1 Introduction
Functionalized piperidines and their derivatives are a very important class of heterocyclic compounds due to a variety of biological activities [1]. Many compounds bearing this heterocyclic unit have revealed diverse and interesting biological activities, such as anti-inflammatory, antibacterial, antimalarial, anticonvulsant properties, and exhibit anti-hypertensive activities [2–5]. Moreover, some of them have been found to be useful in Parkinson's disease involving the MAO-based mechanism [6,7]. In recent times, numerous methods have been reported for the synthesis of highly substituted piperidines by using various catalytic systems such as bismuth nitrate [8], molecular iodine [9], bromodimethylsulfonium bromide (BDMS) [10], tetrabutylammonium tribromide (TBATB) [11], InCl3 [12], CAN [13], ZrOCl2·8H2O [14], and picric acid [15] from the reaction of aromatic aldehyde, amine, and acetoacetic ester. Though different approaches have been reported, there are many limitations, such as the use of expensive and excess amounts of catalysts, elevated temperatures, long reaction time, hazardous organic solvents, reagents, and low yields. Hence, the development of novel methods for the synthesis of functionalized piperidines is of great importance because of their potential biological and pharmaceutical activities. During the last two decades, rare earth metal triflates are often employed as catalysts in organic synthesis, due to their low toxicity, affordability, stability, and ease of handling [16,17]. Cerium(IV) salts have already been used as Lewis acid catalysts in nitrone cycloaddition, epoxide ring-opening, esterification reactions and protection/deprotection protocols [18–23]. In continuation of our interest in developing green synthetic methodologies for organic transformations [24–27], we turned our attention toward the five-component condensation of methyl acetoacetate, two equivalents of aldehyde and two equivalents of amine in acetonitrile as a solvent. Herein, we describe a new, efficient, and green procedure for the synthesis of highly functionalized piperidine derivatives 4 in good yields using commercially available cerium(IV) triflate as an inexpensive and environmentally benign catalyst (Scheme 1).

Multicomponent one-pot synthesis of functionalized piperidines.
2 Results and discussion
In preliminary experiments, the model reaction of benzaldehyde (2.0 mmol), aniline (2.0 mmol), and ethyl acetoacetate (1.0 mmol) catalyzed by cerium(IV) triflate was conducted to screen the optimal reaction conditions and the results are listed in Table 1. It is noteworthy that in the absence of catalyst, the reaction failed to give the desired product, even after long reaction times (24 h, Table 1, entry 1). To determine the appropriate concentration of the catalyst, that is, Ce(OTf)4, the model reaction was investigated at different concentrations of Ce(OTf)4, such as 0.5, 1, and 5 mol%. This study revealed that the product was formed in 60%, 85%, and 86% yields, respectively. This indicates that 1 mol% of Ce(OTf)4 is enough to carry out the reaction efficiently. The reaction was also examined in solvents such as H2O, THF, CH2Cl2, toluene, and diethyl ether. In the presence of solvents, the reaction was sluggish, and the formation of byproducts was observed (Table 1, entries 5–9). Moreover, when the reaction was carried out in acetonitrile medium, the solid product was separated at the end of the reaction.
Effect of different Ce(OTf)4 and solvent on the formation of 4.
Entry | Ce(OTf)4 (mol%) | Condition/solvent | Time (h)/yield |
1 | 0 | r.t./CH3CN | 24/0 |
2 | 0.5 | r.t./CH3CN | 10/60 |
3 | 1 | r.t./CH3CN | 7/85 |
4 | 5 | r.t./CH3CN | 7/86 |
5 | 1 | r.t./THF | 24/50 |
6 | 1 | r.t./CH2Cl2 | 10/20 |
7 | 1 | r.t./H2O | 24/15 |
8 | 1 | r.t./diethyl ether | 24/10 |
9 | 1 | r.t./Tolune | 0.5/30 |
At the beginning of the reaction, the reagents itself were dissolved completely in the medium to form a homogeneous mixture (Fig. 1a), but near the completion of the reaction, the system became a suspension, and the product precipitated at the end of the reaction (Fig. 1b).
