1 Introduction
Metal-organic frameworks (MOFs) are attracting a great deal of attention because of their potential as functional materials [1–4]. On the other hand, many parameters are involved in the formation of the MOFs, such as the coordination geometry of the metal, the structural characteristics of the ligand, the solvent of recrystallization, the nature of the counteranion, and the metal-to-ligand ratio, among others [5]. To gain more information about these subtle factors upon the resulting framework of the coordination polymers, we have undertaken a study on the effect of one parameter at a time upon the topology of the networks by carrying out a systematic examination of a series of coordination polymers using the same building block, same metal center, and same experimental conditions and varying only one or two parameters at time [6–13].
From this systematic investigation of the supramolecular architectures based on the combination of silver(I) salts and RS(CH2)nSR organic spacers, where n is the number of CH2 groups and R is the alkyl or the phenyl group, we have shown that the coordinating ability of the counteranions as well as the length and shape of the dithioether ligands have marked influence upon the topology of the resulting coordination polymers [6–13]. For example, we have evidenced that the non-coordinating counteranions lead systematically to cationic coordination polymers when the lengths of the building blocks are of intermediate size [6–13]. However, in these systems there are still some hurdles to overcome; one known challenge is structural diversity and related supramolecular isomerism. For instance, the moderately coordinating sulfonate counteranion displayed solvent induced supramolecular isomerism [6–13]. In the course of our continuous work on the construction of Ag(I)–dithioether coordination polymers, we report herein the synthesis, X-ray characterization and thermogravimetric analysis (TGA) of two new silver(I) coordination polymers based on the combination of the 1,3-bis(methylthio)propane, L, with AgNO3 and AgCF3CF2COO.
2 Experimental
2.1 General
The 1,3-bis(methylthio)propane, L, was synthesized according to a method reported in the literature [14]. The other reagents required for the synthesis were commercially available and employed without further purification. Elemental analysis was performed on an EAS 1108 apparatus from Fisons Instruments SPA. The IR spectra were recorded on a Perkin-Elmer 1750 FTIR (4000-450 cm−1) with sample prepared as KBr pellets. The 1H (300 MHz) and 19F (376.31 MHz) NMR spectra in solution were recorded on Bruker AV300 spectrometers at 25 oC. Chemical shifts are reported in parts per million and are referenced to tetramethylsilane as internal reference for 1H spectra, while the chemical shifts were referenced to C6H5CF3 (−63.9 ppm) for 19F. The weight loss experiments were carried out, under a nitrogen atmosphere, on a TG Instrument 2950 TGA HR V5.3C thermal analyser at a scan rate of 10 oC min−1.
2.2 [Ag(L)NO3]∞ (1)
A solution of AgNO3 (185 mg, 1.100 mmoL) in methanol (5 mL) was mixed with a solution of L (0.15 mL, 1.102 mmoL) in diethyl ether (5 mL) at 60 oC for 2 hours. The filtrate was recrystallized from petroleum ether. Several day later, single crystals suitable for X-ray analysis were obtained. Yield: 69% based on AgNO3. Anal. Found: C, 19.77; H, 3.47; N, 4.23. Calculated for C5H12S2AgNO3: C, 19.62; H, 3.95 N, 4.12. 1H NMR (CDCl3, 300 MHz): ( 1.972 (qd, 2H, CH3-S-CH2-CH2-CH2-S-CH3), 2.201 (s, 2H, CH3-S-CH2-CH2-CH2-S-CH3), 2.707 (t, 4H, CH3-S-CH2-CH2-CH2-S-CH3). IR (KBr, cm−1): 1384vs (N−O).
