1 Introduction
The construction of C–C bonds is of immense significance for the synthesis of organic compounds and there has been significant interest in the development of novel and highly active catalysts that can be used in C–C bond formation reactions [1]. Glaser's copper-mediated oxidative homocoupling of terminal alkynes for the synthesis of conjugated 1,3-diynes, a representative example of C–C bond formation, is under intense attention for the reason that diynes are precious components in organic synthesis and conjugated 1,3-diynes are recurring building blocks in natural products, pharmaceutical intermediates, electronic and optical materials, and conjugated polymers [2]. Oxidative homocoupling of alkyne is one of the most widely utilized procedures for the synthesis of symmetrical diynes. Despite of the several routes available for the synthesis of conjugated 1,3-diynes, the homocoupling of terminal alkynes is favored due to its simple procedure [3]. The most attractive routes for the homocoupling of terminal alkynes are the catalytic systems containing a combination of palladium (Pd) and copper salts, because of their efficiency and mildness, but palladium reagents are costly and often require expensive ligands and inert conditions. Some palladium-free Cu(I) catalysts have also been utilized for homocoupling, but the susceptibility of Cu(I) salts to redox process desires the utilization of foul smelling nitrogen bases, ligands and/or other additives, etc., to protect and stabilize the active Cu(I) catalyst [4]. Some palladium-free and ligand-free catalytic systems using Cu(I) salts in the presence of non-nitrogenous inorganic bases have also been developed [5].
Jia et al. have described the CuI-mediated alkyne homocoupling reaction, employing inorganic Na2CO3 instead of amines as the base [6]. However, stoichiometric amounts of CuI, 200 mol% base and 100 mol% iodine (as the oxidant) were required. The same group proposed a Cu(I)-catalyzed green oxidative alkyne homocoupling route without palladium, ligands, and bases [7].
Jiang et al. reported the Cu(II)-promoted oxidative homocoupling reaction of terminal alkynes in supercritical carbon dioxide [8], but this protocol requires special equipment, high CO2 pressure and elevated reaction temperatures. Dong Wang et al. reported the homocoupling reactions of terminal alkynes based on catalytic amounts of CuCl2 and triethylamine at 60 °C in air [9]. A copper(II)-chloride-dehydrate-catalyzed oxidative coupling reaction in polyethylene glycol using NaOAc as a base has also been reported [10]. Very recently, a more stable compound, Cu(OAc)2·H2O, has been utilized without any ligand, additive, base or palladium for oxidative alkyne homocoupling [11].
Homogeneous catalysts, though widely used for this purpose, are generally thought to have shortcomings, such as difficulties in catalyst separation and catalyst recycling and product contamination caused by the residual components of the catalysts. So, developing catalytic systems that are stable, easy to handle, inexpensive, environment friendly, and easy to separate and recyclable are highly demanded. Based on these criteria, heterogeneous catalysts are advantageous over their homogeneous counterparts, offering easy recovery, enhanced stability even after recycling them many times [12].
The heterogeneous copper-catalyzed homocoupling of terminal alkynes has also been a subject of recent interest, but the reported catalysts still exhibited some drawbacks. Copper(I) zeolites were tested for this reaction in N,N-dimethylformamide (DMF) at 110 °C and 30 mol% copper loading, with no apparent possibility of catalyst recycling [13]. CuAl hydrotalcite exhibited excellent recyclability at room temperature in acetonitrile, although stoichiometric amounts of N,N,N’,N’-tetramethylethylenediamine (TMEDA) and catalyst (110 mol%) were required [14]. Thus, simpler but effective and environmentally benign novel heterogeneous systems for homocoupling approaches are still demanded. In continuation of our work on heterogeneous catalysis [15], we herein report the efficient heterogeneously catalyzed oxidative homocoupling of alkynes by Cu(II)-clay without any additive (Scheme 1).