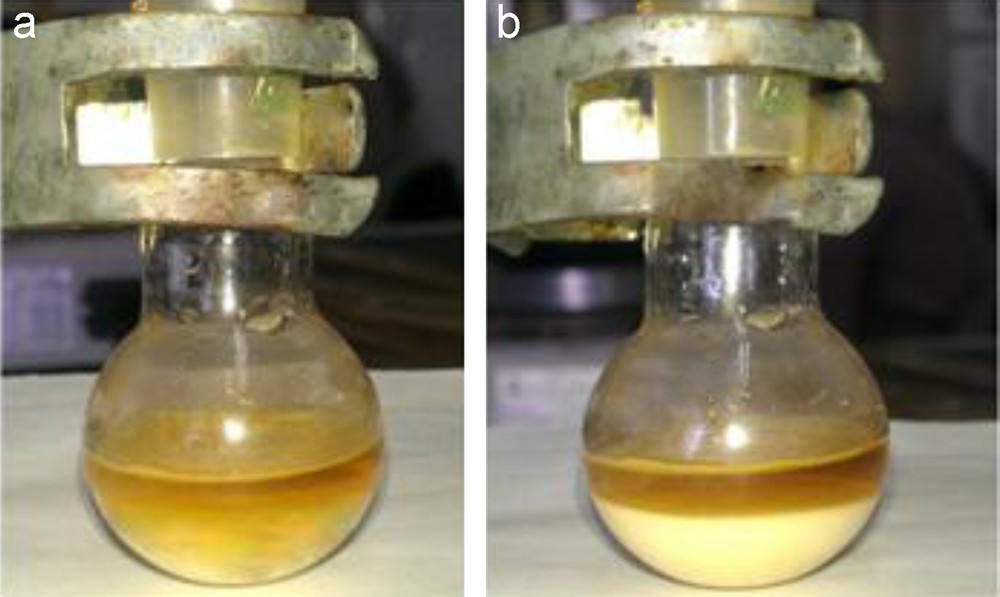
(a) Homogeneous mixture during the reaction, and (b) at the end of the reaction; the product has precipitated.
The products were obtained through simple filtering, and recrystallized from hot ethanol to afford pure products. The corresponding functionalized piperidine-scaffolds 4a, shown in Table 1 and confirmed by NMR measurements, were obtained in good yields (85%).
The scope and limitations of this five-component reaction under optimized conditions were explored using a variety of aldehydes, amines, and β-keto-esters, as summarized in Table 1. Both the electron-rich and -deficient aldehydes worked well, leading to good yields of products 5. Aromatic aldehydes with several functionalities, such as Cl, Me, F and NO2 were found to be compatible under the optimized reaction condition. The electronic effect seemed to have a slight influence on the reaction since either the electron-withdrawing or the electron-donating groups on the different aromatic ring resulted in hardly different yields.
Several aromatic amines were examined to study the generality and scope of the present protocol. Various anilines with substituents such as Me, OMe, Cl, and Br were treated with varying aromatic aldehydes and ethyl acetoacetate under identical reaction conditions. All these reactions underwent smoothly to provide the corresponding piperidine derivatives in moderate to good yields (Table 1). Unfortunately, aliphatic amines gave moderate yields (Table 1, entry 15), presumably due to the higher basicity of aliphatic amines compared to anilines. [Note: The strong affinity of acids for amino groups does not allow regeneration of the acids in the reaction. Furthermore, they are decomposed or deactivated by the amines and their derivatives when more than the stoichiometric amounts are used.] [28].
Satisfactorily, the reactions displayed high functional group tolerance and afforded the corresponding piperidine derivatives with great efficiency. The structure of the products (4a–o) was established from their IR spectral data and comparison of their melting points with those of authentic samples. Also, the structure of some products was confirmed by 1H NMR and 13C NMR spectral data. The relative anti-configuration of the product was confirmed by comparing the physical and spectroscopic data of the known compounds with those in the literature [8–15], and also the lack of a NOE between H2 and H6, which would have been expected if the relative stereochemistry of these substituents were cis [12].
A plausible mechanism for the formation of piperidine derivatives is shown in Scheme 2.

A plausible mechanism for the one-pot synthesis of functionalized piperidines.
Initially, Ce(OTf)4 can serve as a Lewis acid catalyst for the reaction of amine and alkyl acetoacetate to give the enamine I. This enamine I, then, reacts with the aromatic aldehyde to produce the Knoevenagel-type product II. Intermediate II should tautomerize to provide III, stabilized by intramolecular hydrogen bonding. Ce(OTf)4 facilitates the formation of imine as well as enamine I in this multicomponent reaction. This imine and the intermediate III undergo a[4+2] aza-Diels–Alder reaction to provide the functionalized piperidine [10] (Scheme 2).
3 Conclusions
In conclusion, an extremely efficient and green process has been developed for the synthesis of functionalized piperidines through a one-pot, five-component reaction using a catalytic amount of Ce(OTf)4 at room temperature. In contrast to the existing methods using potentially hazardous catalysts/additives, the present method offers the following competitive advantages: (i) mild reaction conditions; (ii) good yields; (iii) high diastereoseletivity; (iv) operational simplicity and the absence of tedious separation procedures; high atom-economy; (v) inexpensive starting materials; and (vi) environmentally benign catalyst are the key advantages of the present MCRs protocol.