2.3 [Ag2(L)2(CF3CF2CO2)2]∞ (2)
This complex was synthesized in the same manner as 1 using AgCF3CF2CO2 (297 mg, 1.097 mmoL) and L (0.15 mL, 1.102 mmoL). Yield: 67% based on AgCF3CF2CO2. Anal. Found: C, 23.73; H, 3.40. Calculated for C16H24S4Ag2F10O4: C, 23.60; H, 2.97. 1H NMR (DMSO-d6, 300 MHz): ( 1.907 (qd, 2H, CH3-S-CH2-CH2-CH2-S-CH3), 2.127 (s, 2H, CH3-S-CH2-CH2-CH2-S-CH3), 2.621 (t, 4H, CH3-S-CH2-CH2-CH2-S-CH3).
19F NMR (DMSO-d6, 376.31 MHz): ( -84.168 (CF3), −120.137 (CF3CF2).
IR (KBr, cm−1): 1713vs (C = O); 1409m (C = O).
2.4 Structural characterization
X-ray data were measured on an SMART 6K CCD equipped with rotating anode (Cu Ka, ( = 1.54178 Å). The program SAINT was used for the data reduction processing [15]. An empirical absorption correction, which was based on the multiple measurements of equivalent reflections, was applied using the program SADABS [16]. The space group was confirmed by the XPREP [17] routine in the program SHELXTL [18]. The structures were solved by direct methods and refined by full matrix least squares and difference Fourier techniques with SHELXTL [18]. Unless otherwise noted, all non-hydrogen atoms were refined anisotropically, while the isotropic hydrogen atoms were introduced at calculated positions using a riding model. Crystal data and data collection parameters are listed in Table 1.
Crystal data and X-ray data collection parameters.
1 | 2 | |
Formula | C5H12S2AgNO3 | C16H24S4Ag2F10O4 |
Mol wt | 306.15 | 814.33 |
Cryst size (mm) | 0.14 × 0.09 × 0.05 | 0.14 × 0.09 × 0.06 |
Space group | P21/c | P21/n |
a (Å) | 8.3329 (1) | 8.0030 (2) |
b (Å) | 6.9106 (1) | 20.5242 (4) |
c (Å) | 17.0680 (3) | 15.8557 (3) |
α (deg) | 90 | 90 |
β (deg) | 94.180 (1) | 93.532 (1) |
γ (deg) | 90 | 90 |
volume (Å3) | 980.25 (3) | 2599.4 (1) |
Z | 4 | 4 |
D(calc) (g cm−3) | 2.074 | 2.081 |
F(000) | 608 | 1600 |
Limiting indices | −10 ≤ h ≤ 10 | −9 ≤ h ≤ 9 |
−7 ≤ k ≤ 8 | −24 ≤ k ≤ 24 | |
−20 ≤ h ≤ 20 | −18 ≤ h ≤ 19 | |
Temp (K) | 150 | 100 |
μ, (mm−1) | 20.273 | 15.993 |
θmax (deg) | 68.32 | 68.80 |
R1a [I > 2σ(I)] | 0.0401 | 0.0247 |
Rwb [I > 2σ(I)] | 0.0997 | 0.0627 |
R [all data] | 0.0468 | 0.0270 |
WR [all data] | 0.1314 | 0.0638 |
Sc | 1.190 | 0.979 |
Largest different peak and hole (e/Å) | 1.02/−1.49 | 0.84/−0.44 |
a .
b .
c (m is the number of reflections and n the number of parameters).
3 Results and discussion
3.1 Synthesis of the coordination polymers
The coordination polymers 1 and 2 were obtained by the reaction of 1,3-bis(methylthio)propane with AgNO3 and CF3CF2COOAg.
All coordination polymers are white powders that precipitated out from the reaction mixture. Those microcrysatlline solids are identical to the corresponding single crystal obtained by recrystallization from petroleum ether as confirmed by elemental analysis. The crystals of those complexes are relatively stable in air, although a noticeable darkening becomes evident after about seven weeks.