2 Experimental
2.1 Catalyst preparation
Our Cu(II)-clay catalyst was prepared by introducing a calculated amount of aqueous Cu(II) oligomer to form a 10 wt % copper loading (pre-optimized loading) onto a montmorillonite-KSF clay; the system was stirred for 15 h, then filtered off and washed several times with distilled water to remove the chlorides. The cake so formed was dried at room temperature, kept overnight in an air oven at 110 °C, powdered and calcined at 425 °C for 3 h to produce the Cu(II)-clay catalyst. The catalyst so obtained was characterized by powder X-ray diffraction using a D-8 ADVANCE (Bruker AXS, Germany), an X-ray diffractometer using an Ni filter and a Gobel Mirror parallel-beam geometry (Cu Kα: λ = 1.5418 Å) in the 2θ range between 5 and 70° in step-scan mode (step size: 0.02°, scan speed: 2 s/step). The phases were identified by search match procedure with the help of DIFFRACPLUS software using JCPDS databank. Temperature programmed reduction (TPR) and BET surface area were determined by the CHEMBET-3000 TPR/TPD/TPO instrument. XPS analysis was performed on a KRATOS-AXIS 165 instrument. SEM observation of the catalyst was carried out using a Jeol Model JEM-100CXII electron microscope with an ASID accelerating voltage of 40.0 kV.
2.2 General procedure for alkyne homocoupling
To a solution of terminal alkyne (0.3 mmol) in DMSO (3 mL) was added 5 mol% Cu(II)-clay in a two-neck round-bottom flask. A condenser was attached to one neck of this flask, whereas the other neck was closed with a rubber septum. Oxygen was bubbled through a needle inserted through the septum. The resulting mixture was refluxed for the required duration. The reaction was cooled at room temperature and the products were extracted with dichloromethane. The combined organic layers were dried with anhydrous MgSO4, filtered off, and concentrated in vacuo to obtain the crude products, which were purified by silica-gel chromatography to afford the corresponding product. The prepared compounds were characterized by comparing the observed spectral data and physical properties with those of the authentic samples. NMR spectra were recorded on a Bruker Avance DPX FT-NMR 400 MHz instrument. ESI–MS and HRMS spectra were recorded on Agilent 1100 LC and HRMS-6540-UHD machines. The melting points were recorded on a digital melting point apparatus. IR spectra were recorded on a PerkinElmer IR spectrophotometer.
3 Results and discussion
3.1 Morphological analysis of the materials
The XRD patterns of the fresh Cu(II)-clay catalyst are shown in Fig. 1 (left). The peak at 2θ = 8.4° is commonly assigned to the basal spacing (d 001) (2:1 TOT) of montmorillonite-KSF; the basal reflection represents the distance between two clay layers, including the thickness of one of them [16]. The very low intensity diffraction peaks at 2θ = 36.1, 37.7, 38.9, 48.8, 53.60, and 58.7 are attributed to hkl values 110, 002, 111, 202 of CuO, indicating that there are no sharp crystalline phases; thus, CuO is supported on montmorillonite-KSF in the form of highly dispersed fine particles [17], which is in agreement with the TPR studies mentioned below. The peaks at 2θ = 20.9, 26.9, 36.5, 39.2, 48.6, 50.0 and 59.6 are due to the reflection of the quartz [SiO2] impurities [18]. Other peaks for montmorillonite appear at 2θ = 20.0, 24.3, 29.8, and 35.5. A sharp peak observed at 2θ = 17.8 along with some small peaks at 30.1, 36.0 and 47.2 correspond to the presence of melanothallite [Cu2OCl2]. The presence of kaolinite is proved by a sharp peak at 12.0, which corresponds to hkl 001, whereas other peaks characteristic of this material appear at 24.1, 32.3, 38.0, and 42.7. The remaining peaks at 29.5, 32.3, 41.5, 45.5, 55.0, and 59.6 are due to the formation of CuAl2O4 [19]. There are two additional humps, the first one at 55.0 and the other one at 61.9, which are due to montmorillonite and CuO in bulk, respectively [20]. By comparison of the XRD patterns of the fresh and of the used catalysts (Fig. 1, right), it is clear that there is almost no variation.

Left: XRD of fresh Cu(II)-clay. Right: XRD of used Cu(II)-clay.
