1 Introduction
The recent development of membrane technologies has aroused a considerable increasing interest concerning polymer inclusion membrane (PIM) and their applications in different systems containing a mixture of metallic ions in complexing aqueous media.
These materials have been introduced to perform some transport properties and to increase stability compared to liquid membrane systems. In order to reduce the amounts of reactants and energy needed for separation and to decrease the environmental and economic impact of LLX separations, several membrane-based separation techniques have been proposed in the past two decades [1–5]. Supported liquid membranes (SLM) have been extensively studied since they offer high transport rates and good selectivity, therefore, being a very interesting option to overcome the LLX downsides [6–9]. Effort has been directed towards the development of such membranes and some promising materials and designs have been described. Facilitated transport was first accomplished in liquid membranes, in which two aqueous phases were separated by an organic solvent containing carrier molecules, such as crown ethers. An exhaustive review of cation transport in liquid membranes has been written by McBride et al. [10]. Diffusion of metal ions in liquid membranes is governed by complex formation with the carrier at the aqueous/organic interface so that selectivities are generally very high, whereas the fluxes in these membranes are very low because they are limited by the convection of the carrier in the organic phase. The biggest disadvantages using liquid membranes are the low fluxes, the leaching of carriers and poor physical stability. Metal ion separations using new plasticized cellulose triacetate (CTA), called a PIM, are currently receiving considerable attention [11–16]. A comparison between SLM and PIM performance has been reported by Ulewicz and Radzyminska-Lenarcik [12], where they show that Cu2+ can be separated very effectively from other transition metal cations as Zn2+, Co2+, and Ni2+ from different equimolar mixtures of these ions using 1-hexyl-2-methylimidazole as the ion carrier. Also, the stability of PIM and SLM doped with 1-hexyl-2-methylimidazole was confirmed in replicate experiments.
We found that PIM fluxes were four orders of magnitude larger than those exhibited by the SLM. PIM systems have been successfully designed for metal extraction using solvating-type extractants, such as crown ethers, TOPO and TBP [17–19].
The first approach combining facilitated carriers or chelating capture of ions have been realized by the use of crown ether noted hexaoxa diazabicyclo hexacosane (2 2 2) and polyethyleneimine. Enhanced selectivity toward copper ion has been observed [20]. This PIM system with complexing properties has been examined in our previous works [21], poly-4-vinylpyridine has been incorporated in TCA–TEHP membrane, giving rise to the separation of ions according to their hydrophilic and hydrophobic balance and the protonation ratio of the nitrogen group of polymer. This concept has been extensively developed by our group when polyethyleneimine was used as the chelating agent at the interface of membrane/aqueous solution. This polymer has been chosen since it exhibits an interesting change in the conformation according to pH and concentration in solutions; this change is strongly dependent on the medium's pH and concentration, which cause interaction between amine and ammonium groups of partially protonated sites [22–24].
For applications in the field of ion separation, glass polymers with large free volumes and the development of PIMs for industrial separation (hydrometallurgy) and clean up and recycling of wastewaters are particularly promising.
It should be emphasized that all the research on PIMs carried out so far has been conducted on a laboratory scale and the transition of this research to a pilot or full-scale application presents a major challenge for the future. In general, PIMs are highly resistant to carrier and plasticizer leakage. This has probably prompted a recent investigation focusing instead on the hydrolysis of base polymers under extreme conditions. To date, all the research on PIMs has been conducted on a laboratory scale.
One of our objectives is the manufacturing of membranes with very high ion selectivity. Our aim consists of establishing these processes in industrial applications in close cooperation with other partners.
In this work, a plasticized CTA membrane containing polyethyleneimine (PEI) and polyethyleneglycole (PEG) has been elaborated. The polyelectrolyte + plasticizer membrane was characterized using chemical techniques as well as Fourier transform infra-red (FTIR), thermogravimetric analysis (TGA) and scanning electron microscopy (SEM). As an application, the separation of bivalents cations (Cu2+, Cd2+, Zn2+) and monovalent cations (K+ and Na+) using PIMs is achieved.