The stoichiometries of the compounds 1 and 2 depend on the metal-to-ligand ratio of the starting materials. Indeed, the crystals of composition [Ag(L)NO3]∞ (1) and [Ag(L)2(CF3CF2CO2)2]∞ (2) were only obtained for a ratio of 1:1 (metal:ligand) of the starting materials, which is that of the resulting coordination polymers. In other words, when varying the molarity of the ligand (1 to 40) or the metal (1 to 40), crystals could be obtained only when there is equimolarity of the two reactants. On the other hand, it seems that the recystallization solvent does not affect the topology of coordination polymers 1, 2 since the same crystals were obtained whatever the solvent of recrystallisation used (petroleum ether, heptane, hexane or pentane).
3.2 Description of the structures of the complexes
[Ag(L)NO3]∞ (1). In the coordination polymer 1 one L ligand bridge two silver atoms. In turn, these two silver atoms are as well associated by one S atom of another L ligand via μ2-S bridge. Thus, the repeat unit of 1 consists of an eight-membered Ag2LS macrocycle (Fig. 1). In this compound, one S atom of each L ligand is connected to one silver atom, while the second S atom of the same ligand is linked to two silver atoms via μ2-S bridge, thus allowing the formation of a ribbon 1D–coordination polymer parallel to the b- axis (Fig. 1).
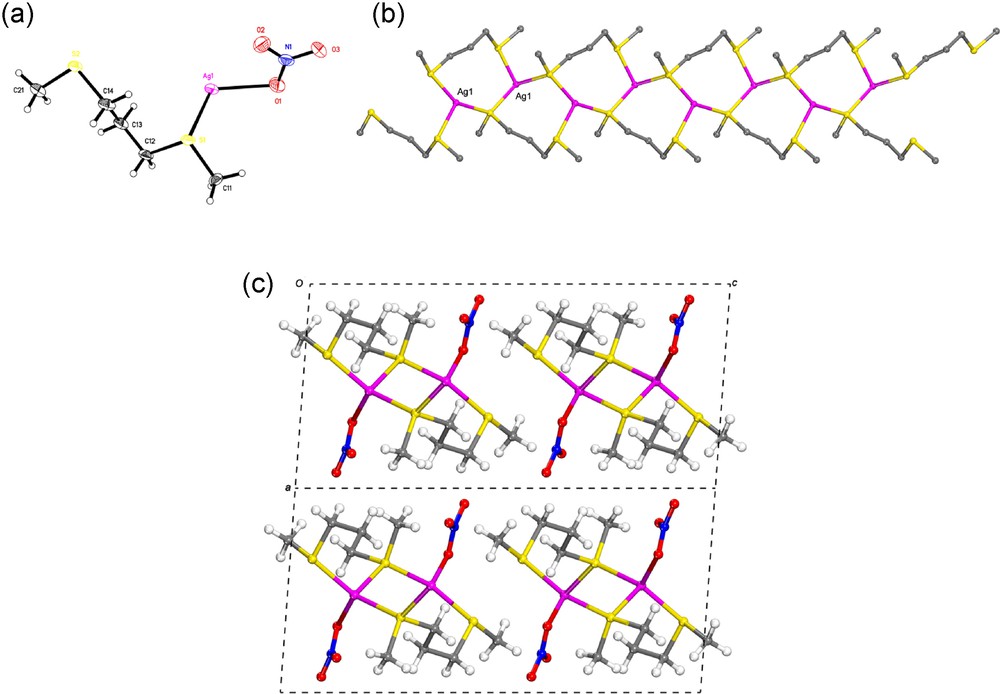
(a) View of the asymmetric unit of 1 (atomic displacement are drawn at 50% probability level). (b) The 1D-coordination polymer of 1 extending parallel to the b-axis (The hydrogen atoms are omitted for clarity). (c) Projection down the b-axis showing the packing of the 1D-chains in 1.