To study the morphology, SEM observations of the catalyst were carried out using the Jeol Model JEM-100CXII Electron Microscope with an ASID accelerating voltage 40.0 kV. The SEM images of the montmorillonite-KSF and Cu(II)-clay with 20 000 magnifications are displayed in Fig. 2. From this study, it is clear that after loading copper on montmorillonite-KSF, the smooth surface of the clay turns patchy, so, it is confirmed that the CuO particles are highly dispersed over the support surface in the form of nano-aggregates. The nano-sized CuO distributed in the form of a mosaic on the surface of the clay leads to a coarse surface (thus, an elevated surface area). From the SEM (EDAX) elemental analysis, it was found that the prepared catalyst contained 9.21% of copper loaded.
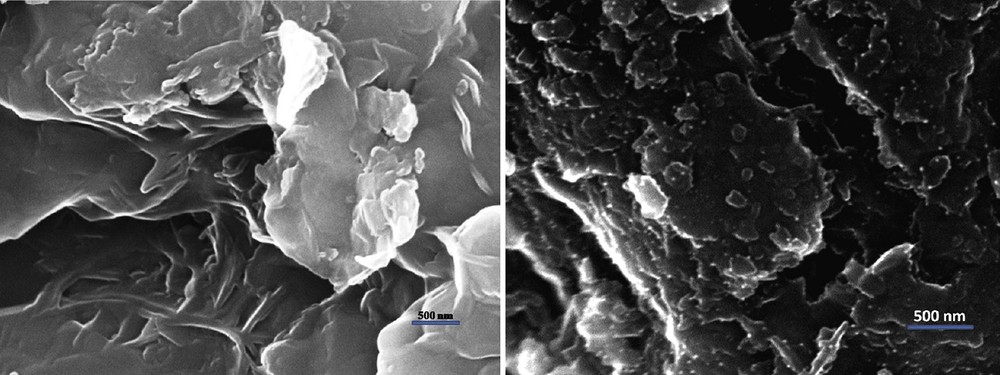
Left: SEM image of montmorillonite-KSF. Right: SEM image of Cu(II)-clay.
TPR is an important technique to characterize the dispersion of metal ions in catalysts for understanding the reduction behavior and thermal stability of the active metal oxide particles. The reduction profile provides information about the dispersion of the metal species on the support and about the interaction between these metal species and the support. The H2–TPR curve for the unmodified montmorillonite-KSF and that modified with copper is depicted in Fig. 3. The unmodified montmorillonite-KSF support showed a broad peak at a higher reduction temperature (Fig. 3, left), which can probably be attributed to the reduction of some iron species in the montmorillonite-KSF material [21]. It has been reported that CuO reduces directly to Cu0 [22] and isolated Cu2+ ions can be reduced to Cu0 by hydrogen in two steps: first, the reduction of Cu2+ to Cu+; and second, the reduction of the Cu+ to Cu0 [23]. The reduction of CuO to Cu0 and isolated Cu2+ to Cu+ occur at lower temperatures than the reduction of Cu+ to Cu0. The major reduction peak observed for the catalyst, centered at 220 °C, was attributed to the reduction of copper oxide located on the surface of the montmorillonite-KSF, probably in the entrance of the pores. The second broad peak at about 370 °C, which is less pronounced, may be attributed to the overlapping of reduction of the isolated Cu2+ to Cu+ ions and the copper oxide species located in the interlamellar space of montmorillonite-KSF [23a,24]. The peak for the Cu+ reduction at 460 °C seems to be less intense and overlapped with the reduction peak of iron impurities in montmorillonite-KSF [25]. The results indicate that the copper ions are located mostly in extra-framework positions in the exchanged form. The different shoulders observed for these solids indicate that they contain Cu2+ species present in small clusters in different environments. The TPR profile of the catalyst suggests that it contains very high exchanged copper sites and that all of the isolated Cu2+ sites observed for Cu(II)-clay catalyst are due to the exchange with the Brønsted acid sites in montmorillonite-KSF. Since the intensity of the peak representing the reduction of CuO particles located on the surface of the montmorillonite-KSF is the highest, this suggests that the highly dispersed CuO species are dominating. This indicates that the main species detected is copper oxide, as CuO aggregates with different particle sizes and dispersion. In this respect, the obtained TPR profile clearly reveals a higher copper heterogeneity, in agreement with other characterization results described above.
