1 Introduction
Epoxides are highly useful intermediates to manufacture a wide range of important commercial products [1]. The catalytic epoxidation of olefins is one of the main routes, which leads to the production of epoxides on both laboratory and industrial scale [2]. Many oxidants have been used to synthesize epoxides. But there is a current intense interest in using hydrogen peroxide as an environmentally friendly oxidant that produces water as a by-product [3]. The low oxidation power of hydrogen peroxide seems to be due to the poor leaving tendency of the hydroxide ions [4,5]. An activation method, capable of modifying the orbital energy and tuning the electrophilic/nucleophilic behavior of the oxidant, is thus required. The activation of hydrogen peroxide takes place in the presence of homogeneous metal catalysts, such as iron, manganese and rhenium or through the addition of stoichiometric amounts of auxiliaries, which convert hydrogen peroxide into a more active oxidant [6]. Other methods for the activation of H2O2 are the formation of peroxyacids [7], peroxycarboximidic acids [8], or the use of sodium perborate or sodium percarbonate in basic solutions [9]. It is also possible to epoxidize a wide range of alkenes with peroxycarboxylic acids generated in situ from either carboxylic acid anhydrides or with aqueous H2O2 [10–20].
Hydrogen peroxide has been widely used as an oxidant in epoxidation reactions catalyzed by metalloporphyrins [21,22]. In addition, the use of co-catalysts significantly affects the catalyst's activity. Addition of carboxylic acids to the manganese porphyrin/hydrogen peroxide systems for alkene epoxidation gives rise to a remarkable increase in reaction rates [23]. Also, peroxyacetic acid generated from excess acetic anhydride and 30% H2O2 has been used in the Mn-porphyrin-catalyzed epoxidation of alkenes [24]. Also, many supported manganese porphyrins have been used as catalysts for the oxidation of organic compounds [25–27]. Heterogeneous catalysts tend to reduce activities compared to their truly homogeneous analogues. However, this drawback can be offset by the advantages of easy catalyst recovery.
In the present research, a metalloporphyrin was immobilized onto the surface of functionalized multi-wall carbon nanotubes and used as a catalyst for alkene oxidation by hydrogen peroxide and acetic anhydrides as activators under ultrasonic irradiation conditions.
2 Experimental
2.1 Instruments and reagents
Chemicals were purchased from Merck or Fluka chemical companies. Meso-tetrakis(4-hydroxyphenyl)porphyrin, (H2THPP), was prepared according to the literature [28]1. [Mn(THPP)OAc] and [Mn(THPP)OAc@MWCNT] were prepared and characterized according to the already reported procedures [26,29]. FT–IR spectra were obtained on an ABB Bomem: FTLA 2000–100 in the 400–4000 cm−1 range using spectral grade potassium bromide. Microstructure observations were conducted with a scanning electron microscope (SEM). Gas chromatography (GC) analyses were conducted on a Shimadzu chromatograph (model GC-14B) equipped with a flame ionization detector (FID) and a capillary column SAB-5 (phenyl methyl siloxane 30 m × 320 mm × 0.25 mm). In the GC experiments, n-octane was used as an internal standard. The reactions were submitted to ultrasonic irradiation at 296 W (WUC-A03H, DAIHAN). The temperature reached to 30 °C during sonication.
2.2 General heterogeneous oxidation procedure
Catalytic experiments were carried out in a 5-mL test tube. In a typical procedure, to a cyclooctene solution in ethanol (0.05 mmol) were added 1.25 μmol of catalyst, 0.12 mmol of imidazole, 0.2 mL of H2O2 (30%) and 0.2 mL of acetic anhydride. The reaction mixture was maintained under ultrasonic irradiation for 30 min. Then, the reaction products were monitored at periodic time intervals using gas chromatography. The oxidation products were identified by comparison with authentic samples (retention times in GC).
2.3 Catalyst reuse and stability
The reusability of [Mn(THPP)OAc@MWCNT] was investigated in the multiple sequential epoxidation of cyclooctene, as described above. At the end of each reaction, the catalyst was separated from the reaction mixture by simple filtration. After isolation, the solid catalyst was washed with ethanol, separated from the solvent as described above and dried in air before being used in the next run.
