1 Introduction
Research in the area of polyoxometalates (POMs) is constantly expanding and is becoming more and more diverse [1]. Heteropolyanions are discrete, metal-oxygen compounds comprising addenda atoms (e.g. W, Mo, V), hetero atoms (e.g. P, Si, As) and oxygen (e.g. [PW12O40]3−), whereas isopolyanions do not contain hetero atoms (e.g. [V6O19]8− [1a]. Lacunary POMs are vacant derivatives of the so-called plenary structures, and hence the former can be considered as inorganic ligands, which react easily with electrophiles such as metal ions, resulting in plenary structures, or larger, more complex assemblies. The structural variety of POMs is virtually unlimited, explaining the constant discovery of novel, unexpected architectures.
Numerous structures of TiIV-containing heteropolytungstates have been so far reported in the literature [1i]. Examples are the sandwich-type dimers which are based on the tri-substituted α- or β-[Ti3XW9O40] Keggin subunit (X = Si [2,3], P [3]), the cyclic, tetrameric [{β-Ti2SiW10O39}4]24− [4], the mono-substituted Lindqvist ion [(MeO)TiW5O18]3− and its alkoxide derivatives [(RO)TiW5O18]3− [5]. Some Wells-Dawson derivatives of this class include the dimeric [(TiP2W15O55OH)2]14− and the tetrameric, tetrahedrally-shaped [{Ti3P2W15O57.5(OH)3}4]24− [6]. The Nomiya group also investigated the reactivity of different TiIV sources with [P2W15O56]12− [7]. A report on the [Ti2(OH)2As2W19O67(H2O)]8− anion containing two TiIV ions in a relatively unusual square pyramidal coordination geometry with terminal hydroxo ligands was published in 2007 [8]. Detailed catalytic and mechanistic studies on homogeneous oxidations of organic substrates with hydrogen peroxide were performed later [9]. In 2010, a monotitanium-substituted tungstoantimonate(III) and a hexatitanium-substituted tungstoarsenate(III) were reported [10].
The cyclic Ti9-containing tungstophosphate [(α-Ti3PW9O38)3(PO4)]18− and the closely related tungstosilicate [(α-Ti3SiW9O37OH)3(TiO3(OH2)3)]17− were also reported [11]. Both polyanions are composed of three (Ti3XW9O37) units linked via three Ti-O-Ti bridges and a capping group, which is either tetrahedral PO4 or octahedral TiO6. The lacunary POM precursors used were K14[P2W19O69(H2O)] for the phosphorus analogue and [{K(H2O)2}α-Si2W18O66]15− [12], which was formed in situ by acidifying a solution of K10[A-α-SiW9O34] to pH 6, for the silicon analogue.
To date, only a few TiIV-containing tungstogermanates are known. In 1993, Yamase's group reported the sandwich-type Keggin dimer [α,α-Ge2W18Ti6O77]14− (Ti6) [13a]. In 2007, the same group reported the structure of [β,β-Ge2W18Ti6O77]14− and also a catalytic study on the dehydrogenative oxidation of methanol [13b]. In 2000, Wang et al. reported the tri-substituted monomers α- and β-[(CpTi)3GeW9O37]7− [14]. In 2008, Tan et al. described the structure of the dimeric [{γ-GeTi2W10O36(OH)2}2(μ-O)2]8− [15], by using the [γ-GeW10O36]8− precursor, first reported by our group [16]. In 2008, Ren et al. published the structures of two trimeric, tri-Ti-containing Keggin ions based on tetrahedral linkers, [K⊂(Ge(OH)O3)(Ti3GeW9O38H2)3]14− and [K⊂(SO4)(Ti3GeW9O38H3)3]10−. The syntheses of these two polyanions were conducted at a rather high temperature (98 °C), also using [γ-GeW10O36]8− as POM precursor [17].
Here we report on the reactivity of different TiIV precursors with the trilacunary Keggin ion [A-α-GeW9O34]10− in aqueous, acidic medium. In particular, we made some surprising discoveries when trying to reproduce [(Ge(OH)O3)(Ti3GeW9O38H2)3]14− following the published procedure of Ren et al. [17].