2 Experimental
2.1 Reagents and apparatus
Metal cation [Cd(II), Cu(II), Zn(II), K(I) and Na(I)] aqueous solutions were prepared by dilution of stock solutions of the corresponding metal nitrate (0.01 M) with distilled water. CTA (Aldrich), chloroform (Aldrich) and tris(2-ethylhexyl-phosphate) (TEHP) (Merck, 99%), PEI and PEG are Merck (high-purity) products, and were used as received without further purification.
2.2 Membranes preparation
PIMs are prepared using the procedure described by Sugiura et al. [25]. Briefly, 0.4 g of CTA and 0.1 g of each polyelectrolyte (PEI and PEG) are dissolved in 40 mL of chloroform and stirred for 4 h. Then, 0.2 mL of TEHP are added and maintained for 2 h under vigorous stirring. The solution is transferred to a circular glass container and let for a slow evaporation during 24 h. The resulting membrane is extracted by the addition of water and dried at 40 °C.
2.3 Analysis
The concentration of M2+ is determined by sampling at different time intervals; aliquots (0.5 mL) from both the feed and strip solutions are analyzed with an atomic absorption spectrometer (Perkin-Elmer 2380). The mass flux J (mol cm2 s−1) of M2+ through the PIM transferred from the feed side to the strip one is determined by applying the definition: J = An/SAt, where An represents the variation in the mole number of M2+ in the receiving solution during the reference time At, and S is the active surface of the membrane. FTIR spectra are recorded with a Perkin-Elmer spectrometer (Spectrum One). X-ray diffractions are determined with a Bruker D8 diffractometer using the monochromatized Cu Kα radiation. TGA experiments are done using a TA Instrument thermogravimeter at a heating rate of 10 °C min−1.
2.4 Transport
The cell used for transport experiments consisted of two compartments, made of Teflon with a maximum filling volume of 50 mL, separated by the PIM [19]. The feed compartment contains initially a mixture of metallic ions Cu2+, Cd2+, Zn2+, K+, Na+ at a concentration of 10−2 M of metal salt; the other compartment noted strip contains distilled water. The two compartments have the same volume (50 mL). Each compartment was provided with a vertical mechanical stirrer at stirring speed 800 rpm, which was previously determined as high enough to minimize the thickness of the boundary layer. The experiments began when starting the stirring motors in the two compartments of the cell. The exposed membrane area was 9.61 cm2. All the experiments were performed in a thermostat at 25 °C.
Transport of metallic ions obeys a facilitated transport. This facilitated transport is obtained both by the presence of PEI as a complexant agent and TEHP as the plasticized and carrier. The role of TEHP as a carrier is inhibited in such membrane due to the formation of a stable complex. The metal ion is complexed at the interface feed-phase/membrane and the complex formed diffuses through the membrane phase to the interface membrane/strip phase where the decomplexation of the metal ion is realized under the condition of the presence of an extractant agent in the strip compartment. In our cases, the use of pure water did not allow the extraction of metal ions from the PEI complex. Moreover, monovalent ions (Na+ and K+) are well transported toward the membrane because no complex formation was observed. Furthermore, it is well known that the solubility of alkaline metals in the water is higher than in the middle containing TEHP. Note that the equilibrium is reached when a chemical potential gradient (the driving force of the process) is established.
3 Results and discussion
3.1 FTIR
The membranes CTA + polyelectrolyte + plasticizer + carrier are characterized by FTIR. Fig. 1a shows the spectra of (CTA + PEI + PEG + TEHP) membrane whose main features are the absorption band located around 1747 cm−1, attributed to stretching vibrations of the carbonyl group. The bands centered at 1242 and 1035 cm−1 correspond to the stretching modes of C–O single bonds. Less intense bands at 2930 and 2880 cm−1 are attributed to C–H bonds while the wide band in the region 3463 cm−1 is assigned to O–H bond stretching modes. The main functions susceptible to develop specific interactions of hydrogen bonding type are the hydroxyl and the carbonyl ones. Fig. 1b shows the spectrum of CTA, PEI, PEG and TEHP.
