1 Introduction
The synthesis of highly functionalized nitrogen containing heterocycles in the context of their wide array of biological activity has always attracted medicinal chemists for a long time. The synthesis of these scaffolds using multi-component coupling reactions (MCRs) represents a highly valuable synthetic tool for the construction of novel and complex molecular structures with a minimum number of synthetic steps. These types of reactions have several possible advantages over the traditional linear synthesis, for instance, lower working costs, shorter reaction times, high degrees of atom economy, the possibility for combinatorial surveying of structural variations, and environmental friendliness.
2-amino-1,3-thiazine, a versatile pharmocophore, has been the subject of great interest due to its wide range of biological activities viz., antimicrobial agents [1], as cannabinoid receptor agonists [2], in the phagocytic activity of human neutrophils [3], antioxidant properties [4], analgesic and anti-inflammatory activities [5], antifilarial properties [6], metalloprotease inhibition activity [7], antihypertensive activity [8], cholecystokinin antagonists [9], and antimycobacterial [10]. Hence, considerable efforts have been carried out for the synthesis of 1,3-thiazines through condensation of thiourea with α,β-unsaturated carboxylic acids in phosphoric acid or sulfuric acid, addition reaction of ethylenethiourea, thiourea, ethyl thioglycolate, or O-ethyl allylthiocarbamate to β-haloacids bearing compounds, microwave irradiated reaction between formaldehyde, amine and phenol, through multicomponent reaction (MCR) of in situ-generated 1-azadienes with carbon disulfide and via reacting cyanoacetamide with isothio-cyanates and cycloketones in a one-pot manner to give rise to spiro-substituted 1,3-thiazine analogues. However, due to the long reaction times, harsh reaction conditions, toxicity and difficulty in product separation of previously reported methods, synthesis of 1,3-thiazine skeleton always remains a matter of investigation for the organic as well as medicinal chemists and is reported extensively in the literature [5,10–17]. Therefore, development of a simple, nature friendly, efficient and cost effective benign methodology for such organic reactions is needed.
Cerium (IV) ammonium nitrate (CAN), among the electron transfer oxidizing agents, is considered as an important catalyst to carry out a variety of organic transformations, for instance, oxidation [18], 1,4-addition [19], protection [20], nitration [21], 1,3-dipolar cycloaddition [22], thiocyanation [23], esterification [24], and the Hantzsch reaction [25] via generation of radical intermediates. In addition to that, it has been associated with many advantages such as excellent solubility in water, inexpensiveness, eco-friendly nature, uncomplicated handling, high reactivity, fast conversions and convenient work up procedures.
The use of organic solvents as media to provide a homogeneous phase that allows molecular interactions effectively and brings the reaction to completion in conventional synthetic procedures is harmful and does not compel the reaction to the total completion. The inexpensiveness, ease of workup, thermally stability, non-toxicity, and recyclability in various organic transformations has made polyethylene glycol (PEG-400) a green solvent to carry out the variety of reactions. Prompted by the green aspects of above two chemicals, we reveal herein the CAN catalysed one-pot MCR green synthesis of 1,3-thiazine derivatives using acetophenone, aromatic aldehydes and thiourea in PEG-400 as reaction media (Fig. 1).
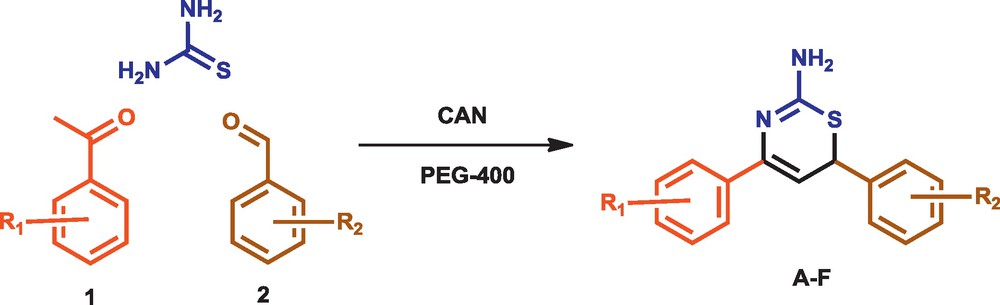
Synthesis of 1,3-thiazine.