4 Experimental
4.1 General experimental procedure for the preparation of functionalized piperidines
A mixture of β-keto ester (1.0 mmol), aldehyde (2.0 mmol), amine (2 mmol), and Ce(OTf)4 (0.01 mmol) in 4 mL of acetonitrile was stirred at room temperature for an appropriate time (Table 1). After this time, the corresponding solid product 4 was obtained through simple filtering, and recrystallized from hot ethanol to afford pure products. The products were characterized by comparison of their physical and spectral data with those of the authentic samples. Spectroscopic data for selected examples are shown below.
4.1.1 Ethyl 2,6-bis(phenyl)-1-(4-methylphenyl)-4-(4-methylphenylamino)-1,2,5,6-tetrahydropyridine-3-carboxylate (4c)
White crystals; mp 196–198 °C. IR (KBr): 3239, 3069, 2988, 2920, 2860, 1651, 1584 cm−1; 1H NMR (500 MHz, CDCl3): δ = 1.45 (t, J = 7.0 Hz, 3H), 2.32 (s, 3H), 2.33 (s, 3H), 2.75 (d, J = 15.5 Hz, 1H), 2.87 (dd, J = 15.0, 5.5 Hz, 1H), 4.28–4.48 (m, 2H), 5.10 (s, 1H), 6.29 (d, J = 6.6 Hz, 2H), 6.40 (s, 1H), 6.53 (d, J = 8.0 Hz, 2H), 6.59 (t, J = 6.6 Hz, 1H), 7.07–7.08 (m, 10H), 7.22 (d, J = 7.5 Hz, 2H), 10.27 (br s, 1H); 13C NMR (125 MHz, CDCl3): δ = 14.8, 21.0, 21.1, 33.7, 54.8, 57.9, 59.8, 98.5, 113.5, 116.0, 125.3, 125.8, 126.4,126.8, 128.7, 128.8, 128.9, 129.3, 135.8, 136.6, 138.2, 139.8, 142.1, 147.1, 156.3, 168.3.
4.1.2 Methyl 2,6-bis(4- chlorophenyl)-1-(4-bromophenyl)-4-(4-bromophenylamino)-1,2,5,6-tetrahydropyridine-3-carboxylate (4h)
White crystals; mp 190–192 °C. IR (KBr): 3246, 3075, 2850, 2960, 1653, 1589, 1489 cm−1; 1H NMR (500 MHz, CDCl3): δ = 2.68 (dd, J = 15.0, 1.9 Hz, 1H), 2.81 (dd, J = 15.2, 5.6 Hz, 1H), 3.95 (s, 3H), 5.05 (bs, 1H), 6.27–6.36 (m, 5H), 6.98–7.04 (m, 4H), 7.10–7.28 (m, 4H), 7.39–7.44 (m, 4H), 10.21 (bs, 1H, NH) ppm; 13C NMR (125 MHz, CDCl3): δ = 33.9, 51.6, 55.3, 56.9, 98.5, 114.4, 120.8, 121.7, 122.6, 127.4, 128.4, 128.9, 129.4, 129.6, 132.1, 132.3, 132.5, 136.5, 141.5, 142.4, 145.2, 155.4. 168.4.
4.1.3 Methyl 2,6-bis(4-chlorophenyl)-1-(4-methoxyphenyl)-4-((4-methoxyphenyl)amino)-1,2,5,6-tetrahydropyridine-3-carboxylate (4i)
White crystals; mp 213–215 °C. IR (KBr): 3242, 3024, 2974, 2915, 2870, 1653, 1589, 1514 cm−1; 1H NMR (500 MHz, CDCl3): δ = 2.60 (dd, J = 15.0, 2.4 Hz, 1H), 2.73 (dd, J = 15.5, 5.5 Hz, 1H), 3.70 (s, 3H), 3.77 (s, 3H), 3.90 (s, 3H), 4.95 (br s, 1H), 6.21 (s, 1H), 6.32 (d, J = 8.0 Hz, 2H, 6.40 (d, J = 8.5 Hz, 2H), 6.65 (t, J = 9.0 Hz, 4H), 7.06 (d, J = 8.0 Hz, 2H), 7.22–7.20 (m, 6H), 10.15 (br s, 1H); 13C NMR (125 MHz, CDCl3) δ = 33.4, 50.5, 55.1, 55.5, 55.7,57.5, 95.5, 114.1, 115.1, 127.8, 127.9, 128.2, 128.4, 128.9, 131.1, 132.1, 132.8, 141.2, 141.6, 143.1, 152.1, 156.7, 159.0, 168.5.