2 Experimental
2.1 Materials and instrumentation
The chemicals were used as purchased without further purification. Na10[α-GeW9O34]·23H2O was prepared according to the literature [18]. Infrared spectra were recorded on KBr pellets using a Nicolet Avatar spectrophotometer. All NMR spectra were recorded on a 400 MHz JEOL ECX instrument at room temperature using H2O/D2O as solvent. An SDT Q 600 TA-Instrument and Universal Analysis 2000 TA-Instruments data processing system were used for the TGA measurements which were run under N2 with a temperature ramp of 10 °C per minute between 25 and 900 °C. Elemental analyses were performed by Service Central D’Analyse, CNRS, Solaize, France.
2.2 Synthesis of Rb13K2Na2[(α-Ti3GeW9O37OH)3(TiO3(OH2)3)]·40H2O (Rb-Ti10)
In the first step, K8[Ti4O4(C2O4)8] was prepared by crystallization from a saturated, aqueous solution of K2TiO(C2O4)2. In the second step, 0.50 g (0.17 mmol) of Na10[A-α-GeW9O34] and 0.25 g (0.19 mmol) of K8[Ti4O4(C2O4)8] were added to a mixture of 10 mL 0.5 M rubidium acetate buffer (pH 4.8) and 15 mL of 1 M lithium acetate buffer (pH 4.8). The solution was heated at 60 °C for 1 h, allowed to cool to room temperature, and then left standing in an open vial for slow evaporation. After a few days rhombohedral crystals of Rb13K2Na2[(TiO3(H2O)3)(Ti3GeW9O37OH)3]·40H2O (Rb-Ti10) appeared (yield 0.16 g, 30%). IR Data. 457 (w), 490 (w), 525 (w), 715 (s), 810 (w), 885 (m), 949 (m), 1405 (w) and 1624 (s) cm−1. Elemental Analysis Data. Calculated (found). Na 0.48 (0.56), K 0.82 (1.33), Rb 11.64 (13.71), Ge 2.28 (2.29), Ti 5.01 (4.74), W 52.0 (50.34). 183W-NMR Data. Solid Rb-Ti10 was dissolved in 1 M lithium acetate buffer (pH 4.8) solution. Adding sodium exchange resin (Chelex 100, sodium form) to the solution enhanced the solubility of Rb-Ti10 allowing for an increased polyanion concentration, which is desirable for 183W-NMR measurements. The room temperature 183W-NMR spectrum showed five singlets at −87.1, −93.7, −101.3, −131.5 and −134.1 ppm with a 2:2:2:2:1 intensity ratio, the peak of smallest intensity being the most upfield.
2.3 Single-crystal X-ray diffraction
A colorless plate of Rb-Ti10 with dimensions 0.15 × 0.14 × 0.05 mm3 was mounted on a Hampton cryo-loop for indexing and intensity data collection at 173 K on a Bruker D8 APEX II CCD using Mo-Kα radiation (λ = 0.71073 Å). Of the 107,781 reflections collected (2θmax = 49.42, 99.6% complete), 4902 were unique (Rint = 0.1015) and 4468 reflections were considered observed (I > 2σ(I)). Lorentz and polarization corrections were applied, and an absorption correction was performed using the SADABS program (G.M. Sheldrick, Siemens Analytical X-ray Instrument Division: Madison, WI, 1995) [19]. Direct methods were used to locate the tungsten atoms (SHELXS-97) [20]. Then the remaining atoms were found from successive Fourier maps (SHELXL-97) [20]. The final cycle of refinement, including the atomic coordinates and anisotropic thermal parameters (Ti, Ge, Rb and W atoms) converged at R = 0.0389 (I > 2σ(I)) and Rw = 0.1428 (all data). In the final difference map, the deepest hole was −1.779 eÅ−3 and the highest peak 3.051 eÅ−3. The crystallographic data for Rb-Ti10 are summarized in Table 1.
Crystallographic data for Rb13K2Na2[(α-Ti3GeW9O37OH)3(TiO3(OH2)3)]·40H2O (Rb-Ti10).