3 Results and discussion
3.1 Preparation and characterization of the catalyst, [Mn(THPP)OAc@MWCNT]
The specification of multi-wall carbon nanotubes containing carboxylic acid groups (MWCNT–COOH), which was used as the support, is listed in Table 1. Scheme 1 shows the preparation route for [Mn(THPP)OAc@MWCNT]. The covalent bonding between the metalloporphyrin and the nanotubes was carried out by ester bond formation between the porphyrin hydroxyl groups and the carboxylic acid of the nanotube using TBTU (2-(1H-benzotriazole-1-yl)-1,1,3,3-tetramethyluronium tetrafluoroborate) as a highly effective uronium salt that has been used as an activation agent for carboxylic acids for the preparation of esters [30,31] in the presence of DIPEA (N,N′-diisopropylamine) [32].
The specification of the MWCNT-COOH used in this study.
Outside diameter | Inside diameter | Length | COOH content | Specific surface area |
10–20 nm | 5–10 nm | ∼ 30 μm | 2% | >200 m2/g |
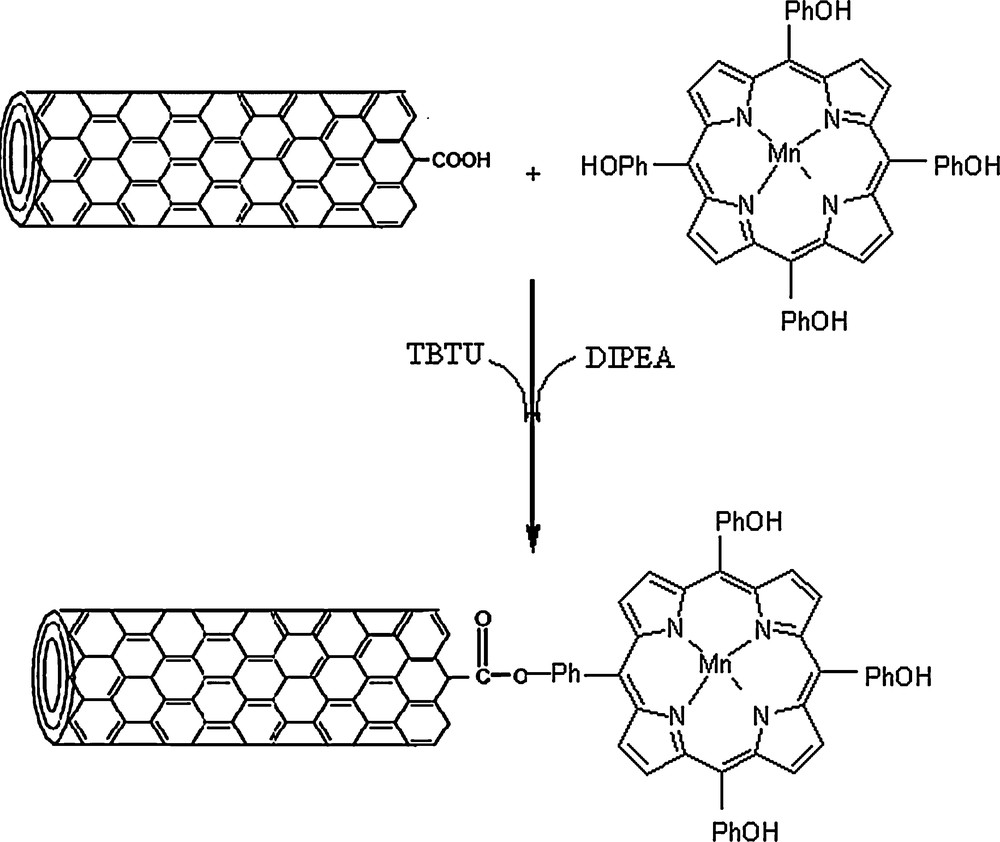
Preparation of [Mn(THPP)OAc@MWCNT].