4.1.4 Ethyl 1-phenyl-4-(phenylamino)-2,6-di-p-tolyl-1,2,5,6-tetrahydropyridine-3-carboxylate (4k)
Yellow solid; mp: 231–232 °C. IR (KBr): 3440, 2980, 1869, 1650, 1593, 1500, 1448, 1371, 1327 cm−1; 1HNMR (500 MHz, CDCl3): δ = 1.46 (t, J = 7.0 Hz, 3H), 2.32 (s, 3H), 2.33 (s, 3H), 2.76 (d, J = 15.5 Hz, 1H), 2.85 (dd, J = 15.0, 5.5 Hz, 1H), 4.32–4.44 (m, 2H), 5.11 (s, 1H), 6.29 (d, J = 6.5 Hz, 2H), 6.40 (s, 2H), 6.53 (d, J = 8.0 Hz, 2H), 6.59 (t, J = 6.5 Hz, 1H), 7.07–7.08 (m, 10H), 7.21 (d, J = 7.5 Hz, 2H), 10.28 (br s, 1H); 13C NMR (125 MHz, CDCl3): δ = 14.6, 21.1, 21.3, 34.1, 54.9, 57.9, 59.5, 98.5, 113.1, 116.1, 125.3, 125.7, 126.4, 126.7, 128.8, 128.9, 129.1, 129.3, 135.8, 136.7, 138.2, 139.8, 142.1, 147.1, 155.7, 168.3.
4.1.5 Ethyl 2,6-bis(4-nitrophenyl)-1-phenyl-4-(phenylamino)-1,2,5,6-tetrahydropyridine-3-carboxylate (4n)
Yellow solid; mp: 247–249 °C. IR (KBr): 3440, 2980, 1650, 1595, 1520, 1434, 1382 cm−1; 1HNMR (500 MHz, CDCl3): δ = 1.25 (t, J = 7.5 Hz, 3H), 2.45 (dd, J = 15.5, 3.0 Hz, 1H), 2.84 (dd, J = 15.5, 3.0 Hz, 1H), 4.14–4.19 (m, 1H), 4.22–4.29 (m, 1H), 4.68 (J = 12.0, 3.0 Hz, 1H), 6.01 (s, 1H), 6.76 (d, J = 8.5 Hz, 2H), 6.84 (t, J = 8.5 Hz, 1H), 7.00 (d, J = 8.0 Hz, 2H), 7.14 (d, J = 7.5 Hz, 2H), 7.18 (t, J = 7.5 Hz, 1H), 7.32–7.73 (m, 6H), 8.07 (d, J = 8.5 Hz, 2H), 8.24 (d, J = 8.5 Hz, 2H), 10.60 (br s, 1H); 13C NMR (125 MHz, CDCl3): δ = 14.5, 36.3, 60.2, 61.1, 62.4, 96.5, 118.1, 120.9, 123.6, 124.2, 125.5, 127.8, 128.4, 129.2, 129.5, 136.2, 147.1, 147.2, 149.7, 150.2, 153.9, 156.3, 168.1 Table 2.
Ce(OTf)4-catalyzed synthesis of functionalized tetrahydropyridines.
Entry | R1 | R2 | R3 | Product | Yield (%) | Melting point (°C) | |
Found | Reported | ||||||
1 | H | Et | C6H5 | 4a | 85 | 173–174 | 174–176 [10] |
2 | H | Et | 4-Cl-C6H4 | 4b | 80 | 199–201 | 200–202 [4] |
3 | H | Et | 4-Me-C6H4 | 4c | 90 | 198–200 | 196–198 [4] |
4 | H | Et | 4-OMe-C6H4 | 4d | 90 | 170–172 | 172–174 [8] |
5 | H | Me | C6H5 | 4e | 88 | 193–195 | 194 [4] |
6 | H | Me | 4-F-C6H4 | 4f | 75 | 175–177 | 172–175 [8] |
7 | 4-Cl | Et | C6H5 | 4g | 90 | 225–227 | 226 [10] |
8 | 4-Cl | Me | 4-Br-C6H4 | 4h | 88 | 190–192 | 190–192 [8] |
9 | 4-Cl | Me | 4-OMe-C6H4 | 4i | 90 | 214–215 | 214 [13] |
10 | 4-Me | Et | 4-OMe-C6H4 | 4j | 90 | 219–221 | 220–222 [13] |
11 | 4-Me | Et | C6H5 | 4k | 88 | 231–232 | 232–234 [13] |
12 | 4-Me | Me | 4-Cl-C6H4 | 4l | 85 | 216–218 | 218–220 [8] |
13 | 4-F | Et | C6H5 | 4m | 85 | 203–204 | 204–208 [13] |
14 | 4-NO2 | Et | C6H5 | 4n | 80 | 247–249 | 247–250 [13] |
15 | 4-Me | Et | PhCH2 | 4o | 70 | 171–174 | 173–175 [13] |
Acknowledgment
This research is supported by the Islamic Azad University, Ayatollah Amoli Branch.