CH3 (Table 1, 74.2% 3j & 44.7% 3l). However, an opposite result was obtained in the case of p-toluidine (Table 1, 3g & 3k).

Mechanistic proposal.
Nanoparticles CsPW-catalyzed aza-Michael addition of amine to chalcone derivatives.
Compound | R | R′ | Time (h) | Yielda (%) |
3a | H | C6H5 | 2 | 92.5, 85.4b |
3b | H | p-CH3C6H4 | 5 | 56.8 |
3c | H | p-OCH3C6H4 | 8 | 76.7 |
3d | H | p-BrC6H4 | 5 | 66.4 |
3e | H | p-NO2C6H4 | 24 | Trace |
3f | Cl | C6H5 | 3 | 77.5 |
3g | Cl | p-CH3C6H4 | 14 | 30.3 |
3h | Cl | p-BrC6H4 | 6 | 73.3 |
3i | Cl | p-NO2C6H4 | 24 | N. R |
3j | CH3 | C6H5 | 3 | 74.2 |
3k | CH3 | p-CH3C6H4 | 4 | 69.7 |
3l | CH3 | p-BrC6H4 | 8 | 44.7 |
a Isolated yield.
b Using 10 mol % conventional CsPW.
3.3 X-ray crystal structure determination for 3-(4-bromophenylamino)-1-phenyl-3-p-tolylpropan-1-one (3l)
Compound 3l crystallizes in the space group P21/n, from CH3OH/CHCl3, as a colorless prism. The crystal data and the details of X-ray analysis are given in Table 2. An ORTEP view of 3l with the numbering scheme is given in Fig. 3. The structure was solved by direct methods and successive Fourier difference synthesis; all H atoms were refined as riding model and all non hydrogen atoms were refined anisotropically. Atomic scattering factors were taken from the international Tables for X-ray Crystallography [34]. All the calculations and refinement were carried out with SHELX-S-97 (Sheldrick 1990), SHELX-L-97 (Sheldrick 1997) [35] and WINGX V.1.80.05 [36].
Selected crystallographic data for compound 3l.
Empirical formula | C22H20BrNO |
Formula weight (g mol−1) | 394.30 |
Temperature (K) | 293(2) |
Crystal system | Monoclinic |
Space group | P21/n |
Crystal size (mm) | 0.03 × 0.10 × 0.20 |
a (Å) | 10.62870(20) |
b (Å) | 17.38010(50) |
c (Å) | 10.91530(29) |
β (°) | 113.0391(11) |
Volume (Å3) | 1855.531(83) |
Z | 4 |
Density (calcd.) (g cm−3) | 1.411 |
μ (mm−1) | 2.224 |
F (000) | 808 |
Index ranges | −13 ≤ h ≤ 12 |
−21 ≤ k ≤ 22 | |
−12 ≤ l ≤ 14 | |
θ (°) | 7.88–54.96 |
Reflections collected | 16580 |
Independent reflections | 4186 (Rint = 0.0594) |
Refinement method | Full-matrix least-squares on F2 |
Goodness-of-fit on F2 | 1.023 |
