1 Introduction
In the field of molecule-based magnetism, one-dimensional magnetic systems have attracted attention for many years [1]. The discoveries of bimetallic chain compounds [2] and polymeric transition metal complexes with bridging nitronylnitroxyde radical ligands [3] exemplify how modern coordination chemists are able to design matter at the molecular level to synthesize magnetic one-dimensional compounds to model and experimentally illustrate basic physic problems such as coupling mechanisms between different spin carriers or the effect of low-dimensionality on the magnetic properties. More recently, Single-Chain Magnets (SCM) [4,5] in which ferro-, ferri-magnetic or uncompensated canted spin configurations along the chains are associated with a large magnetic anisotropy, also raised a strong interest and an active research topic. In this context, paramagnetic dithiolene complexes were rarely investigated as paramagnetic bridging coordinating centers between transition metal complexes in the same way nitronylnitroxydes do through N–O•••MLn••• interactions. For example, the nitrile moieties of paramagnetic complexes such as Ni(mnt)2−•, Ni(adt)2−• [6], or Ni(tfadt)2–• complexes [7] (Scheme 1) are able to engage in secondary coordination with MLn metal centers, forming extended chains through–CN••MLn••• coordination, as for example with Na(18-crown-6)+ cations [7], MnIII metal ions of Mn(tetraphenylporphyrin)+ [8], Cu[bis(ethylenediamine)]2+ [9] or trimetallic [Cu2Ln]3+ tectons [10].
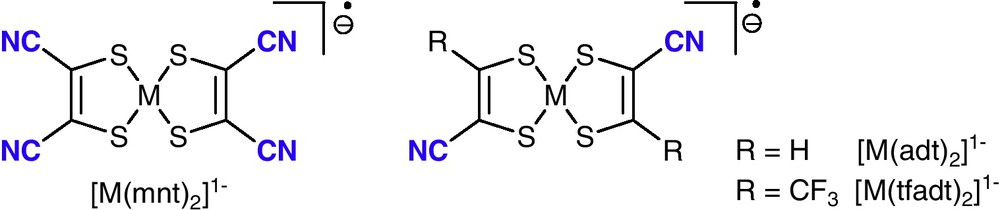
In all cases, antiferromagnetic interactions were systematically observed, either between overlapping [Ni(dithiolene)2]– radical anions [10], or between [Ni(dithiolene)2]– and the paramagnetic metal center [7,8]. In these salts, the SOMO of the paramagnetic dithiolene complex, [Ni(dithiolene)2]–, is the out-of-phase combination of the two dithiolate ligand orbitals with a Nickel d orbital, giving this SOMO a π-type character, with strong delocalization on the ligands (Scheme 2) [11,12]. Recent calculations give a 40% residual spin density on the nickel atom. If one moves from the group 10 nickel complexes to the group 11 copper complexes, the SOMO of the dianionic copper complexes, formulated as [Cu(dithiolene)2]2–, is now built out of the dx2–y2 copper orbital interacting with thiolate moieties, giving this frontier orbital a σ-type character [13,14]. It is therefore anticipated that interactions of either the formally d7 nickel anionic complexes or the d9 copper dianionic complexes, with other metallic centers through secondary coordination, should lead to totally different magnetic interactions despite similar coordination patterns. Note that in both cases, the nitrogen lone pair of the nitrile is not engaged in the SOMO, implying that magnetic interactions through coordination will be always weak.

The contrasted characteristics of the SOMO of the π-type [Ni(mnt)2]– and σ-type [Cu(mnt)2]2– radical species. Adapted from ref. [14c].
We have recently disclosed that the nitrile-containing paramagnetic copper complex6 [Cu(tfadt)2]2– was able to coordinate [Ni(cyclam)]2+ species through two trans CN•••Ni interactions leading to the formation of alternated S = 1/S = ½ spin chains with ferromagnetic interactions. In our following attempts to prepare other such systems, we have successfully isolated and characterized an analogous salt involving [Cu(en)2]2+ as dication and [Cu(mnt)2]2– as dianion which organizes into chains through two trans CN•••Cu interactions, as detailed below.
