1 Introduction
The search for molecule-based materials exhibiting multiphysical properties is one of the current challenges in molecular materials science [1,2]. Great interest is currently devoted to obtain organic/inorganic hybrid materials in which it is expected that there is long-range magnetic coupling between the localised spins on d-orbitals of the paramagnetic transition metal ions of the inorganic part through the mobile electrons of the conducting networks (π-electrons) formed by the organic ligands [1,3,4]. To achieve strong interactions between the paramagnetic metal ions and, for example, the redox-active tetrathiafulvalene (TTF) ligands, the strategy which involves the covalent attachment of metal ion binding groups to π-conjugated TTF derivatives and their linkage into supramolecular systems, has been investigated. With the appropriate choice of metal ions, the resulting systems would be expected to show multiple physical properties, such as electrical conductivity or superconductivity and magnetic effects, optical and magnetic properties or spin-crossover, in a synergistic way. Within this context, the binding of 4′,5′-bis-(propylthio)tetrathiafulvenyl[i]dipyrido[3,2-a:2′,3′-c]phenazine (TTF-dppz) and its analogue 4′,5′-bis(propylthio)tetrathiafulvenyl[i]dipyrido[2,3-a:3′,2′-c]phenazine (TTF-ppb) to a variety of transition metal ions and their excited-state charge separation characteristics have been investigated in our laboratory [5]. Herein, we now describe the synthesis of four new lanthanide complexes (1-4) with the TTF-dppz ligand (Scheme 1), together with their electrochemical, optical and magnetic properties as well as the crystal structure of the corresponding Gd(III) complex, namely [Gd(NO3)3(TTF-dppz)2] (3).

A synthetic route for the preparation of complexes 1-4.
Compared to the numerous studies on coordination compounds composed of d-metal ions and TTF-type ligands, the investigations on corresponding lanthanide complexes are quite rare [6]. In fact, lanthanide compounds are appealing, e.g. by virtue of their specific luminescence, f-electron configurations, nonlinear optics and magnetic characteristics [7,8]. On the one hand, it has been demonstrated that TTF-based ligands act as efficient charge transfer antennae for the sensitization of Yb(III) NIR luminescence [6a,b]. On the other hand, Ouahab et al. reported that a dinuclear Dy(III) complex bearing two electroactive functionalized TTFs behaves as a single-molecule magnet with an energy barrier, in zero magnetic field, as high as 87 K [6f]. During this work, we have explored the coordination ability of TTF-dppz to some lanthanide(III) ions in order to establish a new combination of Ln(III) ions with π-extended redox-active chromophoric ligands.
2 Results and discussion
2.1 Syntheses
The molecule TTF-dppz was synthesized according to the literature procedure [5a] and [Gd(III)(NO3)3]·6H2O was prepared by evaporation of an aqueous solution of gadolinium oxide in the presence of nitric acid. [Nd(III)(NO3)3]·6H2O, [Eu(III)(NO3)3]·6H2O and [Tb(III)(NO3)3]·5H2O are commercial products. The lanthanide(III) complexes [Ln(NO3)3(TTF-dppz)2] with Ln(III) = Nd (1), Eu (2), Gd (3), Tb (4) were obtained following a general synthetic procedure as shown in Scheme 1. To a solution of TTF-dppz ligand (2 equiv.) in CH2Cl2 was added a solution of [Ln(III)(NO3)3]·xH2O (1 equiv.) in ethanol. The color of the solution changed from red purple to blue, immediately followed by the precipitation of the heteroleptic Ln(III) complexes. All complexes are stable in the solid state and can be isolated as deep-blue powders in good yields after washing with a large amount of ethanol and drying in vacuum. Single crystals of 3 were grown by slow diffusion of hexane to its CH2Cl2 solution in the dark.