2 Results and discussions
The first part of the study was aimed at optimizing the reaction conditions. To do this, in the first instance, we explain the effect of various concentration of CAN and temperature on reaction as well as the product yield. In this regard, as depicted from Tables 1 and 2, reactions were carried out with and without using CAN (blank) at room and at higher temperatures. We observed that reaction of equimolar concentration of aldehyde, acetophenone and thiourea using polyethylene glycol (PEG-400) as a solvent medium, with the addition of CAN at 45 °C improved yields as well decreased in total reaction time to afford required 1,3-thiazine in sequential one-pot reaction (Table 1). As the matter of fact, we found that rise in temperature directs the reaction to the completion in shorter periods of time; nevertheless, it also causes reduction in the product yield, which may be due to the decomposition at higher temperatures. Whereas, under the similar set of conditions, addition of 10% CAN lead to 66% higher product yield and saves almost 14 h of reaction time, for instance, 95% in 7 h. Unfortunately, further inflating concentration to 15%, led to the downfall in reaction time and yield as well. Through these experiments, a temperature of 45 °C along with 10% CAN were the optimal condition to complete the reaction in efficient way.
Catalytic activity evaluation for reactiona.
Entry | CAN (%) | Time (h) | Yieldb |
1 | 0 | 28 | 29 |
2 | 2 | 12 | 52 |
3 | 5 | 8 | 81 |
4 | 10 | 7 | 95 |
5 | 15 | 6 | 89 |
a Reaction conditions: aldehyde (1 mmol), acetophenone (1 mmol), thiourea (1 mmol); solvent PEG-400; 45 °C.
b Isolated and unoptimised yields.
Effect of the temperature on reactiona.
Entry | Temp | Time (h) | Yieldb |
1 | Room temperature | 8 | 93 |
2 | 45 | 7 | 95 |
3 | 60 | 6 | 85 |
4 | 80 | 5 | 78 |
a Reaction conditions: aldehyde (1 mmol), acetophenone (1 mmol), thiourea (1 mmol); solvent PEG-400.
b Isolated and unoptimised yields.
Based on the above results and following the reaction optimization, we decided to explore the recyclability of solvent PEG-400. It was surprised to see that, it can be efficiently recycled for three consecutive runs. To our knowledge, at the third run, PEG-400 was operating well to achieve 74% product yield (Table 3). It was predicted that the solvent recovery was efficient with a gradual decline in yield of product. To exemplify the versatility of this MCR for synthesis of different 1,3-thiazines, a variety of electron donating or withdrawing groups were used for a library construction. As seen from Table 4, with electronic and steric variation on the aromatic ring, the corresponding products were obtained in good yields (87%–93%). An electron withdrawing nitro group in substrates B and D reduces product yield with no variation in reaction time, whereas, an electron releasing group (-OH) as in case of C, acquiesced with shorter reaction time and high yield as 93% in 6 h. It is interesting to accept that the isomeric replacement of chloro group from position 2 to 4 acts in accordance with improved yield and reaction time as well.
Recycling yields.
No of cyclesa | Fresh | Run 1 | Run 2 | Run 3 |
Yieldb (%) | 95 | 88 | 80 | 74 |
Time (h) | 7 | 7 | 7 | 7 |
a Reaction conditions: aldehyde (1 mmol), acetophenone (1 mmol), thiourea (1 mmol); solvent PEG-400; 45 °C.
b Isolated and unoptimised yields.
Synthesis of distinguished 1,3-thiazinesa.
Entry | R1 | R2 | Time required (h) | Yieldb (%) |
A | H | 2-NO2 | 7 | 91 |
B | 4-NO2 | 2-NO2 | 9 | 87 |
C | 4-OH | 2-NO2 | 6 | 93 |
D | 4-NO2 | 2-Cl | 7 | 88 |
E | 4-NO2 | 4-Cl | 8 | 90 |
F | 4-NO2 | 4-NO2 | 9 | 89 |
a Reaction conditions: aldehyde (1 mmol), acetophenone (1 mmol), thiourea (1 mmol); solvent PEG-400; 45 °C.
b Isolated and unoptimised yields.
