1 Introduction
Nanoparticles can effectively improve the loading and the catalytic efficiency of immobilized catalysts due to their high surface area:volume ratios. Therefore, the use of nanostructured materials as supports for different types of catalyst immobilization remains an attractive field to the researchers [1,2]. Magnetic nanoparticles have obtained considerable interest in recent years [3–5]. The magnetic nature of these particles allows the easy recovery and recycling of the catalysts by an external magnet, which may optimize operational cost and improve product purity. Recently, magnetic nanoparticles, such as Fe, FePt, Fe2O3, Fe3O4, ZnFe2O4, MnFe2O4, NiFe2O4 and CoFe2O4, have been studied mostly for biomedical applications [6–11]. As Fe-based metallic nanoparticles are chemically not stable [12], therefore, iron oxides, in particular, spinel ferrites with a general formula (AB2O4), have attracted a great consideration. Ni ferrites are one of the most versatile magnetic materials as they have high saturation magnetization, high Curie temperature, chemical stability and relatively high permeability [13]. Due to the sensitivity of the magnetic nanoparticles and the strong surface affinity toward silica, these nanoparticles can be directly coated with amorphous silica. In spite of the recoverability of the silica-supported polyphosphoric acid (PPA), the slow recycling of catalyst by filtration and the unavoidable loss of some solid catalyst in the separation process are some of the drawbacks of the traditional heterogeneous PPA–SiO2. Although the catalytic applications of silica-supported reagents for organic synthesis are well established, it is more interesting to introduce a novel version of PPA immobilized onto magnetic silica for more efficient catalyst recovery, especially from the green chemistry viewpoint. Due to the reasonable needs to clean and green recovery of the heterogeneous catalyst, especially acid catalyst, we decided to design a novel version of silica-supported polyphosphoric acid to ease the efficient recycling of this catalyst. In the present work, an efficient method for the preparation of PPA-functionalized silica-coated magnetic nanoparticles [NiFe2O4@SiO2–PPA] is reported. The prepared composite was successfully used as the catalyst for the N-formylation of amines.
N-formylation of amines is a very important reaction in organic synthesis and medicinal chemistry. A variety of medicinally active compounds have formamides as their intermediates [14]. N-Formyl protection gained popularity in peptide synthesis [15], since N-formyl deprotection can easily be achieved without affecting the peptide bond, and it may serve as a precursor for isocyanide [16] and formamidine [17] synthesis. Reported literature methods for the N-formylation [17–21] use reagents, such as chloral, formic acid–DCC, formic acid–EDCI, formic acid–ZnCl2, formic acid–PEG 400, formic acid esters, CMT, DMF–NaOMe, formic acid–thiamine hydrochloride, imidazole–DMF, HClO4–SiO2, and formamide–NaOMe. Most of these methods suffer from some disadvantages, such as harsh reaction condition, low yield, very high reaction temperature, expensive reagents, etc. Due to our interest in the synthesis of heterocyclic compounds and in continuation of our previous works on the applications of reusable catalysts in organic reactions [22–24], here, we report an efficient and green method for the N-formylation of amines using NiFe2O4@SiO2–PPA as a catalyst (Scheme 1). To the best of our knowledge, this is the first report on the synthesis, characterization and catalytic performance of a NiFe2O4@SiO2–PPA catalyst.

N-formylation of amines in the presence of NiFe2O4@SiO2–PPA.
NiFe2O4@SiO2 nanocrystallites were prepared according to the reported procedure by Massart et al. with minor modifications: fine particles are precipitated in an alkaline solution [25]. (Scheme 2).

Preparation of polyphosphoric acid supported on NiFe2O4@SiO2.