Each silver atom is linked to three sulfur atoms from distinct ligands (AgS: 2.494 (1), 2.564 (1), 2,660 (2) Å) and to one oxygen atom from nitrate anion. Hence the silver atom has the environment of a distorted tetrahedron (SAgO: 117.2(1), 120.0(1)°; SAgS: 105.2(1), 110.4(1)°) (Table 2).
Selected bond lengths (Å) and bond angles (°) for compounds 1 – 2.
Complex 1 | Complex 2 | ||||||
Ag(1)S(1) | 2.494(1) | S(1)AG1O(1) | 117.2(1) | Ag(1)O(3) | 2.451(2) | O(3)AG1S(1) | 97.9(1) |
Ag(1)S(2)#1 | 2.564(1) | O(1)AG1S(2)#1 | 120.0(1) | Ag(1)S(2)#1 | 2.510(1) | S(2)#1AG1S(1) | 135.3(1) |
Ag(1)S(2)#2 | 2.660(2) | S(1)AG1S(2)#2 | 110.4(1) | Ag(1)S(1) | 2.548(1) | O(3)AG1S(3) | 108.8(1) |
Ag(1)O(1) | 2.535(5) | S(2)#1AG1S(2)#2 | 105.2(1) | Ag(1)S(3) | 2.624(1) | S(2)#1AG1S(3) | 113.1(1) |
Ag(2)O(1) | 2.291(2) | O(1)AG2S(4)#2 | 155.1(1) | ||||
Ag(2)S(4)#2 | 2.447(1) | O(1)AG2S(3) | 83.4(1) | ||||
Ag(2)S(3) | 2.656(1) | S(4)#2AG2S(3) | 118.9(1) |
A recent study of the distribution of the AgS distances based on a CSD survey has been reported by Silva et al. [19]. Those authors reported that three-coordinate silver had an AgS bond distance average of 2.52 Å with a range of 2.39–3.00 Å [19]. On the basis of those results, the AgS bond distances of coordination polymer 1, 2.494 (1) – 2.660 (2) Å, may be considered to fall within the normal distance range of silver – thioether compounds [6–13,19].
Black et al. reported a 1D-coordination polymer based on the combination of the 1,3-bis(methylthio)propane, L, and AgBF4 [20]. The structure of this coordination polymer is nearly similar to that of 1. Except that in 1, the nitrate counteranion complete the coordination sphere of the silver atom in a tetrahedral fashion while in the cationic coordination polymer reported by Black et al., the non-coordinating couteranion, BF4−, counterbalance only the charge of the silver atoms [20]. The silver atoms in this cationic coordination polymer adopted a nearly trigonal planar coordination environment [20].
[Ag2(L)2(CF3CF2CO2)2]∞ (2). The combination of 1,3-bis(methylthio)propane, L, and silver(I) pentafluoropropionate lead to the 1D-coordination polymers 2. In this compound, two dithioether building blocks, L, link two adjacent silver atoms in dibridging mode giving rise to the [Ag(L)]2 dimer. In turn, adjacent dimers were connected to each other by two organic spacers L so as to form a tubular 1D-coordination polymer (Fig. 2). The repeat units of these coordination polymers are a 34-membered metallomacrocycle, Ag4(L)6 (Fig. 2b).