2 Results and discussion
2.1 Syntheses and structure
The preparation of the title compound is based on the metathesis reaction between [nBu4N]2[Cu(mnt)2] with [Cu(en)2](BF4)2 in CH3CN. Crystals were obtained after partial evaporation of the mixed solutions. The salt crystallizes in the monoclinic system, space group P21/c, with both dicationic and dianionic complexes located on inversion centers (Fig. 1). Intramolecular bond distances and angles are collected in Table 1.
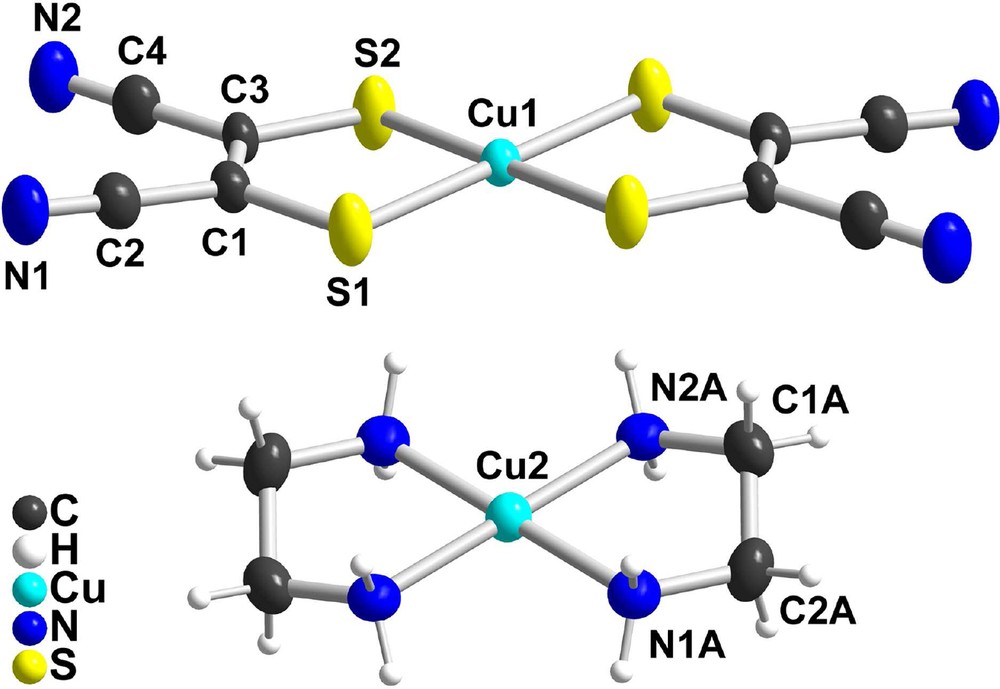
ORTEP drawing of (a) [Cu(mnt)2]2– and (b) [Cu(en)2]2+ moieties with the selected atom-labeling schemes and thermal ellipsoids at 50% probability.
Selected bond distances and angles in [Cu(en)2][Cu(mnt)2].
[Cu(mnt)2]2– | [Cu(en)2]2+ | ||
Bond distances (Å) | |||
Cu1–S1 | 2.2616(10) | Cu2–N1A | 1.998(3) |
Cu1–S2 | 2.2739(10) | Cu2–N2A | 2.015(3) |
S1–C1 | 1.727(4) | N1A–C2A | 1.477(5) |
S2–C3 | 1.733(3) | N2A–C1A | 1.475(5) |
C1–C3 | 1.360(5) | C1A–C2A | 1.495(5) |
Bond angles (°) | |||
S1–Cu1–S2 | 90.76(4) | N1A–Cu2–N2A | 84.22(12) |
Cu1–S1–C1 | 101.55(12) | Cu2–N1A–C2A | 110.3(2) |
Cu1–S2–C3 | 101.59(13) | Cu2–N2A–C1A | 108.8(2) |
S1–C1–C3 | 122.5(3) | N1A–C2A–C1A | 108.5(3) |
S2–C3–C1 | 122.8(3) | N2A–C1A–C2A | 108.4(3) |
In the solid state, the dicationic and dianionic moieties are organized into layers, perpendicular to the c axis (Fig. 2). Within a layer, we observe the formation of chains through the coordination of the Cu(II) center in [Cu(en)2]2+ by the nitrile substituent of the mnt ligand of the [Cu(mnt)2]2– unit with a N(1)•••Cu(2) distance of 2.664(4) Å (Fig. 3). The coordination deviates from linearity as the C≡N•••Cu angle amounts to 146.3(3)°. As a consequence, the magnetic interaction between the two spin carriers through this C≡N•••Cu coordination is expected to be very weak. Furthermore, as a sizeable part of the spin density is delocalized on the sulfur atoms in [Cu(mnt)2]2–, intermolecular S•••S contacts might also provide paths for direct exchange interactions. The shortest S•••S contact, S(2)•••S(2) = 3.84(2) Å, is close to van der Waals contact distance and would lead to the formation of uniform spin chain running along a (Fig. 3).