2 Experimental
2.1 Preparation of the catalyst (NiFe2O4@SiO2–PPA) [25,26]
The solution of metallic salts (FeCl3, 160 mL, 1 M and NiCl2, 40 mL, 1 M) was poured as quickly as possible into the boiling alkaline solution [NaOH (1000 mL, 1 M)] under vigorous stirring. Then, the solution was cooled and continuously stirred for 90 min. The resulting precipitate was then purified by four-times repeated centrifugation (4000–6000 rpm, 20 min) and decantation. Coating of a layer of silica on the surface of the NiFe2O4 nanoparticles was achieved by (ultrasonically) premixing a dispersion of the nanoparticles (8% w/w, 25 mL) obtained with ethanol for 2 h at 60 °C. A concentrated ammonia solution was added and the resulting mixture was stirred at 60 °C for 40 min. Then, tetraethylorthosilicate (TEOS) (1.0 mL) was added to the reaction mixture and continuously stirred at 60 °C for 24 h. The silica-coated nanoparticles were collected by an external magnet, followed by washing three times with methanol and drying in vacuum for 48 h. After that, the resulting magnetic nanoparticles were calcined at 800 °C for 4 h. Polyphophoric acid (PPA) (2.2 g) was charged in the round-bottom flask, and CHCl3 (100 mL) was added. After the mixture had been stirred at 50 °C for 1 h, NiFe2O4@SiO2 (5.0 g) was added to the solution, and the mixture was stirred for another 4 h. The CHCl3 was removed with a rotary evaporator and the resulting solid was washed with cold absolute ethanol, and dried in vacuo at 70 °C for 2 h. The amount of H+ in the NiFe2O4@SiO2–PPA, determined by acid–base titration, was 0.50 mmol/g.
2.2 Typical experimental procedure for N-formylation
A solution of 4-methylaniline (1.0 mmol), formic acid (1.5 mmol), and NiFe2O4@SiO2–PPA (0.02 g, 1 mol%) was stirred at room temperature for 10–30 min. The progress of the reaction was monitored by TLC. Upon completion, ethyl acetate was added to the reaction mixture (the product is soluble in ethyl acetate) and NiFe2O4@SiO2–PPA could be placed on the side wall of the reaction vessel with the aid of an external magnet, then the catalyst was washed and dried to be reused in the next run. The organic phase was washed with a saturated solution of NaHCO3 (5 mL × 3), water, and dried over anhydrous Na2SO4. The solvent was evaporated and the resulting crude product was collected and give a white solid, yield 93%, mp: 52–54 °C, 3291, 1664 (CO); 1H NMR (300 MHz, CDCl3) δ 2.30 (s, 3H), 7.08–7.61 (m, 4H), 8.11 (s, 1H), 8, 15 (s, 1H).
3 Results and discussion
3.1 Characterization of NiFe2O4@SiO2–PPA
NiFe2O4@SiO2–PPA nanocrystallites were characterized by FT–IR (Fig. 1), XRD (Fig. 2) TEM (Fig. 3) and SEM (Fig. 4). FT–IR spectra of NiFe2O4@SiO2, PPA and NiFe2O4@SiO2 -PPA are compared in Fig. 1. In the FT–IR spectrum of NiFe2O4@SiO2–PPA (Fig. 1), most of the bands correspond to NiFe2O4@SiO2 (a) and PPA (b), and are observable with a slight shift, which shows that PPA has been adsorbed well on the SiO2 surface. The FT–IR spectrum of NiFe2O4@SiO2 exhibits strong bands in the low-frequency region (1000–500 cm−1) due to iron oxide skeleton, which agrees with the magnetite spectrum. The characteristic bands of Si–O–Fe and Si–O are observed at 798 and 1087 cm−1, respectively. The peaks at 1440–1650 cm−1 also showed the existence of Fe–O vibrations. To confirm the formation of Ni ferrite in the synthesized magnetic nanoparticles, the XRD spectrum of the sample was studied. The XRD pattern (Fig. 2) shows that these nanoparticles have a spinel structure, with all the major peaks matching the standard pattern of bulk NiFe2O4 (JCPDS 10–325). The TEM and SEM photographs of the sample are displayed in Figs. 3 and 4, respectively. Both the SEM and TEM images demonstrate that the prepared magnetic nanoparticles are spherical, narrowly distributed, and well dispersed, with average size less than 50 nm in diameter.