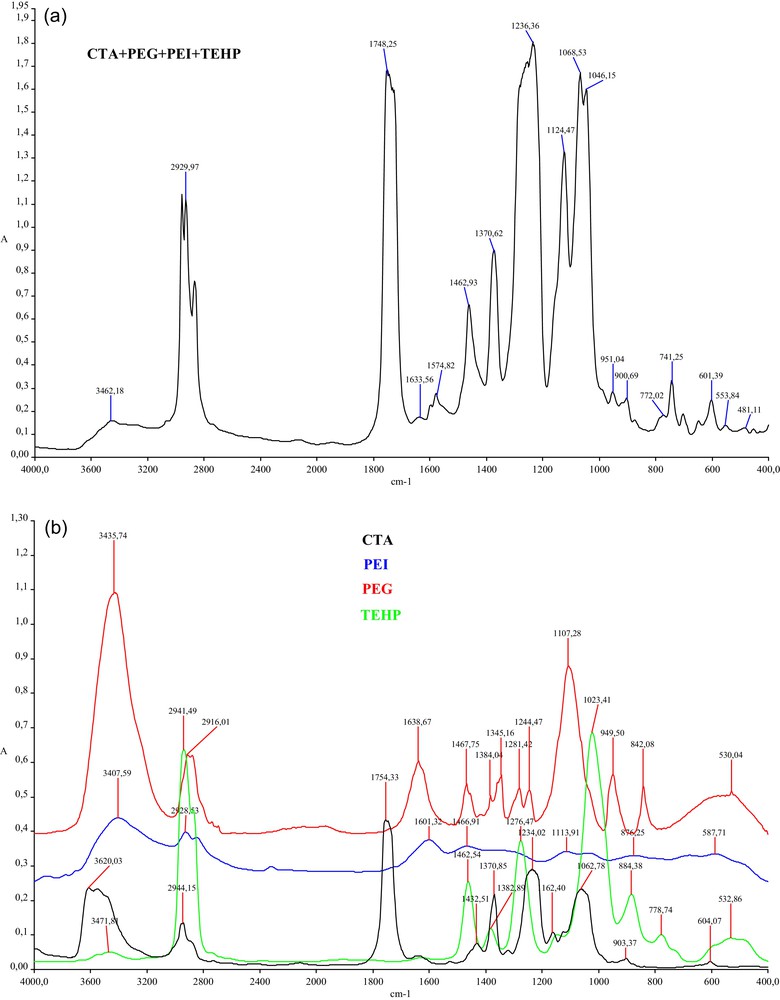
FTIR Spectrum of (CTA + PEG + PEI + TEHP) membrane.
Table 1 collects the peak values and the corresponding radicals of CTA, PEI, PEG, TEHP and (CTA + PEI + PEG + TEHP) membrane.
Peak values and the corresponding radicals of (CTA + PEG + PEI + TEHP) membrane.
Membrane or compound | Peak values (cm−1) | Corresponding radicals |
CTA membrane | 3480–3550 | OH |
1755 | CO | |
1561 | COO− | |
1246 | COC asym | |
1054 | COC sym | |
TEHP (plasticizer) | 2942 | CH |
1276 | PO | |
1020 | POC | |
PEI (liquid polymer) | 3408 | NH |
2920 | CH | |
1462 | CN | |
PEG (solid polymer) | 3435 | OH |
1244 | CO asym | |
1107 | CO sym | |
CTA + PEG + PEI + TEHP membrane | 3463 | OH (TAC and PEG) |
1748 | CO (CTA) | |
1574 | COO− (CTA) | |
1462 | CN (PEI) | |
1285 | PO (TEHP) | |
1236 | COC asym | |
1035 | COC sym |
FTIR studies confirm the presence of plasticizer (TEHP), PEG and PEI in the polymer matrix (CTA) and reveal no signs of covalent bond formation between the polyelectrolyte, the plasticizer and the base membrane skeleton; it is most likely that they are bound to one another by a form of secondary bonding, such as hydrophobic, van der Waals or hydrogen bonds. Further efforts will be directed to the determination of the nature of the interactions between polymer, plasticizer and carrier by the use of other materials and analysis as well.
3.2 Characterisation by SEM
One important aspect of PIMs is the microstructure of the membrane materials, which determines the distribution of polyelectrolytes in the polymer matrix and ultimately affects the membrane transport efficiency.
SEM provides excellent qualitative information (dense or porous membranes) and quantitative capability in measuring important subsurface features, such as porosity and layer thickness.