Rb-Ti10 | |
Formula | Rb13 K2 Na2 Ge3 Ti10 W27 H69 O150 |
Formula weight (g/mol) | 9365.6 |
Crystal system | Rhombohedral |
Space group (Nr.) | R3 m (160) |
a (Å) | 29.6597 (5) |
b (Å) | 29.6597(5) |
c (Å) | 16.0158 (4) |
α (deg) | 90 |
β (deg) | 90 |
γ (deg) | 120 |
Volume (Å3) | 12201.5 (4) |
Z | 3 |
Dcalc (g/cm3) | 3.824 |
Abs. Coeff. μ (mm−1) | 24.034 |
Total reflections | 107781 |
Unique reflections | 4902 |
Final R1a | 0.0375 |
wR2a | 0.1164 |
a ;
2.4 Synthesis of K9H5[α,α-Ge2W18Ti6O77]·22H2O (K-Ti6)
0.60 g (0.21 mmol) of solid Na10[A-α-GeW9O34]·23H2O and 120 μL (0.80 mmol) of TiOCl2·xHCl (d = 1.58 g/mL) solution were added to 20 mL of 1 M potassium acetate buffer. The mixture was heated to 80 °C for 1 h, cooled down to room temperature, and then mixed with 1 mL of 1 M KCl solution. Slow evaporation in a small, open vial resulted in thin, plate-like crystals of K9H5[α,α-Ge2W18Ti6O77]·22H2O (K-Ti6) after a few days (yield 0.28 g, 40%). IR Data. 456 (w), 520 (w), 630 (sh), 759 (m), 808 (w), 873 (m), 946 (m) and 1615 (s) cm−1. 183W-NMR Data. The 183W-NMR spectrum showed the expected two singlets at −98 and −134 ppm, with relative intensities of 1:2. We also measured a single-crystal XRD data set on K-Ti6, which revealed a polyanion isostructural with that reported by Yamase et al. [12a].
3 Results and discussion
Here we report on the synthesis and structural characterization of the novel polyanion [(α-Ti3GeW9O37OH)3(TiO3(OH2)3)]17− (Ti10). This polyanion is composed of three (Ti3GeW9O37) units linked via three Ti-O-Ti bridges and an octahedral TiO6 capping group (Figs. 1 and 2), and was synthesized by reaction of K8[Ti4O4(C2O4)8] (prepared by crystallization from a saturated, aqueous solution of K2TiO(C2O4)2) with Na10[A-α-GeW9O34] in a mixed rubidium/lithium acetate buffer (pH 4.8) upon heating at 60 °C for 1 h, and isolated as the hydrated, mixed rubidium/potassium/sodium salt Rb13K2Na2[(α-Ti3GeW9O37OH)3(TiO3(OH2)3)]·40H2O (Rb-Ti10).

Top view of [(TiO3(H2O)3)(Ti3GeW9O37OH)3]17− (Ti10) highlighting the 3-fold symmetry of this polyanion. Color code of the balls: teal (W), yellow (Ti), red (O), violet (Ge), grey (Rb).

Side view of [(TiO3(H2O)3)(Ti3GeW9O37OH)3]17− (Ti10) highlighting the capping TiO6 group. Color code: teal (WO6 octahedra), yellow (Ti), red (O), violet (Ge).
Polyanion Ti10 is isostructural with [(α-Ti3SiW9O37OH)3(TiO3(OH2)3)]17− and hence represents the Ge-analogue of the latter [11]. The title ion consists of three (A-α-GeW9O34) Keggin units, all substituted by three corner-shared TiO6 octahedra, which are interlinked via three Ti-O-Ti bridges. On the other side of the polyanion, an octahedral TiO6 cap holds the entire structure together via almost linear (average of 179.3°) Ti-O-Ti bonds (Fig. 2). Thereby, three O atoms of the TiO6 moiety act as bridges to the Keggin units, with Ti–O distances of 1.81(2) Å. Polyanion Ti10 has nominal C3v symmetry with the 3-fold rotational axis passing through the capping Ti atom (Fig. 1). Bond valence sum (BVS) [21] calculations indicated that the three terminal, facial ligands of the octahedral TiO6 cap, pointing away from the center of the molecule, are actually water molecules (Ti-Oaqua = 2.11(4) Å). In the solid state, Ti10 is stabilized by four Rb+ counter cations, which are closely associated with the polyanion (Fig. 1). One of the Rb+ ions is located on the three-fold axis of Ti10, opposite the TiO6 capping group. The other three Rb+ ions occupy the spaces in-between the neighboring Keggin units. The Rb–O bond lengths are in the range 2.898(14) – 3.610(15) Å.