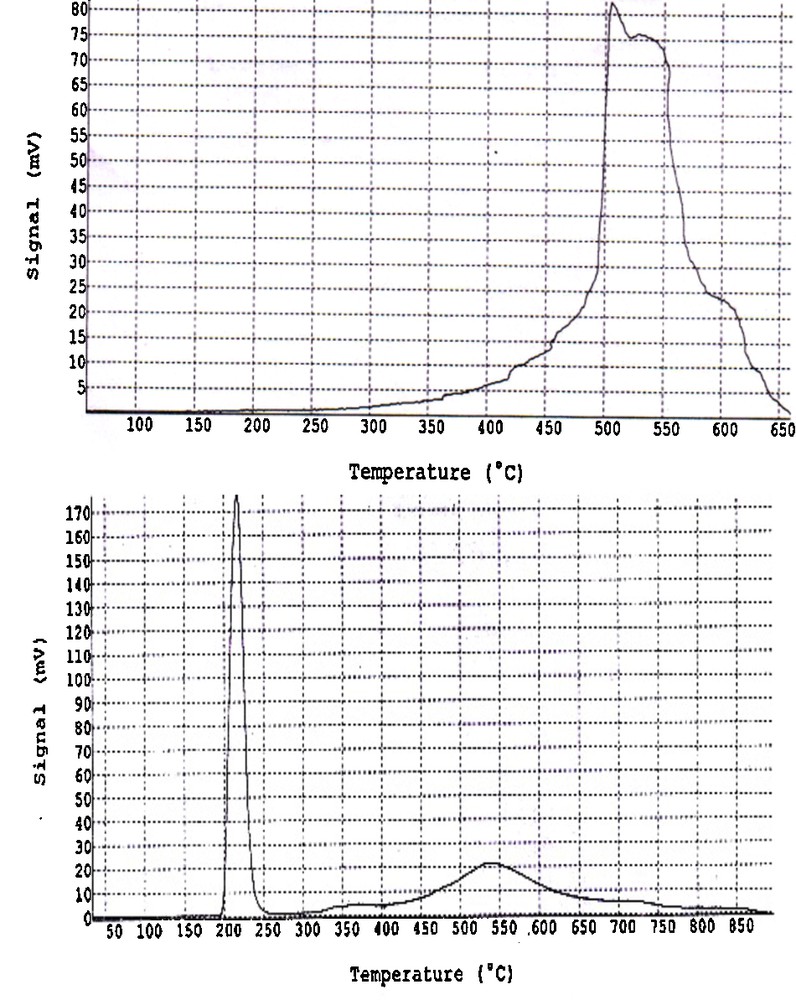
Left: TPR image of montmorillonite-KSF. Right: TPR image of Cu(II)-clay.
3.2 Homocoupling of terminal alkynes
At the onset of this work, we focused on the optimization of the reaction conditions for the homocoupling of phenylacetylene as a model reaction in tetrahydrofuran medium (Table 1) using Cu(II)-clay as the catalyst under atmospheric conditions. Screening was conducted to optimize copper loading on montmorillonite-KSF support. The catalyst with 10 wt% loading was found to be an ideal catalyst for the model reaction showing > 99% conversion and almost 98% product yield within 3 h in the absence of any base, palladium, ligand, reducing agent or external oxygen supply (Table 1, entries 1–8). In contrast to the situation when other terminal alkyne substrates were subjected to the same reaction conditions, only traces of the desired products were obtained even after 24 h, indicating that these conditions are appropriate for phenylacetylene homocoupling only. Thus, in search of appropriate reaction conditions applicable for all the substrates used in our present study, we changed the model reactant from phenyacetylene to 4-tolylacetylene (Table 1, entry 9). Increasing the catalyst amount or catalyst loading could not improve the product yield of this model reaction (Table 1, entries 10, 11). Upon investigating the effect of temperature, we found that there is only little increase in the product yield, even at 100 °C (Table 1, entries 12–14). When molecular oxygen was bubbled through the reaction mixture, stirred at 100 °C, the product yield increased abruptly (Table 1, entry 15). Similar results were obtained at 80 °C (Table 1, entry 16), but at lower temperatures, supplying molecular oxygen proved unproductive (Table 1, entries 16–18). Thus, both O2 as well as high temperature are necessary for the Cu(II)-clay mediated homocoupling of terminal alkynes, except for phenylacetylene homocoupling, for which the catalyst alone does the work. Since the control experiments showed that no reaction occurred with unmodified montmorillonite-KSF, these results demonstrated that Cu(II)-clay acts as a true catalyst for the homocoupling of terminal alkynes.