The morphologies of (CTA + polyelectrolyte + plasticizer) membrane (Fig. 2a: surface, Fig. 2b: cross section) clearly confirm their dense nature. The observations show the existence of two phases represented by white (a mixture of PEI, PEG and TEHP) and grey (CTA) contrast, respectively, the pores are filled by the polyelectrolyte and a plasticizer molecule decomposes, yielding a thick and less membrane. This explanation is based on our previous work [26].
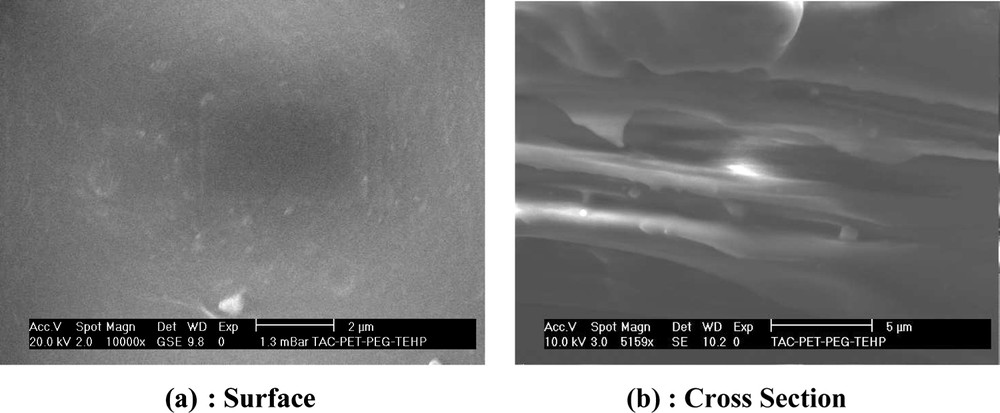
SEM images of (CTA + PEG + PEI + TEHP) membrane.
3.3 Characterisation by TGA
Fig. 3 shows the thermal behavior of (CTA + PEG + PEI + TEHP) membrane, which decomposes in a two-step process. The first mass loss (∼ 80%) occurring at 250–300 °C represents the main thermal degradation of the polymeric chains. The second one starting at 350 °C is due to the complete carbonization of the products to ash. This result confirms that the synthesized membrane exhibits a good thermal stability.
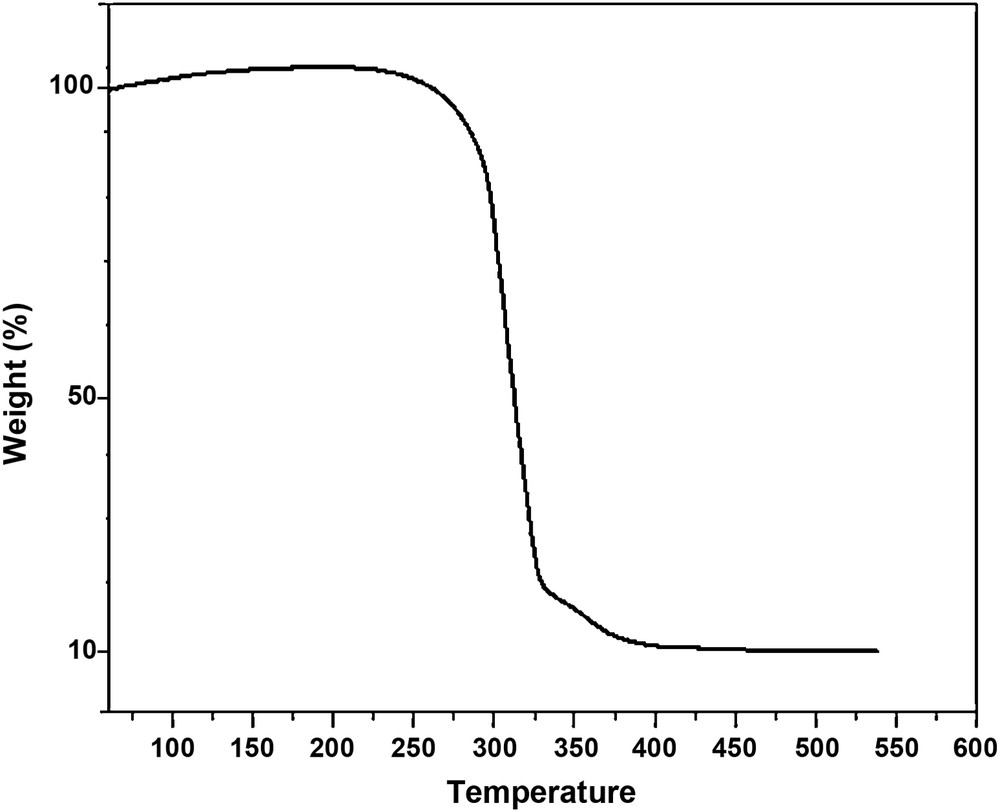
TGA thermogram of (TAC + PEI + PEG + TEHP) membrane.