The possible mechanism for the formation of 1,3-thiazine has been depicted in Fig. 2. The reaction was supposed to be started with reduction of Ce (IV) → Ce (III) with the oxidation of methyl of an acetophenone (i) resulting methylene radical (ii). Thus, resulting highly reactive intermediate (ii) was readily conjugated with an aldehyde (iii) to give rise to chalcone intermediate (iv). On the other hand, thiourea tautomerise (v) easily to the corresponding thiol tautomer, whereas the lone pair present on it, readily attacks on β-carbon corresponds to the carbonyl, resulting in shifting of the double bond. As a result, carbonyl undergoes tautomerisation to yield the hydroxyl. Under influence of PEG-400, this Michael adduct again will tautomerises and underwent a intermolecular cyclocondensation reaction to furnish a 1,3-thiazine ring system.
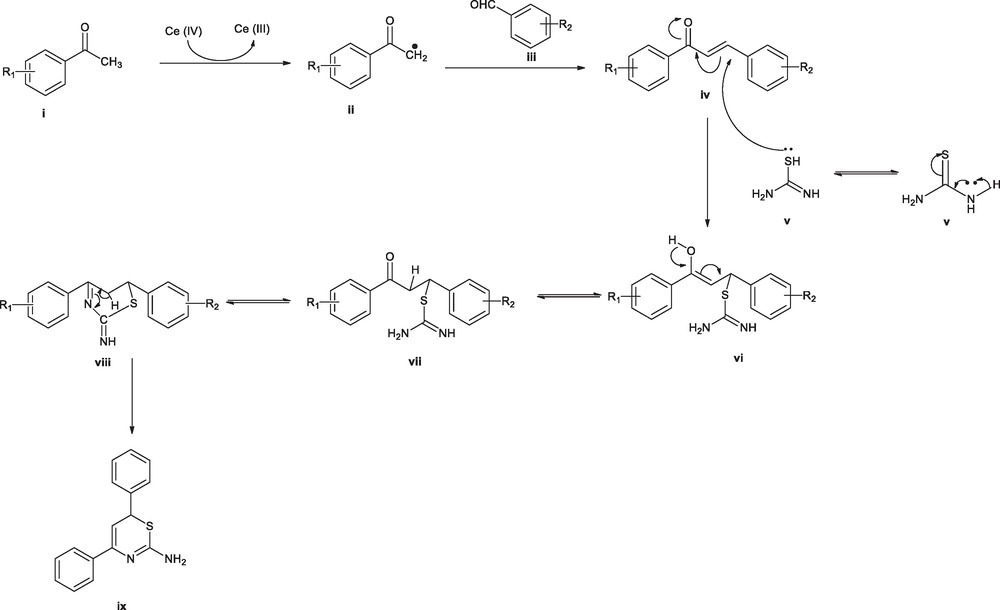
Proposed mechanism for synthesis of 1,3,-thiazine.
FTIR and NMR spectroscopy enabled the elucidation of structure of final compounds. Moreover, CHN analysis was found in agreement with proposed structures. The bands at 3426 and 3362 cm−1 observed in the spectrum are attributed to hydrogen-bonded NH stretching of primary amines. A broad absorption band nearer at 3000 cm−1 is due to the CH stretching. For 1H-NMR, the chemical shift values are ranging from 3.21 to 3.52 ppm for the 1,3-thiazine skeleton. The averaged values of the 1H chemical shift of later ones are 3.39, 5.32 and 5.75 for 2-, 6-, and 5-H respectively. It is noteworthy to mention here that the different substitution pattern brings a change in 1H chemical shift values of aromatic rings, among them, the most deshielded protons appeared in case of the nitro group containing compounds.
The growing incidence of multidrug resistant Gram-negative bacteria has seriously jeopardised patients in healthcare settings and are especially prevalent and problematic in intensive care units. This necessitates the discovery of newer antimicrobial agents which target the novel pathway for their action [26]. Recently, compounds having the core skeleton of 1,3-thiazine have been identified as inhibitors of 4-diphosphocytidyl-2-Cmethyl-D-erythritol (IspE) kinase of Gram-negative bacteria [27]. This enzyme catalyses the ATP-dependent conversion of 4-diphosphocytidyl-2C-methyl-d-erythritol (CDPME) to 4-diphosphocytidyl-2C-methyl-d-erythritol 2-phosphate (CDP-ME2P) with the release of ADP, a part of non-mevalonate pathway of isoprenoid precursor biosynthesis [28]. This pathway has been well utilised by the Gram-negative bacteria and the agents which cause disruptions of this enzyme are deemed to be lethal to the microbres. The absence of this pathway in humans renders the associated enzymes unique for the development of novel antimicrobials and is less likely to cause serious side-effects in the patients.