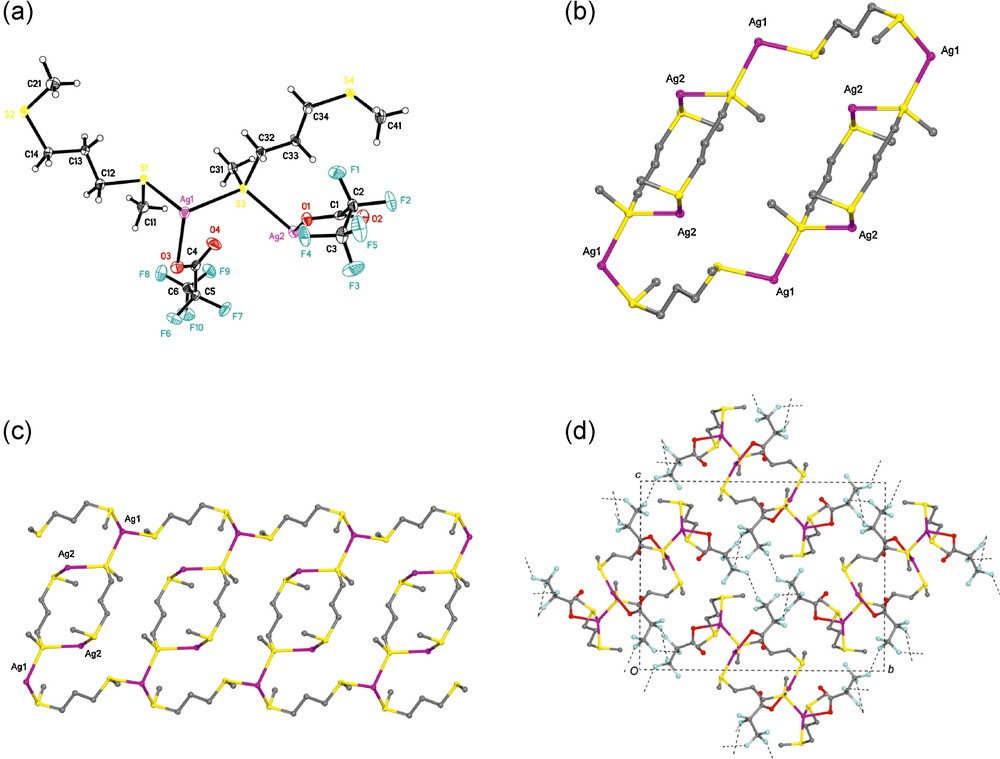
(a) View of the asymmetric unit of 2 (atomic displacement are drawn at 50% probability level). (b) The repeat unit of coordination polymer 2, Ag4(L)6. (c) 1D – coordination polymer of 2 extending parallel to the a-axis (The hydrogen atoms are omitted for clarity). (d) Packing of 2 seen along the chain axis (the tubular chains in 2 are associated through weak CF…FC interactions into 3D – network).
The coordination polymer 2 has two kinds of silver atoms: Ag(1) and Ag(2). Each Ag(1) is linked to the sulfur atoms of three distinct ligands (Ag1S: 2.510 (1), 2.548 (1), and 2.624 (1) Å), to one oxygen from pentafluoropropionate (Ag1O: 2.451 (2) Å) producing a distorted tetrahedral coordination (SAg1S: 135.3(1) and 113.1(1)°; SAg1O: 97.9(1) and 108.8(1)°).
In terms of coordination, each Ag(2) is linked to two sulfur atoms from distinct ligands (Ag2S: 2.447 (1) and 2.656 (1) Å) and to one oxygen atom from pentafluoropropionate group (Ag2O: 2.291 (2) Å) in a triangular fashion. The sum (value of 357.4°) of the angles SAg2S (155.1(1), 83.4(1), 118.9(1)°) confirms a very nearly planar environment around the Ag2 atom (Table 2).
The AgS bond lengths of 2, 2.521(1) – 2.673(1) Å, are in the normal range for silver – sulfur bond distances [6–13,19]. On the other hand, according to Chen et al. the Ag(I) tubular coordination polymers are still rare [21].
In the coordination polymer 2, each chain is surrounded by six others. They are bound to one another via weak CF…FC interactions. A 3D network may be considered when these interactions are taken into account (Fig. 2c).
Halogen–halogen contacts are characterized by the fact that the interhalogen distance is less than the sum of the van der Waals radii (rvdW) of the corresponding atoms [22,23]. Although the physical origin of short halogen–halogen contacts is still a controversy at present, it has been generally accepted that the short contact frequently exists between halogen atoms or between a carbon bonded halogen atom and an electronegative atom in molecular crystals [24]. Furthermore, the experimental charge density have demonstrated that F…F intermolecular interactions are significantly detectable and reinforce the crystal packing [25–27].