View of the unit cell of [Cu(en)2][Cu(mnt)2] projected in the bc plane.
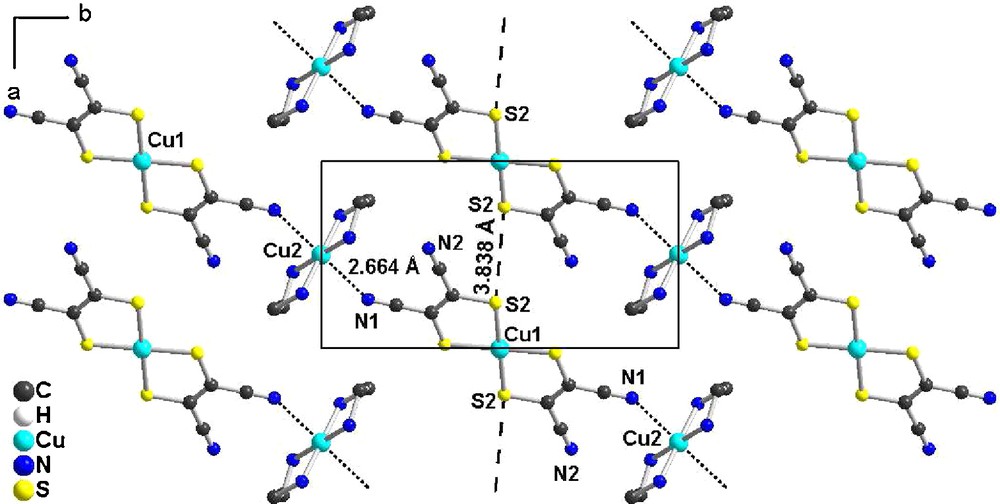
View of the coordination chains running along (a + b) in [Cu(en)2][Cu(mnt)2] projected in the ab plane. Hydrogen atoms were omitted for clarity.
2.2 Magnetic properties
The temperature dependence of the magnetic susceptibility is given on Fig. 4 as χT product vs. T plot. As a first approach and based on the structure of this compound, the magnetic susceptibility has been modeled taking into account only the coordination chain formed by alternating [Cu(mnt)2]2– and [Cu(en)2]2+ units. Therefore an isotropic regular chain model of S = ½ quantum spins has been used to fit the experimental data with the following spin Hamiltonian: H = − 2J∑SiSi+1 with J being the intra-chain magnetic interaction between [Cu(mnt)2]2– and [Cu(en)2]2+ spin carriers [2]. The best set of parameters obtained considering the experimental data down to 10 K is J/kB = –3.8(5) K and g = 2.02(5) (note that this last value is significantly higher than 2 as expected for Cu(II) centers). As shown on Fig. 4 (red line), this model is not able to reproduce the data below 10 K, leading to a theoretical χT product much lower than experimentally observed. Furthermore, the introduction of antiferromagnetic interchain interaction (through intermolecular S•••S contacts between [Cu(mnt)2] anions) cannot improve the quality of the obtained model as it will only further lower the theoretical χT product.