To assess the potential medicinal utility of these analogues, the synthesized 1,3-thiazine derivatives were rigorously screened through the parameters of Lipinski's Rule of 5 (Ro5) for its drug likeness prediction [29]. Surprisingly, it was revealed that none of the 1,3-thiazine derivatives has violated the above rule (Table 6). Encouraged by the above findings, a panel of Gram-negative bacteria was chosen to carry out the antibacterial screening studies viz., Proteus vulgaris, Escherichia coli, Pseudomonas aeruginosa. It was revealed from the Table 5, the entire analogues showed potent antibacterial activity against selected microorganisms. Out of them compound D, showed the most potent activity against E. coli and P. aeruginosa, while equipotent against P. vulgaris in comparison to standard. Compound E, revealed the equipotent antibacterial activity against E. coli in comparison to cefixime and potent against rest of the strain. It is considered as next influential molecule. The least antibacterial was observed in the case of compound C. The rest of the molecules (A, B and F) presented significant activity against tested bacteria in a comparison test with reference to standard. On close inspection, a strong correlation (r2 = 0.82, 0.76 and 0.42 against E. coli, P. vulgaris and P. aeruginosa respectively) was revealed in between miLogP and MIC of the compounds described herein.
Drug likeness of 1,3-thiazine in the Eye of Lipinski's Rule of 5.
Compounds | miLogP | TPSA | natoms | MW | nON | nOHNH | nviolations | nrotb | Volume |
A | 3.356 | 84.211 | 22 | 311.366 | 5 | 2 | 0 | 3 | 264.866 |
B | 3.315 | 130.035 | 25 | 356.363 | 8 | 2 | 0 | 4 | 288.201 |
C | 2.877 | 104.439 | 23 | 327.365 | 6 | 3 | 0 | 3 | 272.884 |
D | 4.034 | 84.211 | 23 | 345.811 | 5 | 2 | 0 | 3 | 278.402 |
E | 4.082 | 84.211 | 23 | 345.811 | 5 | 2 | 0 | 3 | 278.402 |
F | 3.363 | 130.035 | 25 | 356.363 | 8 | 2 | 0 | 4 | 288.201 |
Antibacterial activity of 1,3-thiazine compounds.
Compound | Minimum Inhibitory Concentration (MIC; μg mL−1) | ||
P. vulgaris | E. coli | P. aeruginosa | |
A | 12.5 | 12.5 | 50 |
B | 25 | 12.5 | 6.25 |
C | 25 | 25 | 50 |
D | 3.125 | 1.56 | 3.125 |
E | 6.25 | 3.12 | 12.5 |
F | 12.5 | 6.25 | 12.5 |
Cefixime | 3.125 | 3.12 | 6.25 |
It was observed that ClogP is the main influencing physico-chemical property for the generation of antibacterial activity which favourably enhances the antimicrobial activity of the compounds e.g., Compounds D and E found as the pronounced antibacterial agent along with the high LogP values. While compound C having the lowest LogP value showed least inhibitory activity. The alteration of steric effects on the phenyl ring of molecules which in turn alter the ease of penetration across the bacterial cell wall owing to the presence of electron withdrawing groups, might be the possible cause for difference in activity.
As further to enlarge the biological spectrum of 1,3-thiazine and novel insight about the mechanism, a molecular docking study of the most active 1,3-thiazine (D) has been carried out on the protein model of IspE kinase (2V34.pdb). The binding mode of compound D showed that it was deeply engulfed and stabilised into the active site of IspE kinase by making five hydrogen bonds and binding energy of −56.22, Fig. 3. The four hydrogen bonds were originated from the oxygen of NO2 present on the phenyl ring with Gly90, Ala91, Gly92, Gly95 (O…H) (Table 7). The remaining H-bond was established in between hydrogen of by NH2 and oxygen of Ile127 (H…O). This finding was suggesting that our molecules acted as a potent inhibitor of Gram-negative bacteria via inhibition of IspE kinase.