Optimization of reaction conditions.
Entry | Substrate | Catalyst amount (mg) | Catalyst loading (wt %) | Temperature | Atmosphere | Time | Yielda |
1 | 20 | 5 | RT | Air | 3 h | 67% | |
2 | 20 | 10 | RT | Air | 3 h | 98% | |
3 | 20 | 15 | RT | Air | 3 h | 98% | |
4 | 20 | 20 | RT | Air | 3 h | 98% | |
5 | 10 | 10 | RT | Air | 3 h | 73% | |
6 | 15 | 10 | RT | Air | 3 h | 79% | |
7 | 25 | 10 | RT | Air | 3 h | 98% | |
8 | 30 | 10 | RT | Air | 3 h | 98% | |
9 | 20 | 10 | RT | Air | 24 h | Traces | |
10 | 30 | 10 | RT | Air | 24 h | Traces | |
11 | 50 | 10 | RT | Air | 24 h | Traces | |
12 | 20 | 10 | 50 | Air | 24 h | Traces | |
13 | 20 | 10 | 80 | Air | 24 h | 11% | |
14 | 20 | 10 | 100 | Air | 24 h | 11% | |
15 | 20 | 10 | 100 | O2 | 25 min | 97% | |
16 | 20 | 10 | 80 | O2 | 25 min | 97% | |
17 | 20 | 10 | 80 | O2 | 25 min | 98% | |
18 | 20 | 10 | RT | O2 | 24 h | Traces |
a Isolated yield.
A range of solvents were screened to determine the best fit for this method, we observed that dimethylsulfoxide (DMSO) shows excellent behavior as a medium for terminal alkyne homocoupling conducted at 80 °C in the presence of molecular oxygen (Table 2, entry 7). It is evident that solvents, like DMSO, DMF, toluene, and acetonitrile (Table 2, entries 5–8) exhibited significant superiorities over other solvents, like methanol, ethanol and THF (Table 2, entries 2–4). The use of water and solvent-free conditions led only to traces of the desired product (Table 2, entries 1 and 9).
Solvent optimization.
Entry | Solvent | Temperature (°C) | Time (min) | Yielda |
1 | Water | 80 | 320 | Traces |
2 | Ethanol | 80 | 30 | 13% |
3 | Methanol | 80 | 30 | 13% |
4 | THF | 80 | 30 | 11% |
5 | Toluene | 80 | 30 | 67% |
6 | CH3CN | 80 | 30 | 63% |
7 | DMSO | 80 | 30 | 97% |
8 | DMF | 80 | 30 | 80% |
9 | Neat | 80 | 30 | Traces |
a Isolated yield.
With optimized conditions in hands, the substrate scope of the catalytic oxidative coupling was studied, as summarized in Table 3. Various terminal alkynes, including aliphatic and aromatic ones, were examined under the optimal conditions; the results are summarized in Table 3. Arylacetylenes showed excellent reactivity to produce the coupling products, that is, aromatic diynes (Table 3, entries 1–9). For aromatic alkynes, the homocoupling processes were affected dramatically by the substituents on the aromatic ring. Electron-donating substituents, such as alkyl and methoxy groups facilitate the reaction, allowing better yields and shorter reaction times (Table 3, entries 6–8), while electron-withdrawing groups, like–F, –CF3, –NO2, etc., were found to decrease the transformation rate very much (Table 3, entries 2–5). Linear aliphatic alkynes of 1-hexyne, 1-heptyne, and 1-decyne were also converted into the corresponding aliphatic diynes in high yields (Table 3, entries 10–12). Ethynylcyclopropane was also found to react smoothly to afford the desired product with good yields (Table 3, entry 13). Propargyl alcohol was found to react very slowly to give the corresponding diyne with low yields (Table 3, entry 15).