The prepared catalyst was characterized by elemental analysis, FT–IR spectroscopy and scanning electron microscopy (SEM). The nitrogen content of the catalyst was determined by CHN analysis, which showed a value of 0.8% for the Mn catalyst. Based on this value, the manganese porphyrin content of the catalyst obtained was about 357 μmol per gram of the catalyst. The Mn content of the catalyst was also measured by atomic absorption spectroscopy; the results proved comparable to data obtained by CHN analysis.
The most informative spectroscopic data, which confirmed the covalent anchoring of Mn(THPP)OAc on the functionalized MWCNT, were obtained by comparison of the IR spectra of MWCNT–COOH and [Mn(THPP)OAc@MWCNT]. The CO stretching band of the carboxylic acid group of MWCNT–COOH appeared at 1642 cm−1. When porphyrin was attached to MWCNT–COOH, an ester vibration was observed at 1709 cm−1. These observations clearly confirmed the attachment of the manganese porphyrin to the MWCNT.
The scanning electron micrograph of MWCNT–COOH (Fig. 1a) shows the morphology of the MWCNT used in this study. Comparison of SEM images of [Mn(THPP)OAc@MWCNT] (Fig. 1b) and of the carbon nanotube clearly indicates that the manganese porphyrin has been supported on the nanotubes.
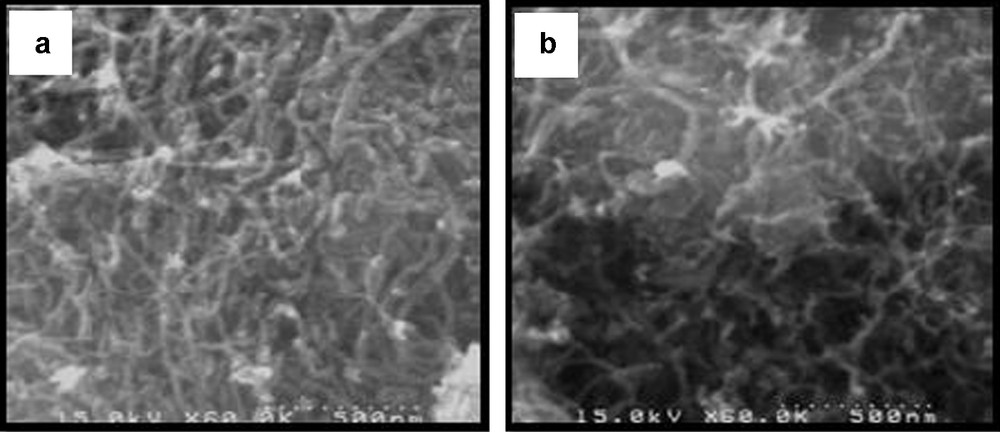
The SEM (a) image of MWCNT (b) image of [Mn(THPP)OAc@MWCNT].
3.2 Catalytic oxidation of cyclooctene
The catalytic activity of the heterogeneous catalyst was investigated in the oxidation of cyclooctene with hydrogen peroxide and acetic acid or acetic anhydride as an activator (Table 2). The results show that in the absence of an activator, the reaction proceeds with only 3% conversion, while in the presence of acetic acid or acetic anhydride, 25% or 100% of cyclooctene oxide were obtained, respectively.
Epoxidation of cyclooctene catalyzed by [Mn(THPP)OAc@MWCNT] with H2O2 in ethanol in the presence of different activators under ultrasonic irradiation a,b.
Entry | Additive | Conversion (%) | Selectivity (%) (Epoxide) |
1 | None | 3 | 100 |
2 | Acetic acid | 25 | 100 |
3 | Acetic anhydride | 100 | 100 |
a Reaction conditions: the molar ratio for catalyst:ImH:cyclooctene:additive:H2O2 (30%) are 1:100:40:X:1600.
b Reaction time: 30 min.
The effect of the amount of acetic anhydride on the epoxidation of cyclooctene was studied (Table 3). The results showed that the reaction needs an equimolar amount of acetic anhydride with respect to the hydrogen peroxide for completion.
The effect of acetic anhydride amounts on the epoxidation of cyclooctene catalyzed by [Mn(THPP)OAc@MWCNT] with H2O2 in ethanol under ultrasonic irradiation a,b.