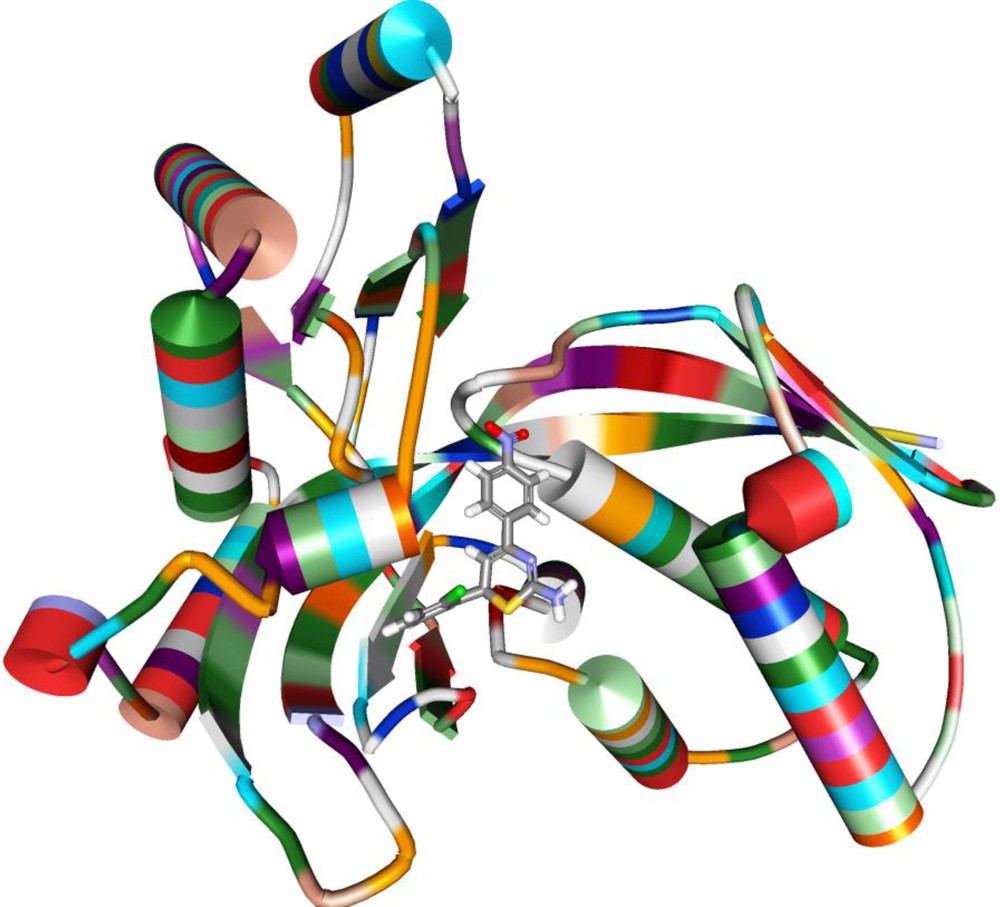
Orientation of compound D in the active site of IspE kinase.
Docking interaction of ligand (D) in complex with IspE kinase.
Compound | Hydrogen Bond (Distance in Å) |
D | A:GLY90:HN – D.mol:O16 (2.46) |
A:ALA91:HN – D.mol:O16 (2.46) | |
A:GLY92:HN – D.mol:O16 (2.05) | |
A:GLY95:HN – D.mol:O15 (2.18) | |
D.mol:H26 – A:ILE127:O (2.03) |
3 Experimental
3.1 Analytical methods
Melting points of synthesized compounds were determined in open capillary tubes in a Hicon melting point apparatus and are uncorrected. Thin layer chromatographic analysis was done to monitor the completion of reaction as well as for identification and characterization of compounds. The different mobile phases were selected according to the assumed polarity of the products. The spots were visualized by exposure to iodine vapor and UV light. The structures of the compounds were established on the basis of spectral (FT-IR, 1H-NMR) and elemental analysis. FT-IR (in 2.0 cm−1, flat, smooth, abex, KBr) spectra were recorded on Biored FTs spectrophotometer. 1H-NMR spectra were recorded on Bruker Avance II 400 and 300 MHz NMR spectrometer in CDCl3-d6 and DMSO using tetramethylsilane (TMS) as internal standard. 13C NMR spectra were recorded on Bruker Avance II 300 NMR Spectrometer. Chemical shifts are reported in parts per million (ppm, δ) and signal are described as s (singlet), d (doublet), t (triplet), q (quartet) and m (multiplet). Elemental analysis was carried out on a Vario EL III CHNOS elemental analyzer.