It must be noted that the boiling point of a plasticizer (TEHP) is 220 °C, meaning that TEHP has probably interacted with one of the three polymers (CTA, PEI or PEG).
3.4 Characterisation by X-ray diffraction
XRD was used to determine the mechanism of transfer of the ions between feed and strip phases. Two of the most likely mechanistic possibilities are complex-diffusion (amorphous structure) and fixed-site jumping (crystalline structure). With this latter mechanism, the solute moves through the membrane by jumping from one fixed-site to another. The theory of fixed-site jumping has been described by Cussler et al. [27] and Noble [28].
The X-ray spectrum of the CTA + PEG + PEI + TEHP membrane has been investigated (Fig. 4). The shape of the spectra is classical for a polymer constituted of a crystalline part and a vitreous one. The ratio of the areas of the main peaks to that of the unresolved background gives an approximate value of the percentage of both structures of the polymeric membrane. When the plasticizer is added to the CTA + PEG + PEI, the crystalline part of the three polymers is not very affected, only the diffraction of the amorphous part is changed [20].
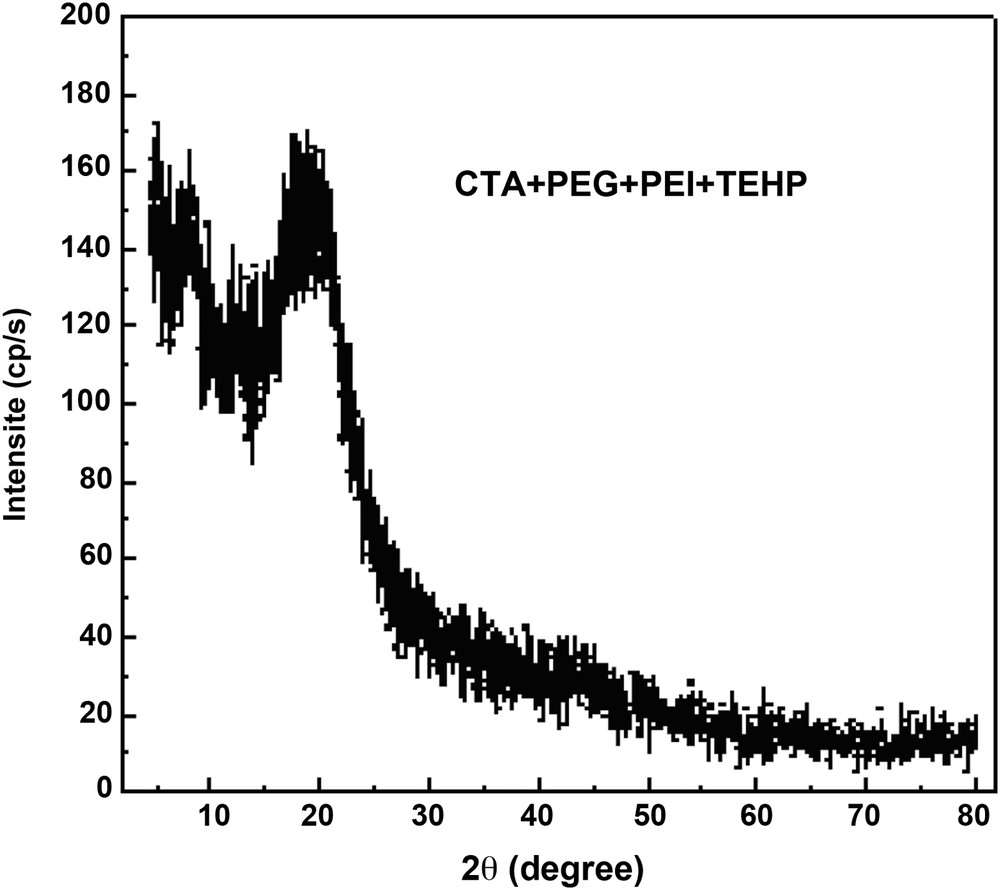
X-ray curve for (CTA + PEG + PEI + TEHP) membrane.