Final R indices [I > 2σ (I)] | R1 = 0.0479, wR2 = 0.0910 |
R indices (all data) | R1 = 0.1038, wR2 = 0.1090 |
Largest diff. peak and hole (eÅ−3) | 0.400 and −0.583 |
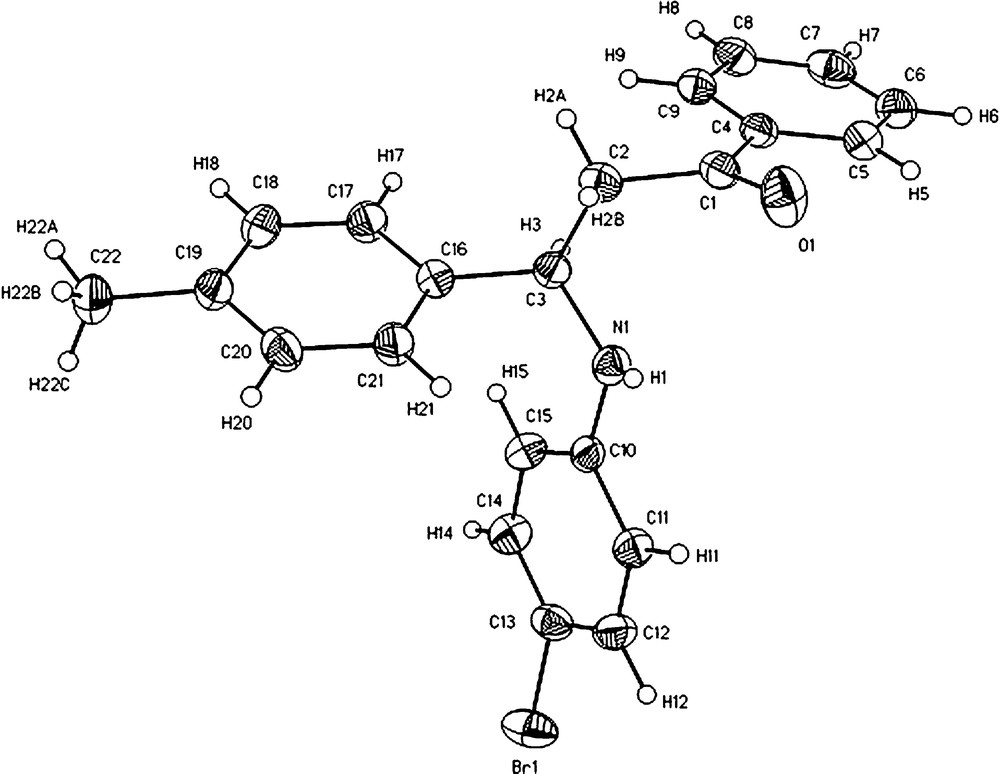
ORTEP view of a monomeric unit of compound 3l. Thermal ellipsoids are at 50% probability level, and H-atoms are shown as spheres of arbitrary radius.
In general, all bond distances for 3l are within the values characteristic according the atom involved, bond distances selected are: C(1)–C(2) 1.506(4), C(2)–C(3) 1.537(4), C(1)–C(4) 1.487(4), C(3)–C(16) 1.522(4), C(3)–N(1) 1.452(4), C(1)–O(1) 1.217(3) and C(10)–N(1) 1.393(4) Å. Therefore, torsion angle values for O(1)–C(1)–C(4)–C(5), N(1)–C(10)–C(11)–H(11) and C(3)–C(16)–C(21)–H(21) are: 12.03 (0.45)o, 2.55 (0.48)o and −2.38 (0.49)o respectively, indicating that carbonyl and NH groups and aromatic ring are almost planar. The molecule exists as a centrosymmetric dimer (Ci symmetry) via N-H…O hydrogen bond (N…O = 3.012(4) Å) in crystal lattice.
4 Conclusion
The CsPW catalyst was prepared ultrasonically, for the first time, in the nano-scale (average particles size: 22.2 nm and surface area: 49.16 m2g−1) and used as a heterogeneous catalyst for syntheses of some β-amino ketone derivatives in the mild conditions with high yields. We have examined some chalcone and amine derivatives with both electron withdrawing and electron donating substituents on aromatic ring in our work. The processes were simple and generated a diverse range of β-amino carbonyl derivatives in excellent yields which have been investigated by spectroscopic characterization and crystal structure determination for 3-(4-bromophenylamino)-1-phenyl-3-p-tolylpropan-1-one.
As a result, ease of handling, high thermal stability, separability, reusability, corrosiveness, safety, high selectivity and economy of nanoparticles CsPW have motivated its increasing use for catalysis in organic synthesis and biomedical.
Acknowledgement
The authors gratefully acknowledge the support of this work by the Ferdowsi University of Mashhad. We would also like to thank Chemical Engineering Research Lab, FUM for the TGA and BET measurements.