Entry | Acetic anhydride (mL, mmol) | H2O2/Anhydride | Conversion (%) |
1 | 0 | – | 3 |
2 | 0.1 mL, 1 mmol | 2 | 76 |
3 | 0.2 mL, 2 mmol | 1 | 100 |
4 | 0.4 mL, 4 mmol | 0.5 | 27 |
a Reaction conditions: the molar ratio for catalyst:ImH:cyclooctene:acetic anhydride:H2O2 (30%) are: 1:100:40:X:1600.
b Reaction time: 30 min.
The epoxidation of cyclooctene catalyzed by [Mn(THPP)OAc@MWCNT] with H2O2 in ethanol under mechanical stirring (MS) and ultrasonic irradiation (US) has been carried out (Fig. 2). Running the oxidation of cyclooctene in the absence of ultrasound and by using magnetic stirring led to a conversion of 36%. On the other hand, catalyst pre-treatment with ultrasounds enhanced the conversion to 51%, but the continuous use of ultrasounds gave a conversion of 100%. Therefore, the ultrasounds are suggested to be a promising alternative to magnetic stirring. Also, the structure of heterogeneous catalysts may be modified and become more dispersed due to ultrasonic vibrations.
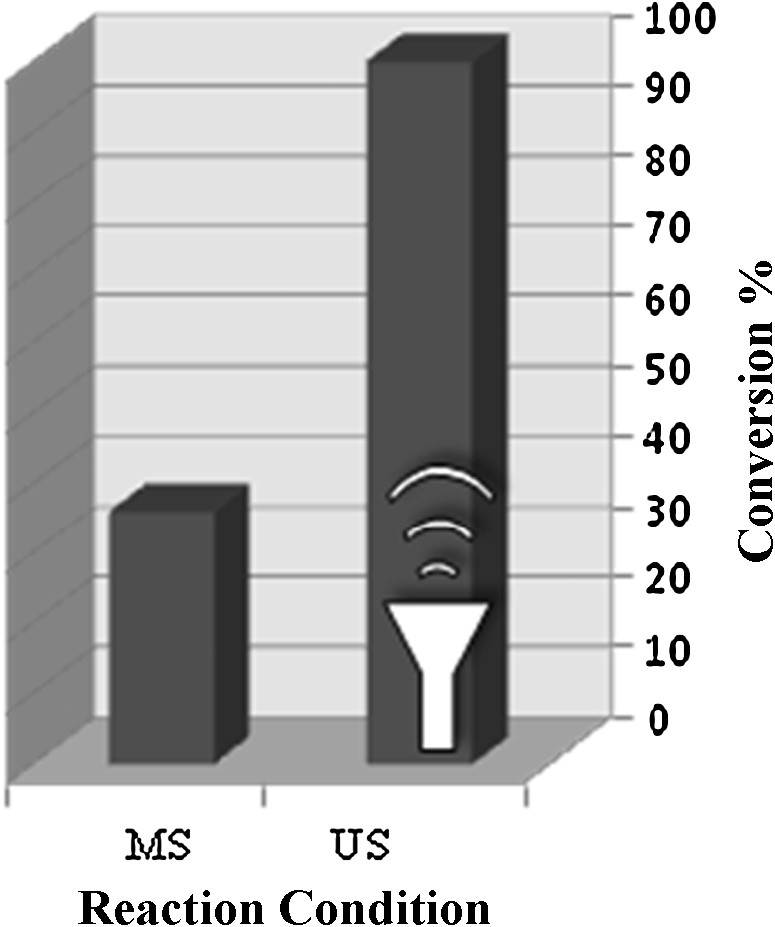
Various reaction conditions on the epoxidation of cyclooctene catalyzed by [Mn(THPP)OAc@MWCNT] with H2O2 in ethanol for a reaction time of 30 min (the molar ratio for catalyst:ImH:cyclooctene:acetic anhydride:H2O2 (30%) are: 1:100: 40:1600:1600).