We have discovered a simple synthetic procedure leading to the pure, trimeric polyanion Ti10. The isostructural silicon analogue [(α-Ti3SiW9O37OH)3(TiO3(OH2)3)]17− and the closely related phosphorus analogue [(α-Ti3PW9O38)3(PO4)]18− (with a tetrahedral PO4 capping group) have also been reported by our group [11]. In the same year, Ren et al. reported [(Ge(OH)O3)(Ti3GeW9O38H2)3]14− and [(SO4)(Ti3GeW9O38H3)3]10− [17], which are isostructural with our [(α-Ti3PW9O38)3(PO4)]18−, and are closely related to Ti10. The syntheses were performed at 98 °C and [γ-GeW10O36]8− was used as POM precursor.
However, in our hands this synthetic procedure reported by Ren et al. did not lead to the claimed product [(Ge(OH)O3)(Ti3GeW9O38H2)3]14−, but rather to a mixture of salts of the dimeric [α,α-Ge2W18Ti6O77]14− and the plenary Keggin ion [GeW12O40]4−. Based on our observations, several reaction parameters had to be optimized, in order to obtain Ti10 in a pure form. Firstly, the abundant presence of rubidium ions seems to be indispensible for the selective crystallization of the clean target product; secondly, heating to temperatures above 60 °C can adversely affect the purity, and thirdly, the combination of lithium acetate and rubidium acetate buffers presents a more suitable crystallization medium than rubidium acetate alone.
We also investigated the solution stability of Ti10 by 138W-NMR at room temperature by dissolving Rb-Ti10 in 1 M lithium acetate buffer (pH 4.8) with the aid of a sodium-exchange resin. We observed five singlets at −87.1, −93.7, −101.3, −131.5, and −134.1 ppm with a 2:2:2:2:1 intensity ratio (Fig. 3). This correlates with the polyanion structure in the solid state, and hence demonstrates that Ti10 is indeed stable in solution. The same number of peaks and relative intensities were observed for 138W-NMR of the Si-analogue, but the signals are shifted upfield by about 20–30 ppm (−116.7, −124.7, −133.8, −150.7, and −153.4 ppm) [11].
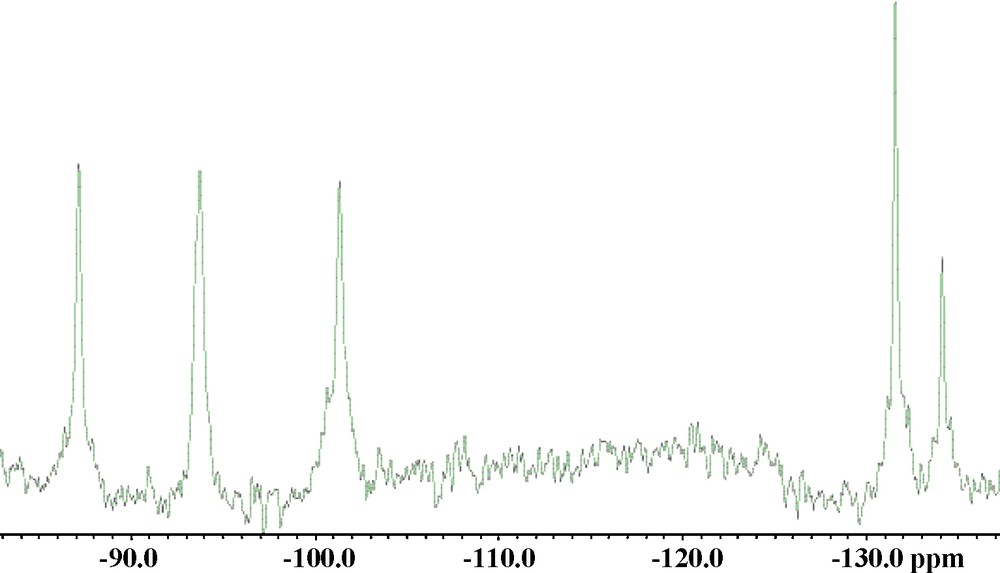
Room temperature 183W-NMR spectrum of solid Rb-Ti10 dissolved in 1 M lithium acetate buffer (pH 4.8).