2.2 Crystal structure of complex 3
Complex 3 crystallizes in the orthorhombic polar space group Fdd2 as an inversion twin with a component ratio of 0.625(10)/0.375(10). It is composed of a Gd(III) ion coordinated with three nitrate ligands and two TTF-dppz molecules. There are four complexes per asymmetric unit and thus 64 complexes in the huge unit cell. One bis-propylthio-TTF fragment is disordered over two conformations with a site population ratio of 0.436/0.564. One propylthio group has 0.5/0.5 disorder over two sites. Fig. 1 shows one of the four crystallographically independent complexes. The Gd atom is coordinated by four N atoms of two TTF-dppz ligands and six O atoms of three nitrate ligands to form a 10-fold coordination which may be seen as a distorted bicapped square antiprism (top square: O1/O5/N2/N31; bottom square: O2/O7/N32/N1; capped by O4 and O8). One nitrate ligand bridges from the top cap to the upper square (O4/O5), and a second one bridges from the lower cap to the lower square (O8/O7); the third nitrate ligand as well as the two TTF-dppz molecules connect the upper with the lower square (O1/O2, N2/N1 and N31/N32). The ranges of bond distances for the four complexes are 2.488(14)–2.628(12) Å, 2.562(14)–2.614(13) Å, 2.537(13)–2.598(14) Å, 2.571(14)–2.617(14) Å for Gd-N and 2.418(11)–2.583(10) Å, 2.476(11)–2.526(11) Å, 2.459(12)–2.504(13) Å, 2.428(12)–2.575(15) Å for Gd-O, respectively, indicating that their coordination geometries are similar but have slightly different distortions. The mean Gd-N bond distances of the four complexes of 2.54(6) Å, 2.58(3) Å, 2.57(2) Å and 2.59(6) Å are slightly longer than the mean Gd-O bond distances of 2.50(7) Å, 2.499(19) Å, 2.473(13) Å and 2.48(5) Å. They match the corresponding mean distances of the closely related [Gd(NO3)3(phen)2] [9] of 2.54(3) Å and 2.51(3) Å. The TTF-dppz ligands are stacked head-to-tail along the [01–1] and [0–1–1] directions with their molecular planes almost parallelly aligned (Fig. 2). Ligands A and B have nearly perfect antiparallel orientations. The other ligand pairs, C and D, are aligned in a crossed orientation. The shortest contacts between atoms in neighbouring ligand planes are S61-C131 3.379 Å, C76-C138 3.351 Å, C4-C76 3.373 Å, C227-C17 3.358 Å and C138-C222 3.333 Å and thus indicate a pronounced π-π stacking effect. The only S-S contact which is shorter than the sum of the v.d.W. radii is S32-S186 with 3.563 Å.

ORTEP representation [12] of the molecular structure of complex 3; view of one of the four independent complexes (30% probability ellipsoids; H-atoms given arbitrary displacement parameters for clarity). The atoms at the vertices of the bicapped square antiprism are labeled.

Crystal packing pattern of 3; stacking of the TTF-dppz ligands along [01–1] (interatomic distances given in Å, H-atoms are omitted for clarity).
2.3 Electrochemical studies
The electrochemical properties of the lanthanide(III) complexes 1-4 in CH2Cl2 were investigated by cyclic voltammetry as illustrated in Fig. 3. Their cyclic voltammetric data are collected in Table 1 together with those of the TTF-dppz ligand for comparison. In each case, two reversible single-electron oxidation waves around 0.83 and 1.17 V are observed, both typical of the TTF unit (see: E1/2ox1 and E1/2ox2 in Table 1). In the negative potential direction, one (quasi)-reversible reduction is observed corresponding to the reduction of the dppz moiety of the ligand. Upon coordination, both oxidation and reduction potentials are positively shifted by 100 mV due to the electron-withdrawing effect of the lanthanide(III) ions.

Cyclic voltammograms of 1-4 in CH2Cl2.
Cyclic voltammetric data.a
E/V | E1/2red1 | E1/2ox1 | E1/2ox2 |
TTF-dppz | −1.17 | 0.73 | 1.08 |
1 | −1.08a | 0.81 | 1.16 |
2 | −1.06 | 0.83 | 1.16 |
3 | −1.08 | 0.84 | 1.17 |
4 | −1.08 | 0.84 | 1.17 |
a Conditions: all oxidation potentials were determined in CH2Cl2 under Ar at r.t. containing 0.1 M Bu4N(PF6), with Ag/AgCl as reference electrode, Pt disk as working electrode and glassy carbon as counter electrode, scan rate 100 mV s−1.
2.4 Optical properties
The electronic absorption spectra of the lanthanide(III) complexes 1-4 were determined in CH2Cl2 solution, as shown in Fig. 4. The key feature of the absorption spectra is the intense broad absorption band which appears for all four compounds around 18,000 cm−1 (555 nm). This optical absorption band can be readily attributed to a spin-allowed electronic π-π* intraligand charge-transfer transition (1ILCT) which is based on the TTF subunit as an electron donor and the dppz subunit as an electron acceptor; this electronic transition is well documented in case of the optical absorption spectrum of the free TTF-dppz ligand [5a]. Compared to the free ligand, the 1ILCT bands for the complexes 1-4 are red-shifted by about 700 cm−1, a fact which is also well documented for other metal complexes with the TTF-dppz ligand [5b]. At energies higher than 25,000 cm−1 (400 nm), the optical absorptions correspond mainly to electronic π-π* transitions of the dppz units.