The results showed that the use of US irradiation increased the conversion to 100% and reduced the reaction time to 30 min. This enhancement may be due to the production of hot spots upon sonication, and/or the breakup of agglomerates during the sonication process through mixing of the reactants [33]. The effect of ultrasounds on the catalytic activity of the title catalyst may be explained on the basis of the generation of cavitation microbubbles [34–37], and the increased catalyst disaggregation resulted by sonication of the reaction mixture.
In order to find the suitable reaction conditions, the effect of the various reaction parameters that may affect the conversion of the reaction were studied. Catalyst, oxidant and imidazole concentrations are the factors that have been evaluated.
In order to optimize the concentration of the catalyst, different amounts of catalyst were used in the epoxidation of cyclooctene. A blank experiment revealed that the presence of a catalyst is essential for an effective reaction. In the absence of catalyst (entry 1 in Table 4), the reactions do not proceed. The highest conversion was obtained with 2.6 mg of the catalyst (entry 3 in Table 4) and further increments of catalyst can slightly decrease the conversion. This may be due to the higher degradation of oxidant at higher concentrations of the catalyst [38].
Optimization of the catalyst amounts in the epoxidation of cyclooctene with H2O2 under ultrasonic irradiation a,b.
No | Catalyst amount | Conversion (%) |
1 | 0 | 12 |
2 | 1.3 mg, 0.0005 mmol | 84 |
3 | 2.6 mg, 0.0010 mmol | 100 |
4 | 3.9 mg, 0.0015 mmol | 89 |
a Reaction conditions: the molar ratio for catalyst:ImH:cyclooctene:acetic anhydride: H2O2 (30%) are X:100:40:1600:1600.
b Reaction time: 30 min.
The effect of H2O2 concentration on the oxidation of cyclooctene has also been studied (Table 5). The H2O2 concentrations used were 0, 1, 2 and 4 mmol, while the amount of cyclooctene was kept fixed. It is clear from Table 5 that the percentage of cyclooctene conversion increases with increasing the H2O2 content.
The effect of oxidant amounts on the epoxidation of cyclooctene catalyzed by [Mn(THPP)OAc@MWCNT] under ultrasonic irradiation a,b.
No | Oxidant amount | Conversion (%) |
1 | 0 | 0 |
2 | 0.1 mL, 1 mmol | 16 |
3 | 0.2 mL, 2 mmol | 100 |
4 | 0.4 mL, 4 mmol | 100 |
a Reaction conditions: the molar ratio for catalyst:ImH:cyclooctene:acetic anhydride: H2O2 (30%) are: 1:100:40:1600:X.
b Reaction time: 30 min.
3.2.1 The effect of different catalyst/imidazole (ImH) molar ratios on the epoxidation of cyclooctene
The reactivity of metalloporphyrin catalysts in the oxidation reactions can be improved by the addition of different nitrogenous bases as co-catalyst [39–41]. For a better understanding of the role of the imidazole in this catalytic system, the effect of different molar ratios of the ImH/catalyst on the epoxidation of cyclooctene was investigated. In the absence of imidazole, the reaction proceeds only in 21% yield, whereas the addition of imidazole increased the reaction conversion (Fig. 3). An increase in the ImH/catalyst molar ratio up to 100 remarkably improved the reaction rate. Beyond this ratio, a significant decrease in catalytic efficiency is observed, which may be attributed to the formation of a six-coordinate (MnTHPP(ImH)2) inactive species [42].