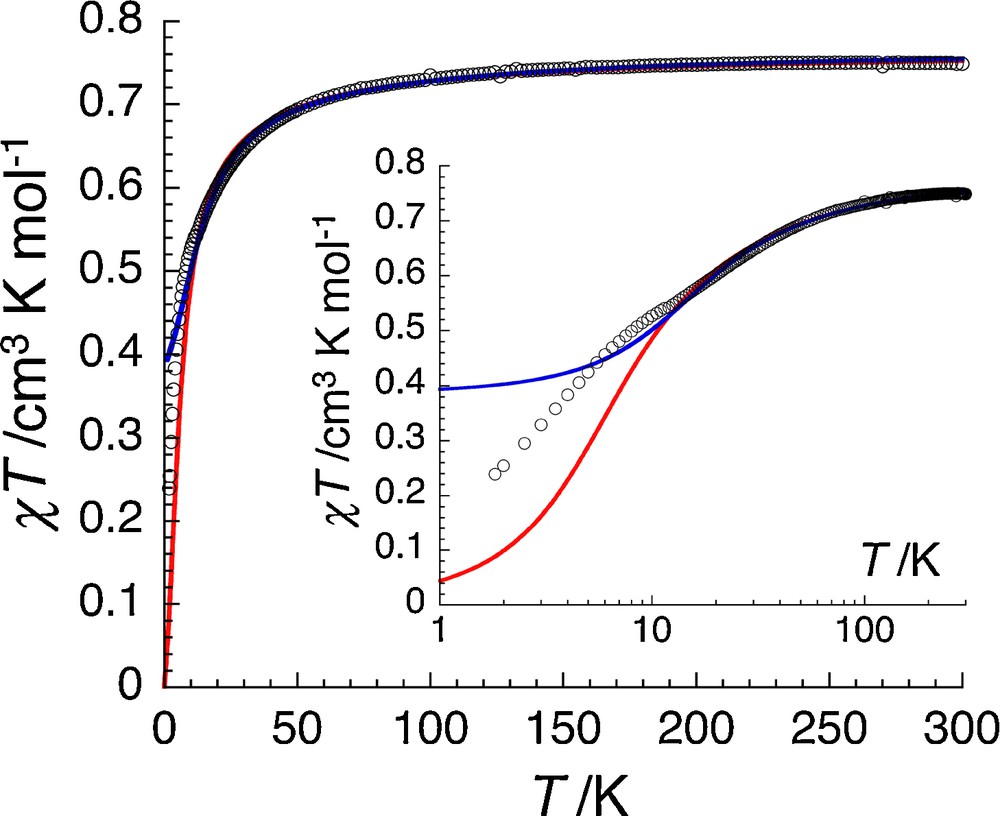
Temperature dependence of the χT product at 1000 Oe for [Cu(en)2][Cu(mnt)2] (with χ defined as the molar magnetic susceptibility equal to M/H per [Cu(en)2][Cu(mnt)2] unit) in linear (main) and semi-logarithm (inset) plots. Solid red and blue lines indicate the best fits obtained with the models described in the text.
This result demonstrates that the dominant antiferromagnetic interactions in this system are likely mediated by the S•••S contacts between [Cu(mnt)2] units and not between [Cu(mnt)2]2– and [Cu(en)2]2+ units along the coordination chain. Hence, an alternative model has been used considering only the [Cu(mnt)2]•••[Cu(mnt)2] antiferromagnetic interactions in the frame of an isotropic regular chain model of S = ½ quantum spins (see the Hamiltonian above) and treating the [Cu(en)2]2+ cations as a Curie contribution. Using this approach, the theoretical curve (Fig. 4, blue line) is able to reproduce the measured susceptibility down to 10 K with J/kB = –9.6(5) K and g = 2.03(5). This time, the calculated χT product is closer and slightly higher than the experimental data below 10 K. This makes this approach more realistic considering that the presence of antiferromagnetic [Cu(en)2]•••[Cu(mnt)2] interactions should further lower and adjust the estimated χT product. Unfortunately, an analytical 2D network model of S = ½ quantum spins with two different J parameters is not available to further analyze these magnetic data. Nevertheless on the basis of the previous analyses, it can be speculated that the [Cu(mnt)2]•••[Cu(mnt)2] antiferromagnetic interaction is likely falling in the –5 and –9 K range while the [Cu(en)2]•••[Cu(mnt)2] coupling should not exceed –1 K.