3.1.1 General procedure for synthesis of 1,3-thiazine derivatives
Substituted acetophenone (1 mmol), aromatic aldehydes (1 mmol) and thiourea (1 mmol) in PEG-400 were mixed. To this, CAN (ceric ammonium nitrate) was added and reaction mixture was stirred at 45 °C. The progress and completion of reaction mixture was monitored by TLC. After completion of reaction the reaction mixture was cooled in dry ice-acetone bath to precipitate the PEG-400 and extracted with ether (PEG being insoluble in ether). Ether layer was decanted, dried and concentrated under reduced pressure. The recovered PEG-400 can be reused for consecutive runs. The structures of all the products were ascertained on the basis of their spectral (IR, 1H-NMR, 13C NMR) and elemental analysis.
3.1.1.1 6-(2-Nitrophenyl)-4-phenyl-6H-1,3-thiazin-2-amine (A)
Yellow crystals; mp: 162–164 °C; Rf: 0.45; FT-IR (νmax; cm−1 KBr): 3356 (NH), 1687 (C-N), 1656–1645 (aromatic C = N), 1596 (C = C), 1520, 1355 (Ar-NO2), 877, 754; 1H-NMR (400 MHz, CDCl3-d6, TMS) δ ppm: 5.63 (s, 1H, H5), 7.33–7.30 (m, 3H), 7.66 (d, 1H, J = 7.9 Ar-H), 8.35 (d, 1H, J = 7.1 Ar-H), 5.52 (bs, 1H); 13C NMR (100 MHz, CDCl3) δ ppm: 26.8 (CH), 112.7 (CH), 120.9 (CH), 127.3 (CH), 128.1 (CH), 128.9 (CH), 135.2 (CH), 143.5 (C), 148.0 (C), 162.7 (C); Anal. Calcd. For C16H13N3O2S: C, 61.72; H 4.21; N, 13.50. Found: C, 61.68; H, 4.20; N, 13.54.
3.1.1.2 6-(2-Nitrophenyl)-4-(4-nitrophenyl)-6H-1,3-thiazin-2-amine (B)
Dark brown crystals; mp: 168–170 °C; Rf: 0.65; FT-IR (νmax; cm−1 KBr): 3360 (NH), 1683 (C-N), 1597 (C = C), 1658 (aromatic C = N), 1526, 1358 (Ar-NO2), 876, 756; 1H-NMR (400 MHz, CDCl3-d6, TMS) δ ppm: 5.68 (s, 1H, H5) 7.43–7.40 (m, 3H), 7.69 (d, 1H, J = 7.8 Ar-H), 8.06 (d, 1H, J = 7.1 Ar-H), 5.56 (bs, 1H); 13C NMR (100 MHz, CDCl3) δ ppm: 27.0 (CH), 112.8 (CH), 121.2 (CH), 127.6 (CH), 129.0 (CH), 135.4 (CH), 143.7 (C), 143.9 (C), 148.3 (C), 162.8 (C); Anal. Calcd. For C16H12N4O4S: C, 53.93; H 3.39; N, 15.72. Found: C, 53.95; H, 3.42; N, 15.72.
3.1.1.3 4-(2-Amino-6-(2-nitrophenyl)-6H-1,3-thiazin-4-yl)phenol (C)
Brownish crystals; mp: 175–178 °C; Rf: 0.54; FT-IR (νmax; cm−1 KBr): 3359 (NH), 1680 (C-N), 1610 (C = C), 1646–1635 (aromatic C = N), 1519, 1348 (Ar-NO2), 889, 778; 1H-NMR (400 MHz, CDCl3-d6, TMS) δ ppm: 5.46 (s, 1H, H5) 7.37–7.67 (m, 3H), 7.69 (d, 1H, J = 7.6 Ar-H), 8.06 (d, 1H, J = 7.1, Ar-H), 5.45 (bs, 1H); 13C NMR (100 MHz, CDCl3) δ ppm: 26.4 (CH), 112.2 (CH), 120.7 (CH), 127.0 (CH), 128.4 (CH), 134.7 (CH), 143.1 (C), 143.4 (C), 148.0 (C), 162.1 (C); Anal. Calcd. For C16H13N3O3S: C, 58.70; H 4.00; N, 12.84. Found: C, 57.50; H, 4.10; N, 12.80.