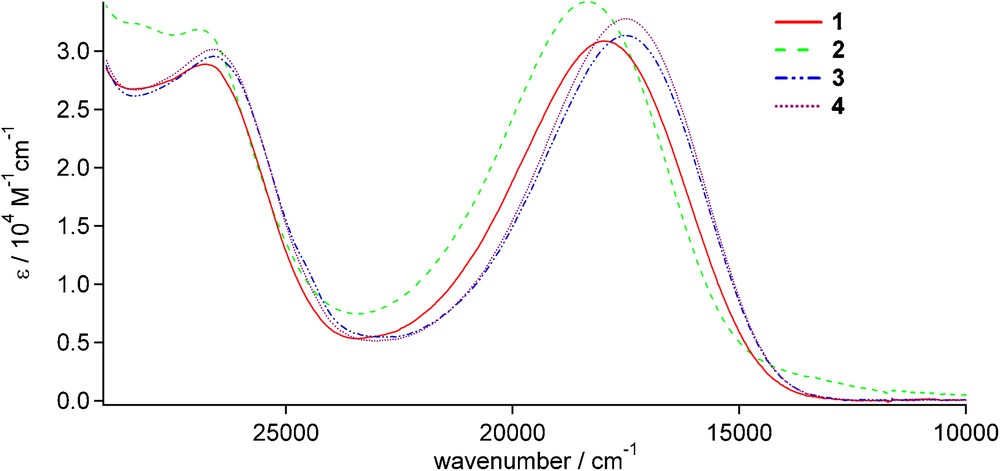
Electronic absorption spectra of 1-4 in CH2Cl2.
2.5 Magnetic properties of complexes 3 and 4
Among the four mononuclear paramagnetic lanthanide(III) compounds presented herein, the complexes 3 and 4 have been selected for an investigation by magnetic SQUID measurements. These two representative examples will demonstrate that this type of mononuclear complexes exhibit predictable magnetic signatures. In the case of 3, the thermal variation of χMT together with the inverse susceptibility vs temperature curve and the magnetization data are presented in Fig. 5. The χMT product exhibits a value of 7.74 cm3 K mol−1 at room temperature which is very close to the expected value of 7.88 cm3 K mol−1 for the spin-only center of a Gd(III) ion with an 8S7/2 ground state (S = 7/2, g = 2) [8]. Since there is no spin-orbit coupling and the first electronic excited state is about 30,000 cm−1 above the ground state, no deviation from the Curie law is expected and accordingly, the experimental χMT values stay practically constant over the whole temperature range. Similarly, the inverse susceptibility curve shows a linear behaviour in the temperature range from 50 to 290 K and the Curie constant C gives a value of 61.5 Nβ2/3k, what compares well with the theoretical value 63.0 Nβ2/3k. The magnetization data which are taken at 1.9 K reach a magnetic moment value of 6.37 μB with a magnetic field of 5 T; this value is already quite close to the theoretical saturation value of 7 μB.
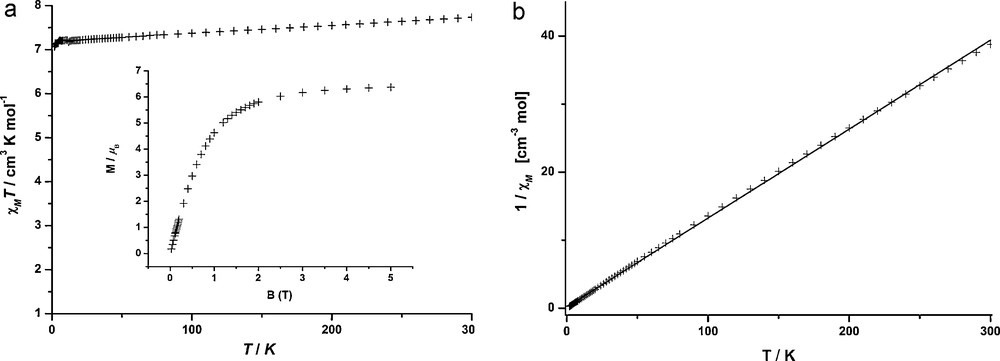
a: thermal variation of χMT and magnetization versus field at 1.9 K (inset) for 3; b: inverse magnetic susceptibility versus temperature (solid line is a fit from 50 to 290 K) for 3.