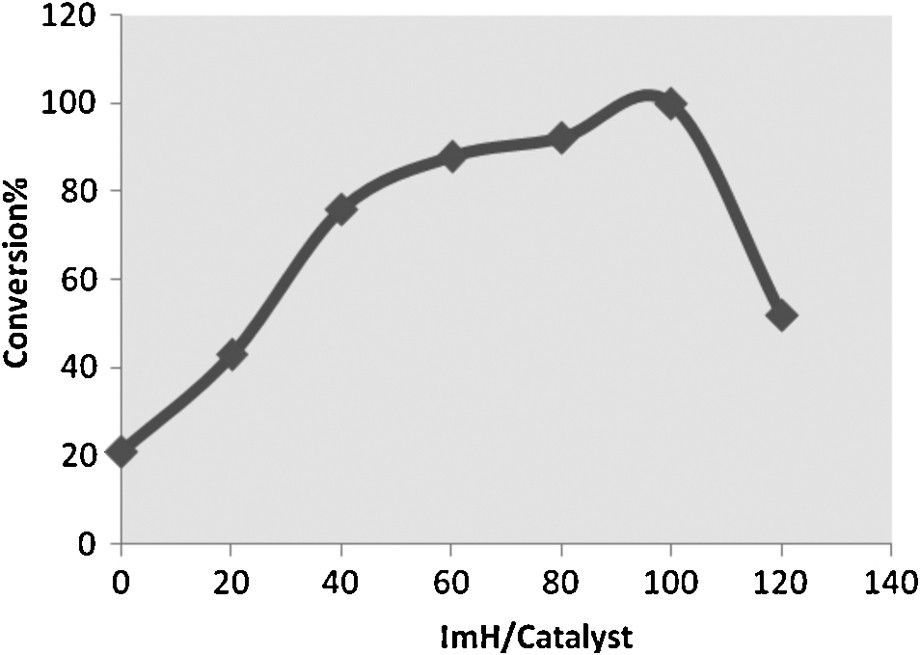
Influence of different ImH/catalyst molar ratios on the epoxidation of cyclooctene with H2O2 in ethanol under ultrasonic irradiation for a 30-min reaction time (the molar ratio catalyst:ImH:cyclooctene:acetic anhydride:H2O2 (30%) is: 1:X:40:1600:1600).
3.3 Oxidation of various alkenes with H2O2 catalyzed by [Mn(THPP)OAc@MWCNT] under ultrasonic irradiation
The [Mn(THPP)OAc@MWCNT] catalyst was used for the oxidation of different olefins with H2O2 under optimized conditions (Table 6). Cyclohexene was oxidized in 24% yield and cyclohexene oxide was the sole product. The 1-methyl-1-cyclohexene was oxidized in 32% yield, in which 1-methyl-1-cyclohexene oxide was produced as the major product in 25% yield and 2-methyl-2-cyclohexen-1-one was obtained in 8% yield. In the oxidation of styrene, 32% styrene oxide and 14% benzaldehyde were produced. Oxidation of α-methylstyrene produced 75% α-methylstyrene oxide. In the oxidation of 4-methylstyrene, 83% 4-methylbenzaldehyde was produced. In the case of linear alkenes, such as 1-octene, the corresponding epoxides were obtained in 11% yield with 100% selectivity.
Oxidation of alkenes with hydrogen peroxide by [Mn(THPP)OAc]@MWCNT under ultrasonic irradiationa.
No. | Alkene | Conversiona,b (%) | Epoxide yieldc (%) | Epoxide selectivityc (%) |
1 | 100 | 100 | 100 | |
2 | 24 | 24 | 100 | |
3 | 32 | 25 | 78 | |
4 | 46 | 32 | 70 | |
5 | 75 | 75 | 100 | |
6 | 83 | 0d | 0 | |
7 | 11 | 11 | 100 |
a Reaction conditions: the molar ratio for catalyst:ImH:cyclooctene:acetic anhydride: H2O2 (30%) are: 1:100:40:1600:1600.
b Reaction time: 30 min.
c GC yield based on the starting alkene.
d The by-product is 4-methylbenzaldehyde.
3.4 Proposed catalytic mechanism
Addition of acetic acid/or acetic anhydride into the reaction mixture resulted in an increase in catalytic activity [43–45]. This observation raised the possibility of the in situ formation of peracetic acid (Scheme 2, I) from acetic acid or/acetic anhydride and H2O2. Peracetic acid by itself is capable of epoxidation, but it required prolonged reaction time or high reaction temperature [46]. On the other hand, the presence of the catalyst is essential for the reaction (Table 4, No. 1); therefore it seems that peracetic acid will be activated by coordination to the Mn-center [47,48], and then oxygen transfer to the olefin will occur.