3.1.1.4 6-(2-Chlorophenyl)-4-(4-nitrophenyl)-6H-1,3-thiazin-2-amine (D)
Pale yellow crystals; mp: 183–184 °C; Rf: 0.65; FT-IR (νmax; cm−1 KBr): 3360 (NH), 1680 (C-N), 1638 (C = C), 1648–1630 (aromatic C = N), 1529, 1348 (Ar-NO2), 890, 772; 1H-NMR (400 MHz, CDCl3-d6, TMS) δ ppm: 5.73 (s, 1H, H5) 7.31–7.38 (m, 3H), 7.62 (d, 1H, J = 7.9 Ar-H), 8.09 (d, 1H, J = 7.2 Ar-H), 5.45 (bs, 1H); 13C NMR (100 MHz, CDCl3) δ ppm: 27.6 (CH), 113.5 (CH), 121.7 (CH), 128.0 (CH), 129.6 (CH), 135.9 (CH), 144.3 (C), 144.6 (C), 148.9 (C), 163.1 (C); Anal. Calcd. For C16H12ClN3O2S: C, 55.57; H 3.50; N, 12.15. Found: C, 55.60; H, 3.60; N, 12.10.
3.1.1.5 4-(4-Chlorophenyl)-6-(4-nitrophenyl)-6H-1,3-thiazin-2-amine (E)
Brown crystals; mp: 188–191 °C; Rf: 0.58; FT-IR (νmax; cm−1 KBr): 3362 (NH), 1690 (C-N), 1634 (C = C), 1650–1633 (aromatic C = N), 1535, 1350 (Ar-NO2), 880, 782; 1H-NMR (400 MHz, CDCl3-d6, TMS) δ ppm: 5.52 (s, 1H, H5) 7.35–7.36 (m, 3H), 7.56 (d, 1H, J = 7.6 Ar-H), 8.06 (d, 1H, J = 7.3, Ar-H), 5.56 (bs, 1H); 13C NMR (100 MHz, CDCl3) δ ppm: 27.2 (CH), 113.0 (CH), 121.5 (CH), 127.9 (CH), 129.2 (CH), 135.6 (CH), 143.8 (C), 144.1 (C), 148.5 (C), 162.9 (C); Anal. Calcd. For C16H12ClN3O2S: C, 55.57; H 3.50; N, 12.15. Found: C, 55.57; H, 3.52; N, 12.13.
3.1.1.6 4,6-Bis (4-nitrophenyl)-6H-1,3-thiazin-2-amine (F)
Reddish orange crystals; mp: 195–197 °C; Rf: 0.57; FT-IR (νmax; cm−1 KBr): 3359 (NH), 1682 (C-N), 1635 (C = C), 1656-1630 (aromatic C = N), 1540, 1354 (Ar-NO2), 890, 756; 1H-NMR (400 MHz, CDCl3-d6, TMS): δ 5.76 (s, 1H, H5) 7.34–7.36 (m, 3H), 7.64 (d, 1H, J = 7.6 Ar-H), 8.10 (d, 1H, J = 7.3, Ar-H), 5.52 (bs, 1H); 13C NMR (100 MHz, CDCl3) δ ppm: 26.8 (CH), 112.7 (CH), 121.2 (CH), 127.5 (CH), 135.2 (CH), 143.4 (C), 143.7 (C), 146.3 (C), 148.2 (C), 162.6 (C); Anal. Calcd. For C16H12N4O4S: C, 53.93; H 3.39; N, 15.72. Found: C, 53.90; H, 3.44; N, 15.70.
3.2 Biological and computational studies
3.2.1 Antibacterial activity
3.2.1.1 Minimum Inhibitory Concentration
Entire compounds were screened for their minimum inhibitory concentration (MIC, μg/mL) against selected Gram-negative organisms, viz. P. vulgaris (NCIM-2027), E. coli (NCIM-2065) P. aeruginosa (NCIM-2036) by the broth dilution method as recommended by the National Committee for Clinical Laboratory Standards with minor modifications. Cefexime was used as the standard antibacterial agent. Solutions of the test compounds and reference drug were prepared in dimethyl sulfoxide (DMSO) at concentrations of 100, 50, 25, 12.5, 6.25, 3.125 μg/mL. Eight tubes were prepared in duplicate with the second set being used as MIC reference controls (16–24 h visual). After sample preparation, the controls were placed in a 37 °C incubator and observed for any macroscopic growth (clear or turbid) the next day.