Homocoupling of terminal alkynes catalyzed by Cu(II)-clay.
Entry | Substrate | Temperature | Time (min) | Atmosphere | Yield (%)a |
1 | 80 | 25 | O2 | 98 | |
2 | 80 | 90 | O2 | 67 | |
3 | 80 | 120 | O2 | 58 | |
4 | 80 | 120 | O2 | 53 | |
5 | 80 | 60 | O2 | 89 | |
6 | 80 | 25 | O2 | 97 | |
7 | 80 | 25 | O2 | 96 | |
8 | 80 | 35 | O2 | 83 | |
9 | RT | 180 | Air | 98 | |
10 | 80 | 30 | O2 | 88 | |
11 | 80 | 30 | O2 | 89 | |
12 | 80 | 30 | O2 | 93 | |
13 | 80 | 25 | O2 | 88 | |
14 | 80 | 45 | O2 | 78 | |
15 | 80 | 120 | O2 | 65 |
a Isolated yield
Comparing catalytic activity of the catalyst with some clay-supported copper bimetallic catalysts (prepared for this study) and commercially available CuO and Cu2O for the model reaction under optimized conditions, we found that combining other metals with Cu(II) does not improve the activity of the catalyst; no improvement in the results was observed; some decreased yields were obtained instead (Table 3, entries 1–7). The Cu(II)-clay catalyst was also found to show far better results than the commercially available CuO and Cu2O (Table 3, entries 8 and 9). This might be due to the high dispersion and to the nano-sized character of Cu(II) on clay support [26,27] (Table 4).
Comparison of catalytic activity of Cu doped clay catalyst with other Cu catalysts.
Entry | Catalyst | Mol% of catalyst | Solvent | Time (min) | % Yielda |
1 | CuNi/clay | 10 | DMSO | 30 | 53 |
2 | CuMn/clay | 10 | DMSO | 30 | 53 |
3 | CuZn/clay | 10 | DMSO | 30 | 61 |
4 | CuAl/clay | 10 | DMSO | 30 | 58 |
5 | CuFe/clay | 10 | DMSO | 30 | 39 |
6 | CuSn/clay | 10 | DMSO | 30 | 62 |
7 | CuCo/clay | 10 | DMSO | 30 | 65 |
8 | CuO | 10 | DMSO | 30 | 34 |
9 | Cu2O | 10 | DMSO | 30 | 43 |
a Isolated yield.
Recyclability of the catalyst was investigated for the homocoupling of 4-tolylacetylene as a model reaction. This experiment proved excellent recycling capability of the catalyst without significant loss of activity. The catalyst could be easily recovered by simple filtration and reused without any pre-treatment. Conversions were recorded in the homocoupling of 4-tolylacetylene for at least six consecutive runs. These results point to a process of heterogeneous nature (Fig. 4).

Recyclability of the catalyst.
4 Conclusions
In conclusion, we have developed an easy-to-prepare, robust, heterogeneous, and inexpensive catalyst for the synthesis of 1,3-butadiynes from terminal alkynes. A full characterization of the catalyst allowed concluding that the copper nanoparticles are mainly oxidized in the form of CuO. This catalyst was found to be much more efficient than other commercially available catalysts based on copper. The catalyst is environmentally friendly and can be recycled several times without significant loss of activity. This method is an efficient and simple process for the synthesis of 1,3-butadiynes without requiring palladium, base, ligand, or additive. Although phenylacetylene homocoupling does not need high temperature or oxygen supply, the other congeners react under reflux under O2 atmosphere. Overall, the present procedure is fast, practical, interesting and inexpensive for alkyne homocoupling. These features make this methodology economical and attractive for use in combinatorial chemistry and industrial preparation.
Acknowledgement
We are grateful to the Director of the Indian Institute of Integrated Medicine (IIIM), Canal Road, Jammu for providing necessary facilities and good environment to carry out the research work. We are also thankful to CSIR–Delhi for financial support.