There are two preferred arrangements for these contacts: the first arrangement occurs when the RY1…Y2 angle = Y1…Y2R angle (type I in Scheme 1). The second arrangement occurs when the RY1…Y2 angle = 180° and the Y1…Y2R angle = 90° (type II, Scheme 1).
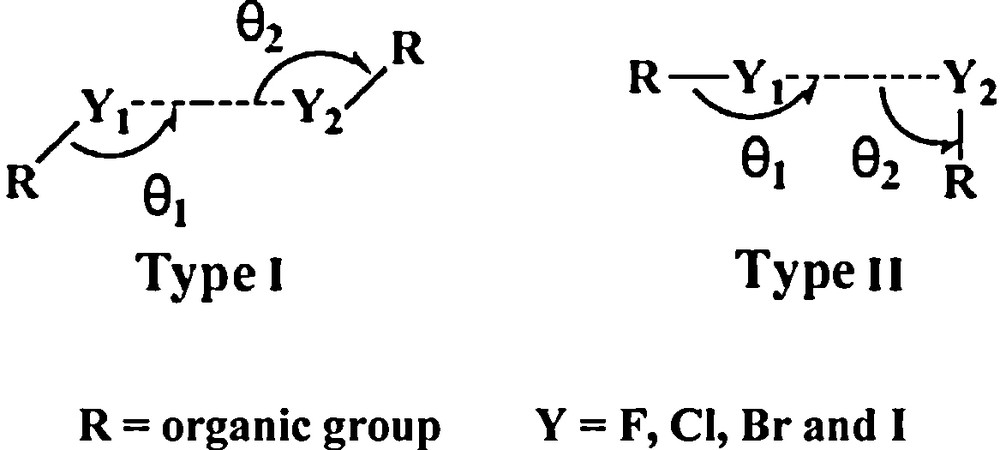
The shortest F…F distances of 2.737 and 2.938 Å were found in the coordination polymer 2. These distances are shorter than the sum of the van der Waals radii of F atoms (2.94 Å). The CF4…F10B angle of 102.8° is equal to the F4…F10BC angle; this arrangement belongs to the preferred arrangement (Type I, Scheme 1).
3.3 Infrared spectroscopy
The absorption band at 1384 cm−1 for 1 is assigned to the NO3− anion ν1(A1) vibration of [28]. On the other hand, infrared spectroscopy is usually used for the carboxylate moieties when X-ray structures are not available in order to differentiate the distinct coordination mode of the carboxylate groups, such as the bridging bidentate, chelating bidentate or unidentate modes on the basis of Δν values [Δν = νasym(CO2) – νsym(CO2)] [29,30]. The peaks observed at 1713 and 1409 cm−1 in the IR spectrum of 2 are assigned respectively to the characteristic antisymmetric and symmetric stretching bands of the carboxylate group. Furthermore, in agreement with the established crystal structure of compound 2, the 304 cm−1Δν value, points out the occurrence of the monoatomic binding mode [31].
3.4 Thermogravimetric investigation
Thermogravimetric experiments were performed in order to study the thermal stability of the coordination polymers 1 and 2. The thermogram of 1, [Ag(L)NO3]∞, and 2, [Ag2(L)2(CF3CF2CO2)2]∞, are shown in Figs. 3 and 4, respectively. The TGA curves of compounds 1 and 2 exhibits a one-step decomposition, which started at about 180 °C and completed at 320 °C. The weight loss of 1 corresponded to the departure of the L ligands and the nitrate anions (exp/theo = 63.8/64.7%) leaving behind metallic silver, and the weight loss of 2 corresponded to the departure of the L ligand and the pentafluoropropionate anions (exp/theo = 71.7/73.5) so that the final decomposition product of 2 is the metallic silver.