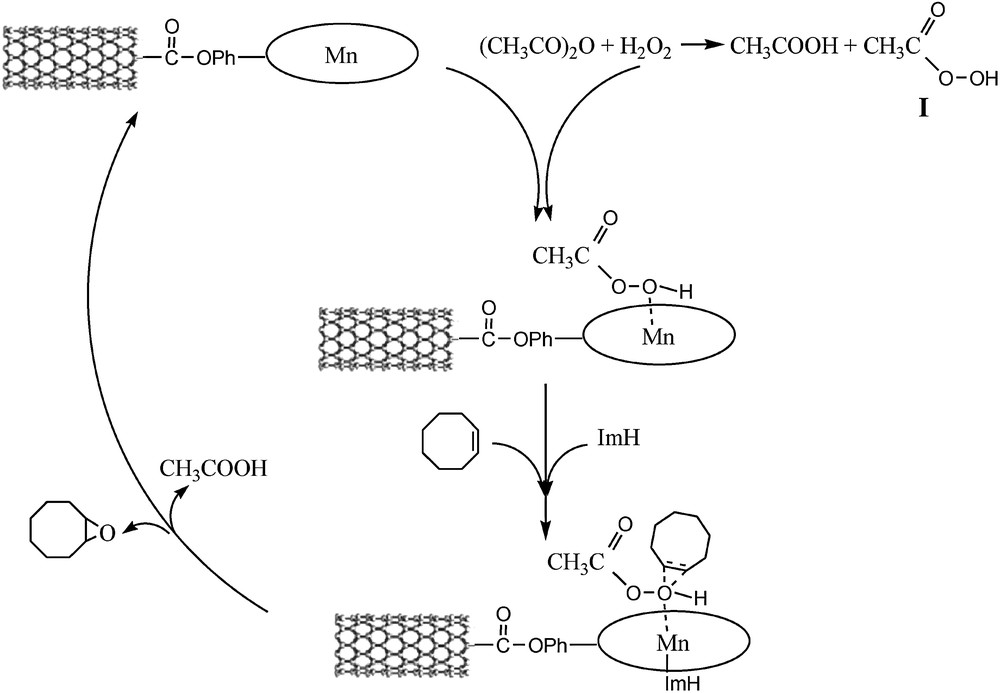
Proposed catalytic cycle.
3.5 Catalyst reuse and stability
The reusability of a heterogeneous catalyst is of great importance in catalyst design. The homogeneous [Mn(THPP)OAc] is readily degraded and cannot be recovered even once; in contrast, the nanotube-supported manganese porphyrin catalyst can be filtered and reused several times without significant loss of its activity.
The reusability of the catalyst was investigated in the multiple sequential epoxidation of cyclooctene with hydrogen peroxide under ultrasonic irradiation. The catalyst was separated from the reaction mixture after each experiment by simple filtration, washed with ethanol (three times) and dried carefully before being used in the subsequent run. After re-use of each catalyst for five consecutive times (with the same reaction times), the epoxide yields were 97%. Furthermore, the IR spectrum of the recovered catalyst was unchanged after it had been reused several times.
A comparison of the results obtained for our present catalytic system with those reported in the literature using hydrogen peroxide as an oxidizing agent [49–52] reveals that the reaction time is shorter than with other systems and that higher conversion rates will be achieved for epoxide formation.
4 Conclusions
In conclusion, this catalytic system is highly efficient for biomimetic epoxidation of alkenes with hydrogen peroxide as an environmentally friendly oxidant, under ultrasonic irradiation in ethanol. Addition of acetic anhydride to the manganese porphyrin/hydrogen peroxide systems led to a remarkable increase in the conversion yields. Irradiation of this catalytic system by ultrasonic waves reduced the reaction times and increased the conversion rates. Excellent selectivity (100%) for epoxide formation was obtained in the case of cyclooctene, cyclohexene, 1-octene, and α-methyl styrene.
Acknowledgements
The financial support of this work by K.N. Toosi University of Technology research council is acknowledged.
1 H2THPP: UV–vis (DMF): λmax (nm): 422, 517, 555, 594, 647. 1H NMR (MeOD, 500 MHz, δ (ppm)): 8.88 (s, 8H, β-pyrrole), 7.99–8.02 (d, 8, o-phenyl), 7.19–7.22 (d, 8H, m-phenyl).