Fig. 6 illustrates the magnetic behaviour of complex 4 including the χMT(T) curve, the inverse susceptibility vs temperature curve and the magnetization data. The Tb(III) ion is described with a 7F6 ground state (S = 3, L = 3, J = 6, gJ = 3/2), thus the theoretical χMT value is 11.82 cm3 K mol−1. At room temperature, the experimental χMT value is 11.25 cm3 K mol−1, which is close to the theoretical one. For Tb(III), the first electronic excited multiplet is separated by more than 1000 cm−1 from the ground state and importantly, the latter is split in Stark sub-levels; the relevant crystal field effects are of the order of 100 cm−1. Consequently, a deviation from the Curie law is expected through a depopulation of these sub-levels when the temperature is decreased. Accordingly, the experimental χMT values decrease continually while lowering the temperature. A fit over the 50 to 290 K temperature range of the inverse susceptibility data revealed a C value of 88.9 Nβ2/3k (94.5 Nβ2/3k in theory). The magnetization data which are taken at 1.9 K show a magnetic moment value of 4.33 μB with a magnetic field of 5 T, close to a saturation value.

a: thermal variation of χMT and magnetization versus field at 1.9 K (inset) for 4; b: inverse magnetic susceptibility versus temperature (solid line is a fit from 50 to 290 K) for 4.
3 Conclusion
In conclusion, four new lanthanide complexes with the π-conjugated redox-active chromophoric TTF-dppz ligand were synthesized and characterized. They form stable and well defined complexes exhibiting an intense colour. Further studies to probe the TTF-dppz ligand as an antenna for the sensitization of NIR emitters also in function of the redox states of the ligand is in progress in our laboratory.
4 Experimental
4.1 Synthesis
4.1.1 General methods and instrumentation
Unless stated otherwise, all reagents were purchased from commercial sources and used without additional purification. The compound 4′,5′-bis-(propylthio)tetrathiafulvenyl[i]dipyrido[3,2-a:2′,3′-c]phenazine (TTF-dppz) was prepared according to literature procedure [5a]. Elemental analyses were performed on an EA 1110 Elemental Analyzer CHN Carlo Erba Instruments. FTIR data was collected on a Perkin-Elmer Spectrum One spectrometer at a resolution of 4 cm−1. Cyclic voltammetric measurements were conducted on a VA-Stand 663 electrochemical analyzer.
4.1.2 Lanthanide complexation procedure
To a solution of [Ln(NO3)3]·xH2O (Ln(III) = Nd, Eu, Gd, Tb) (1 equiv.) in ethanol (10 mL) in a 50 mL beaker was added a solution of 4′,5′-bis-(propylthio)tetrathiafulvenyl[i]dipyrido[3,2-a:2′,3′-c]phenazine (TTF-dppz) ligand (2 equiv.) in CH2Cl2 (5 mL). The resulting deep blue mixture was stirred for 3 h at r.t. A deep blue precipitate was immediately formed, filtered off and washed with ethanol (3 × 20 mL). The collected solid was dried under vacuum to afford the corresponding target complex as a deep blue powder.
[Nd(NO3)3(TTF-dppz)2] (1). Yield: 52.7 mg (66.9%); IR (KBr, cm−1): 2958, 2926, 1638, 1486, 1400, 1384, 1287, 1088, 737; Anal. calcd for C56H44N11NdO9S12: C, 43.56; H, 2.87; N, 9.98. Found: C, 43.29; H, 2.93; N, 9.62.
[Eu(NO3)3(TTF-dppz)2] (2). Yield: 53.6 mg (72.1%); IR (KBr, cm−1): 2962, 2926, 1631, 1486, 1400, 1384, 1291, 1089, 737; Anal. calcd for C56H44EuN11O9S12·H2O: C, 42.85; H, 2.95; N, 9.68. Found: C, 42.79; H, 2.87; N, 9.43.
[Gd(NO3)3(TTF-dppz)2] (3). Yield: 66.8 mg (72.0%); IR (KBr, cm−1): 2959, 2922, 1656, 1486, 1401, 1384, 1290, 1088, 737; Anal. calcd for C56H44GdN11O9S12: C, 43.20; H, 2.85; N, 9.90. Found: C, 42.88; H, 2.87; N, 9.72.