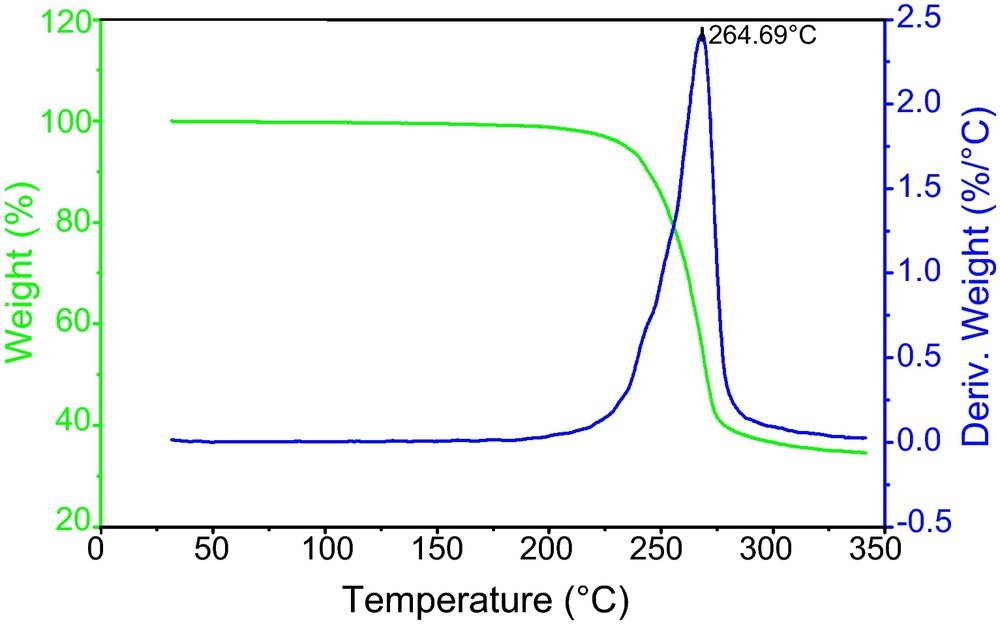
The TGA and DTGA curves for compound 1 recorded at a heating rate of 10 °C min−1.

The TGA and DTGA curves of compound 2, recorded at a heating rate of 10 °C min−1.
In a study on chemical vapour deposition of silver films [32], it was reported that the silver (I) heptafluorocarboxylate complex decomposed between 250 and 380 °C and the residue consists of metallic silver. The decomposition of compound 2, which incorporates the pentafluoropropionate anion, is actually at a lower temperature, 320 °C. Thus, the coordination polymer 2 could be used as a material for the vapour decomposition. This suggestion could be confirmed by a volatility study.
4 Conclusions
Two new coordination polymers based on the combination of 1,3-bis(methylthio)propane and Ag(I) salts have been prepared and characterized. The complexes all form a 1D polymer-like structure and the counter-anions complete only the coordination sphere of the silver ions. Furthermore, the tubular chains in 2 are, in turn, associated through weak CF…FC interactions into 3D-structures. Thermogravimetry analysis revealed that 1 and 2 have similar thermal behaviour. It was also observed that the stoichiometries for coordination compounds 1 and 2 depended on the ratio of the starting materials and did not depend on the recrystallization solvents.
Supplementary data
CCDC 641104 and 641108 contain the supplementary crystallographic data for 1 and 2. These data can be obtained free of charge via http://www.ccdc.cam.ac.uk/conts/retrieving.html, or from the Cambridge Crystallographic Data Centre, 12, Union Road, Cambridge CB2 1EZ, UK; fax: (+44) 1223-336-033; or e-mail: deposit@ccdc.cam.ac.uk.
Acknowledgement
The authors would like to thank Dr Youssouf Djibril Soubaneh for helpful discussions.