3.5 Transport of metallic ions
3.5.1 Effect of the initial concentration of metal in a feed-phase
Fig. 5 represents the variation of Ni(II), Zn(II) and Cu(II) ions transferred percentages versus the initial concentration of the three ions.
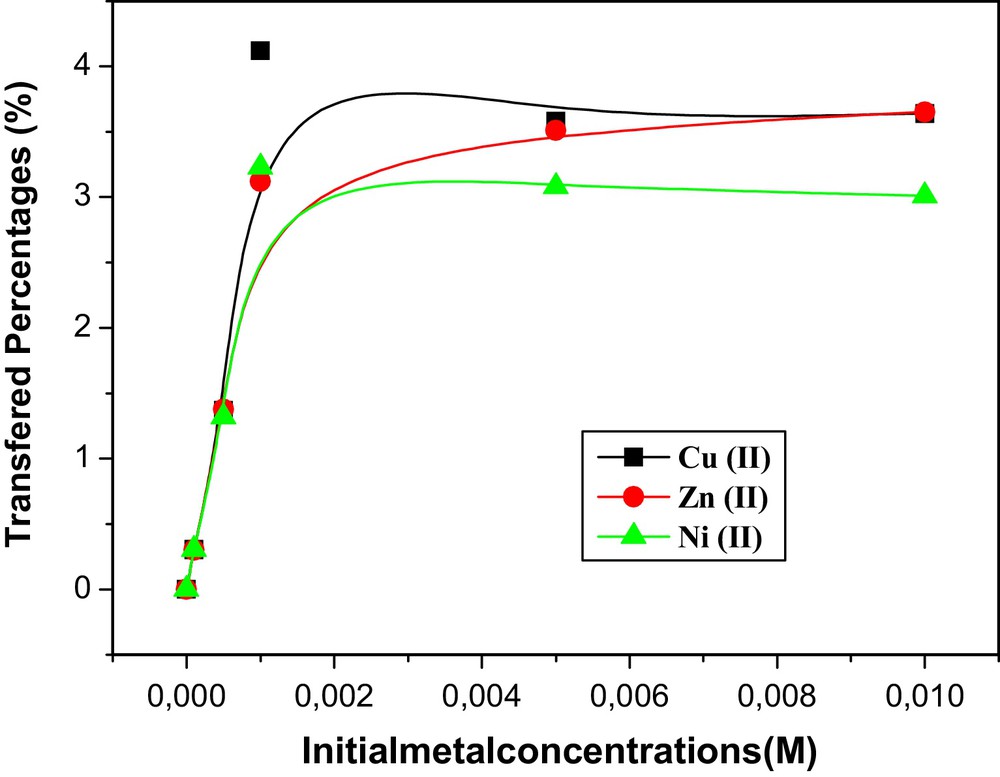
Evolution of the transferred percentages of Ni(II), Zn(II) and Cu(II) in a strip phase versus the initial concentration of metallic ions in the feed compartment using CTA + PEG + PEI + TEHP) membrane. Experiment duration: 4 h.
The results show that the transport percentages are a function of the feed initial concentration (transport percentages of metal ions increase sharply with increasing the initial concentration of each metal in the source phase). However, beyond 10−3 M, the transport percentages tend to have maximal value and become independent of M2+ concentration. This is due to the saturation of the membrane by the metal-carrier complex molecules and the decomplexation at the strip interface is presumably the rate-limiting step in such process. Fujimori [29] revealed the existence of copper and nickel complex with PEI in the stoichiometry 3.2 and 3.7 for PEI:Cu and PEI:Ni, respectively. This is due to the geometry of complex (square planar in the case of copper and octahedral for nickel). These complexes are more stable than PEI:Zn. In Table 2 are given the overall formation constants of the three complexes [30]. As it can be observed, the stabilities are involved in the order: PEI:Cu > PEI:Ni > PEI:Zn.