[Tb(NO3)3(TTF-dppz)2] (4). Yield: 61.3 mg (65.3%); IR (KBr, cm−1): 2958, 2929, 1631, 1487, 1400, 1384, 1291, 1089, 737; Anal. Calcd (%) for C56H44N11O9S12Tb: C, 43.15; H, 2.85; N, 9.24. Found: C, 43.14; H, 2.91; N, 9.47.
4.2 X-ray crystallography
X-ray data were measured on an Oxford Diffraction SuperNova area-detector diffractometer using mirror optics monochromated Mo Kα radiation (λ = 0.71073 Å). The crystal was non-merohedrally twinned with a minor twin component of 10%. Data reduction was performed only on the reflections of the major twin component using the CrysAlisPro program [10]. The intensities were corrected for Lorentz and polarization effects, and an absorption correction based on the multi-scan method using SCALE3 ABSPACK routine in CrysAlisPro [10] was applied. The crystal did not scatter to higher resolution ranges, i.e. the mean I/sigma(I) drops to 2.16 in the resolution range 0.98–0.93 Å. The structure was solved by direct methods using SIR92 [11], which revealed the positions of only a part of the non-hydrogen atoms. One bis-propylthio-TTF fragment is disordered over two conformations with a population ratio of 0.436/0.564. One propylthio group is 0.5/0.5 disordered over two sites. All other propylthio groups have large adp's but no disorder model could be derived. The 1,2 and 1,3 distances of all disordered fragments and of all propylthio groups were restrained to the distances of the free ligand [5a]. The adp's of all disordered atoms and the ones of the propylthio groups were kept isotropic and were restrained to be similar (SHELX SIMU instruction). The non-hydrogen and not disordered atoms were refined anisotropically, but their adp's had to be restrained by the SHELX ISOR instruction. The H-atoms were placed in geometrically calculated positions and refined using a riding model where each H-atom was assigned a fixed isotropic displacement parameter with a value equal to 1.2 Ueq of its parent atom (1.5 Ueq for the methyl groups). Refinement of the structure was carried out on F2 using full-matrix least-squares procedures, which minimized the function Σw(Fo2–Fc2)2. The weighting scheme was based on counting statistics and included a factor to down-weight the intense reflections. All calculations were performed using the SHELXL-97 program [12]. Data collection and refinement parameters are listed in Table 2.
Crystal data and structure refinement for 3.
Empirical formula | C56H44GdN11O9S12 |
Formula weight | 1556.99 |
Temperature | 173(2) K |
Wavelength | 0.71073 Å |
Crystal system | Orthorhombic |
Space group | F d d 2 |
Unit cell dimensions | a = 56.3714(8) Å |
b = 47.6098(6) Å | |
c = 43.4418(6) Å | |
Volume | 116590(3) Å3 |
Z, Z’ | 64, 4 |
Density (calculated) | 1.419 Mg/m3 |
Absorption coefficient | 1.31 mm−1 |
F(000) | 50240 |
Crystal size | 0.292 × 0.103 × 0.073 mm3 |
Theta range for data collection | 1.46 to 26.04° |
Index ranges | −69 ≤ h ≤ 68, −58 ≤ k ≤ 58, −52 ≤ l ≤53 |
Reflections collected | 259053 |
Independent reflections | 53903 [R(int) = 0.0692] |
Completeness to theta = 25° | 99.8% |
Max. and min. transmission | 1 and 0.93075 |
Refinement method | Full-matrix least-squares on F2 |
Data/restraints/parameters | 53903/1881/2680 |
Goodness-of-fit on F2 | 1.667 |
Final R indices [I > 2sigma(I)] | R1 = 0.1062, wR2 = 0.249 |
R indices (all data) | R1 = 0.1672, wR2 = 0.2665 |
Largest diff. peak and hole | 1.195 and −0.969 e·Å−3 |
Acknowledgment
Support for this research by the Swiss National Science Foundation (grant No. 200020-130266/1) is gratefully acknowledged. The X-ray diffraction analysis was possible thanks to the Swiss National Science Foundation (R’Equip project 206021_128724).
Supplementary material
Crystallographic data have been deposited with the Cambridge Crystallographic Data Centre, 12 Union Road, Cambridge CB2 1EZ, UK, as supplementary material No. SUP 857998 and can be obtained by contacting the CCDC (fax: +44 1223 336 033; deposit@ccdc.cam.ac.uk).