Interestingly, after Rb-Ti10 had been completely harvested, the same crystallization solution yielded crystals of K-Ti6; the identity and purity of the latter were confirmed by various analytic techniques. The 138W-NMR spectrum of K-Ti6 redissolved in water showed the expected two singlets at −98 and −134 ppm, respectively, with relative intensities of 1:2. We also collected a single-crystal XRD data set on K-Ti6, which revealed a polyanion isostructural with that reported by Yamase et al. [13a]. Therefore, our XRD and NMR data fully confirmed the results of these authors. The synthesis procedure of Yamase et al. involves mixing the precursors Na10[α-GeW9O34] and TiCl4 in water, refluxing for 30 min, adding KCl and then recrystallizing twice in order to obtain the final product. We were able to obtain the same polyanion Ti6 following a slightly different procedure, where TiOCl2 and Na10[α-GeW9O34] were mixed in a 1 M potassium acetate buffer pH 4.8, heated for 1 h at 80 °C, and then left for slow evaporation of the solvent.
In summary, we have developed a synthetic procedure which allows isolating the title polyanion Ti10 in a pure form. Interestingly, this result has evolved from original attempts trying to reproduce [(Ge(OH)O3)(Ti3GeW9O38H2)3]14− by following the published procedure of Ren et al. [17], which did not work in our hands, in spite of numerous attempts. At the same time, we also discovered an alternative procedure for the preparation of Ti6, which is at least as simple as the one reported by Yamase et al. [13a]. Our work demonstrates that several synthetic parameters such as Ti-precursor, solvent, temperature, and counter cations play a major role in determining the identity and purity of various Ti-containing tungstogermanates. The dimeric Ti6 appears to be favored in the presence of potassium ions, either alone or together with one or more types of alkali ions. This also explains the formation of Ti6 following Ren's procedure, where K+ was the only available counter cation in solution. On the other hand, the trimeric Ti10 is favored in the presence of Rb+ ions.
4 Conclusions
We have investigated the reactivity of TiIV ions with the trilacunary 9-tungstogermanate [A-α-GeW9O34]10− in some detail. Reaction of different Ti-sources with [A-α-GeW9O34]10− led to various mixtures of different polyanions. A subtle tuning of the reaction conditions allowed us finally to isolate the pure trimeric polyanion [(α-Ti3GeW9O37OH)3(TiO3(OH2)3)]17− (Ti10) in the form of a crystalline, mixed rubidium/potassium/sodium salt. Several reaction parameters needed to be carefully controlled for the successful synthesis and selective crystallization of Ti10, such as using K8[Ti4O4(C2O4)8] as precursor, the right mixture of rubidium and lithium acetate buffers, a temperature of 60 °C and very importantly, the abundant presence of rubidium ions. During our work, we also discovered that the synthetic procedure reported by Ren et al. [17] did not lead in our hands to the claimed product [(Ge(OH)O3)(Ti3GeW9O38H2)3]14−, but rather to a mixture of salts of the dimeric [α,α-Ge2W18Ti6O77]14− and the plenary Keggin ion [GeW12O40]4−. Our studies reemphasize that the aqueous solution chemistry of TiIV sources with lacunary heteropolytungstates is rather complex and great analytical care is required to isolate pure compounds. In particular, solution studies (e.g. multinuclear NMR) are essential to shed light on polyanion stability, structure, and purity.
5 Supplementary material
Details on the crystal structure investigation of Rb-Ti10 may be obtained from the Fachinformationszentrum Karlsruhe, 76344 Eggenstein-Leopoldshafen, Germany (fax: +49 7247 808 666; e-mail: crysdata@fiz-karlsruhe.de), on quoting the depository number CSD 423125.
Acknowledgements
U.K. thanks the German Science Foundation (DFG-KO-2288/9–1) and Jacobs University for research support. Figs 1 and 2 were generated using Diamond, version 3.2 (copyright Crystal Impact GbR).