Overall stability constant for the PEI-metal complex.
PEI:Cu | PEI:Ni | PEI:Zn | |
logK | 11.1 | 10.8 | 10.2 |
3.6 Quantities of metallic ions transferred using dialysis
Fig. 6a and b present respectively the evolution of the percentages of heavy and alkaline metals transferred to the strip compartment as a function of time using fixed-site membranes (CTA + PEG + PEI + TEHP). The saturation of the concentration of nickel, zinc and copper ions in the strip compartment with the time for the CTA-polyelectrolyte-plasticizer membrane indicates that transport is very low (only 5% of the ion transfer). This result confirms the formation of metal ion/PEI complexes in the membrane.
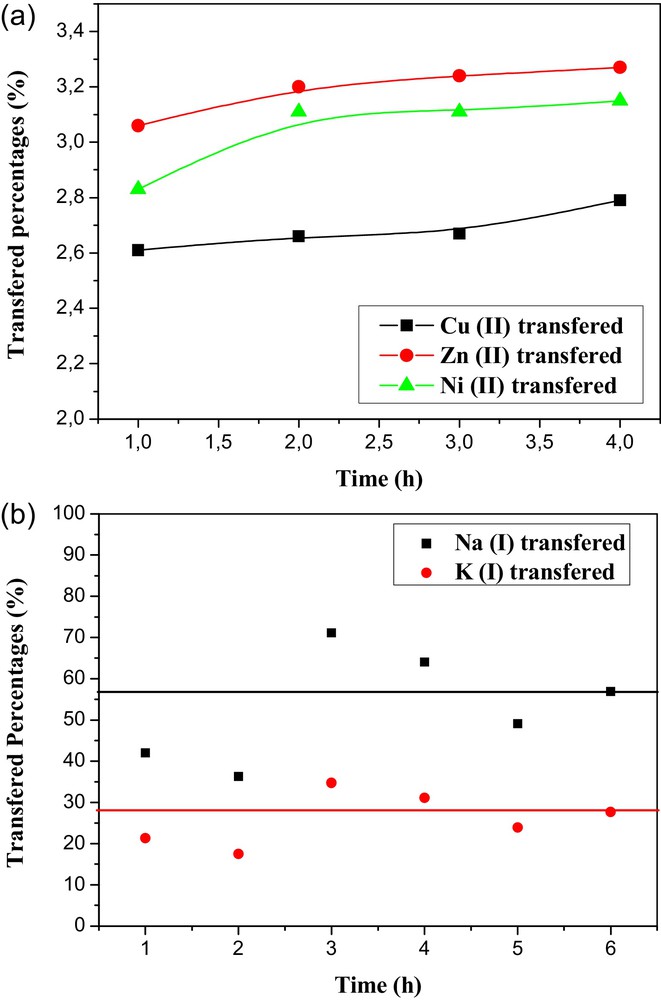
(a): Evolution of the transferred percentages of Ni(II), Zn(II) and Cu(II) in a strip phase versus time using (CTA + PEG + PEI + TEHP) membrane; (b): evolution of the transferred percentages of Na(I) and K(I) in a strip phase versus time using (CTA + PEG + PEI + TEHP) membrane.
3.7 Quantities of metallic ions fixed in the membrane
The effect of the quantity of metallic ions fixed in the membrane has been studied. Fig. 7a and b represent respectively the variation of the percentages of bivalents and monovalents ions fixed in the membrane versus transport time. These figures show that the concentration of each metallic ion increases considerably with time.