Into each tube, 0.8 mL of nutrient broth was pipette (tubes 2–9), tube 1 (negative control) received 1.0 mL of nutrient broth and tube 10 (positive control) received 0.9 mL of nutrient. Tube 1, the negative control, did not contain bacteria or antibiotic. The positive control, tube 10 contained bacteria but not antibiotic. The test compound were dissolved in DMSO (125 μg/mL), 0.1 mL of increasing concentration of the prepared test compounds which are serially diluted from tube 2 to tube 7 from highest (125 μg/mL) to lowest (0.97 μg/mL) concentration (tubes 2–7 containing 125, 62.5, 31.25, 15.62, 7.81, 3.91, 1.95, 0.97 μg/mL). After this process, each tube was inoculated with 0.1 mL of the bacterial suspension whose concentration corresponded to 0.5 McFarland scale (9 × 108 cells/mL) and each tube was incubated at 37 °C for 24 h at 150 rpm. The incubation chamber was kept humid. At the end of the incubation period, MIC values were recorded as the lowest concentration of the substance that gave no visible turbidity, i.e., no growth of inoculated bacteria [30].
3.2.2 Molecular docking studies
The 3D X-ray crystal structure of ISPE in complex with cytidine and ligand; 2V34:pdb) was used as starting model for this study. The protein was prepared, docked, scored and the molecular dynamics simulation carried out using standard procedures. All computational analysis was carried out using Discovery Studio 2.5 (Accelrys Software Inc., San Diego; http://www.accelrys.com).
3.2.2.1 Preparation of receptor
The target protein that complexed with the ligand was taken, the ligand was extracted, and the bond order was corrected. The hydrogen atoms were added, and their positions were optimized using the all-atom CHARMm (version c32b1) forcefield with Adopted Basis set Newton Raphson (ABNR) minimization algorithm until the root mean square (r.m.s) gradient for potential energy was less than 0.05 kcal/mol/Å [31,32]. Using the ‘Binding Site’ tool panel available in DS 2.5, the minimized IspE kinase editing domain was defined as receptor, binding site was defined as volume occupied by the ligand in the receptor, and an input site sphere was defined over the binding site with a radius of 5 Å. The centre of the sphere was taken to be the centre of the binding site, and side chains of the residues in the binding site within the radius of the sphere were assumed to be flexible during refinement of postdocking poses. The receptor having defined binding site was used for the docking studies.
3.2.2.2 Ligand set-up
Using the built-and-edit module of DS 2.5, ligand (1,3-thiazine derivative, D) was built, all-atom CHARMm forcefield parameterization was assigned and then minimized using the ABNR method. A conformational search of the ligand was carried out using a stimulated annealing molecular dynamics (MD) approach. The ligand was heated to a temperature of 700 K and then annealed to 200 K. Thirty such cycles were carried out. The transformation obtained at the end of each cycle was further subjected to local energy minimization, using the ABNR method. The 30 energy-minimized structures were then superimposed and the lowest energy conformation occurring in the major cluster was taken to be the most probable conformation.
3.2.2.3 Docking
LigandFit [33] protocol of DS 2.5 was used for the docking of ligands with ISPE kinases. The LigandFit docking algorithm combines a shape comparison filter with a Monte Carlo conformational search to generate docked poses consistent with the binding site shape. These initial poses are further refined by rigid body minimization of the ligand with respect to the grid based calculated interaction energy using the Dreiding forcefield [34]. The receptor protein conformation was kept fixed during docking, and the docked poses were further minimized using all-atom CHARMm (version c32b1) forcefield and smart minimization method (steepest descent followed by conjugate gradient) until r.m.s gradient for potential energy was less than 0.05 kcal/mol/Å. The atoms of ligand and the side chains of the residues of the receptor within 5 Å from the center of the binding site were kept flexible during minimization.
4 Conclusions
In conclusion, for the first time an unprecedented, one-pot synthesis of 1,3-thiazine derivatives in PEG-400 as reaction media has been described. This methodology is very efficient in terms of the work up and reusability of solvent in different consecutive steps which reduces the operating time and the amount of waste generated. Additionally, we have identified these 1,3-thiazines as a inhibitor of Gram-negative bacteria via arresting IspE kinase, a novel target. This study opens the new avenues for ongoing drug discovery program against MDR Gram-negative pathogens.
Acknowledgement
We are grateful to the Sam Higginbottom Institute of Agriculture, Technology & Sciences for providing necessary infrastructural facilities.