In conclusion, the paramagnetic dithiolene complex [Cu(mnt)2]2– (mnt: 1,2-dicyanoethylene-1,2-dithiolate) has been coordinated to the dicationic [Cu(en)2]2+ (en: ethylenediamine) complex to form chains of alternating [Cu(mnt)2]2– and [Cu(en)2]2+ moieties through C≡N•••Cu•••N≡C trans coordination. As shown by the magnetic susceptibility measurements and their analysis, the [Cu(en)2][Cu(mnt)2] salt is better described as a two-dimensional magnetic network with dominating [Cu(mnt)2]•••[Cu(mnt)2] antiferromagnetic interactions through intermolecular S•••S contacts and much weaker [Cu(en)2]•••[Cu(mnt)2] antiferromagnetic interactions mediated by direct coordination.
3 Experimental
3.1 Synthesis
[Cu(en)2Cu(mnt)2]. A solution of [Cu(en)2(BF4)2] [15] (10 mg, 0.028 mmol) in CH3CN is mixed with a solution of (TBA)2[Cu(mnt)2] [16] (23 mg, 0.028 mmol) in CH3CN (1 mL). Slow evaporation of half of the solvent yielded [Cu(en)2Cu(mnt)2] (10 mg, 0.019 mmol, 68%) as red brown platelets. Mp > 300 °C. Elem. Anal. Calcd. for C12H16Cu2N8S4 (Found): C, 27.31 (27.57); H, 3.06 (3.03); N, 21.24 (21.38). IR (ATR): .
3.2 Crystallography
Single crystals were mounted on the top of a thin glass fiber. Data were collected on a Stoe IPDS-I diffractometer at room temperature with graphite-monochromated Mo-Kα radiation (λ = 0.71073 Å). Structures were solved by direct methods (SHELXS-97) and refined (SHELXL-97) by full-matrix least-squares methods, [17] as implemented in the WinGX software package [18]. Absorption corrections were applied. Hydrogen atoms were introduced at calculated positions (riding model), included in structure factor calculations, and not refined. Crystallographic data: C12H16Cu2N8S4, M = 527.65, monoclinic, P21/c, a = 7.8817(11), b = 14.220(3), c = 9.2313(13) Å, β = 108.464(16)°, V = 981.4(3) Å3, Z = 2, Dc = 1.786 g cm−3, T = 293(2) K, 9357 reflections collected, 1839 unique (Rint = 0.0916), R1 [I > 2σ(I)] = 0.0304, wR2 (F, all data) = 0.0611, GoF = 0.803. CCDC-862006 contains the supplementary crystallographic data for this paper. These data can be obtained free of charge from The Cambridge Crystallographic Data Centre via www.ccdc.cam.ac.uk/data_request.cif.
3.3 Magnetic characterizations
The magnetic susceptibility measurements were obtained with the use of a Quantum Design SQUID magnetometer MPMS-XL. This magnetometer works between 1.8 and 400 K for dc applied fields ranging from –7 to 7 T. Measurements were performed on a polycrystalline sample of 28.06 mg introduced in a polyethylene bag (3 × 0.5 × 0.02 cm). M vs H measurements has been performed at 100 K to check for the presence of ferromagnetic impurities that has been found absent. The magnetic data were corrected for the sample holder and the diamagnetic contribution calculated from the Pascal's constants [19].
Acknowledgements
Financial supports from the Centre national de la recherche scientifique (CNRS), the University of Bordeaux, the Université Rennes 1, the Conseil régional d’Aquitaine, GIS Advanced Materials in Aquitaine (COMET Project), the ANR through contract n° ANR-09-BLAN-0175-04 (to R.C.; NT09_469563, AC-MAGnets project) and ANR-09-BLAN-0173-03 (to M. F.) are gratefully acknowledged.