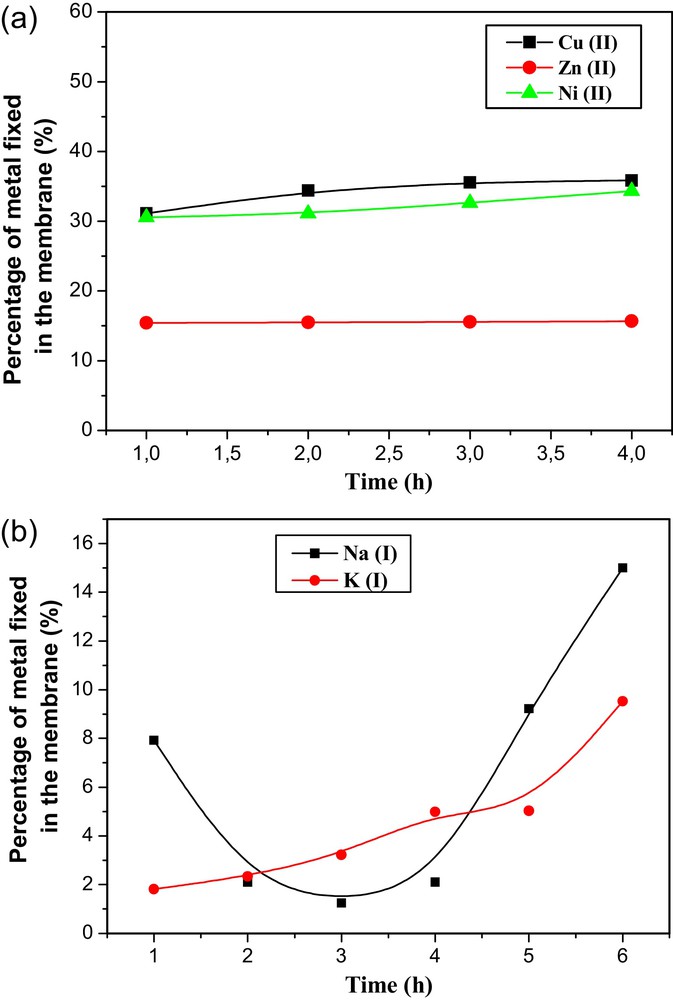
(a): Evolution of the percentages of Ni(II), Zn(II) and Cu(II) fixed in (CTA + PEG + PEI + TEHP) membrane versus time; (b): evolution of the percentages of Na(I) and K(I) fixed in (CTA + PEG + PEI + TEHP) membrane versus time.
The main results reveal that copper(II), nickel(II) and zinc(II) were predominately more retained by the modified membrane (containing PEI polyelectrolyte). It may be seen that the copper(II) ion is mainly adsorbed by the PEI, the final solution contains very small amounts of metallic ions.
Polyethyleneimine is known as a suitable polyelectrolyte in aqueous solutions having chelating properties as well as good solubility and different conformation depending on the viscosity of the medium. Chelating properties have been studied elsewhere [30,31]; it has been found using both potentiometric and spectrophotometric methods that PEI/Cu(II) ability complexes using branched form was ten times more important than the linear form. Also, the stability constants obtained for PEI/Cu complexes were higher than those obtained for other heavy metals, such as Cd and Zn.
4 Conclusion
A mixture of CTA and polyelectrolytes membrane containing TEHP as plasticizer is successfully synthesized. These polymers + plasticizer membranes are characterized using physical methods like FTIR, X-Ray diffraction, thermal analysis and scanning electron microscopy. The membranes are constituted by the mixture of CTA + polyelectrolyte + plasticizer with amorphous structure with no diffraction peak. It can be due to the absence of crystallization within the membrane. The degradation of the membranes occurs via a two-step process whose main loss starts at 200 °C, which is due to the thermal degradation of the polymeric chains. This result confirms that all the synthesized membranes exhibit a good thermal stability. The SEM observation shows that the membranes present a dense and homogeneous structure.
The optimum value and the behavior of the main parameters influencing the transport of bivalent and monovalent ions through new polymeric membranes were determined. In transport experiments, the transmembrane concentration increased with increasing the initial metal concentration, reaching a limiting value when the concentration was greater than 10−3 M. The transport efficiency obtained with bivalents cations was slightly lower than those with monovalents cations. A lower diffusivity of (PEI/M2+) complexes with respect to (PEI/M+) complexes for all ions and a sequence of diffusivity D(K+) > D(Na+) > D(Zn2+) > D(Ni2+) > D(Cu2+) was obtained. The PEI-modified membrane has a preferential selectivity towards heavy metals and particularly the copper(II) ion.
Modified membrane were used in successive retentions and allowed the separation of transition metals from alkaline ones, or the separation of monovalent ions from multivalent ones or separation of less from more hydrated ions.