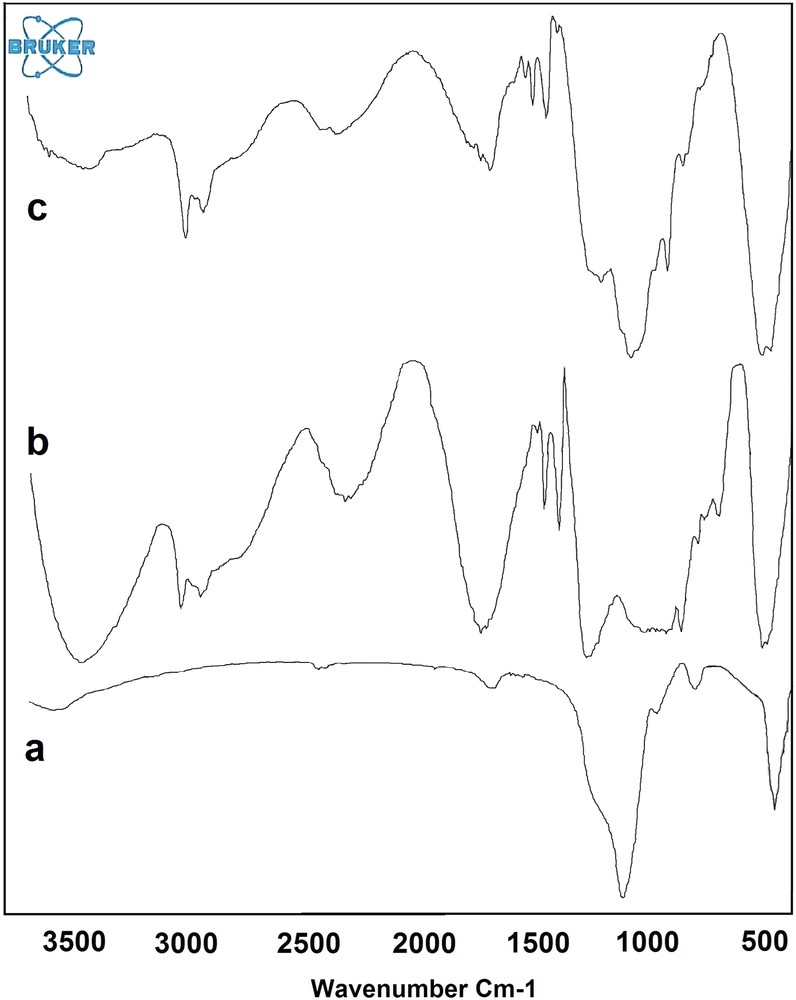
The FT–IR spectrum of (a) NiFe2O4@SiO2 (b) PPA and (c) NiFe2O4@SiO2–PPA.
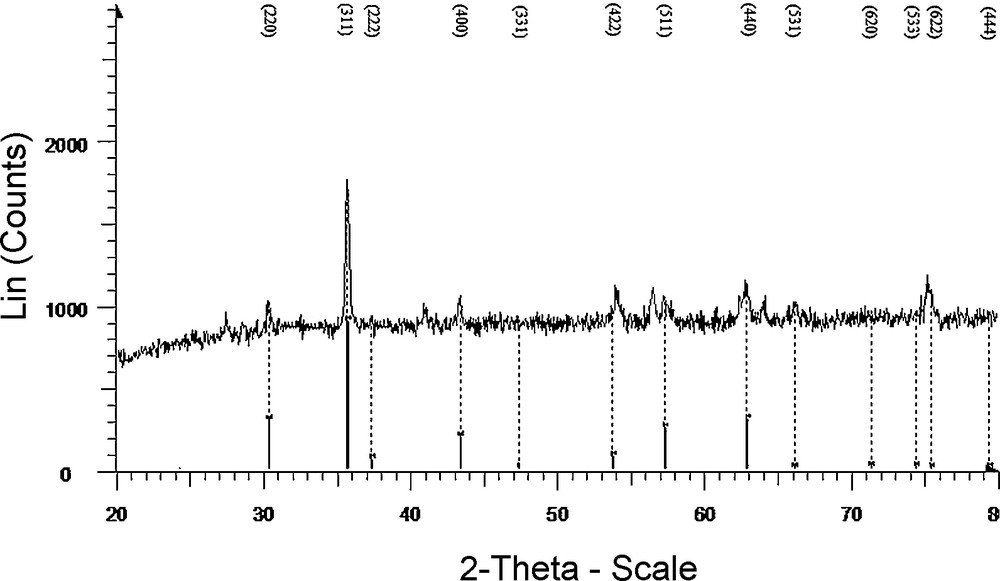
XRD spectrum of NiFe2O4 nanoparticles.
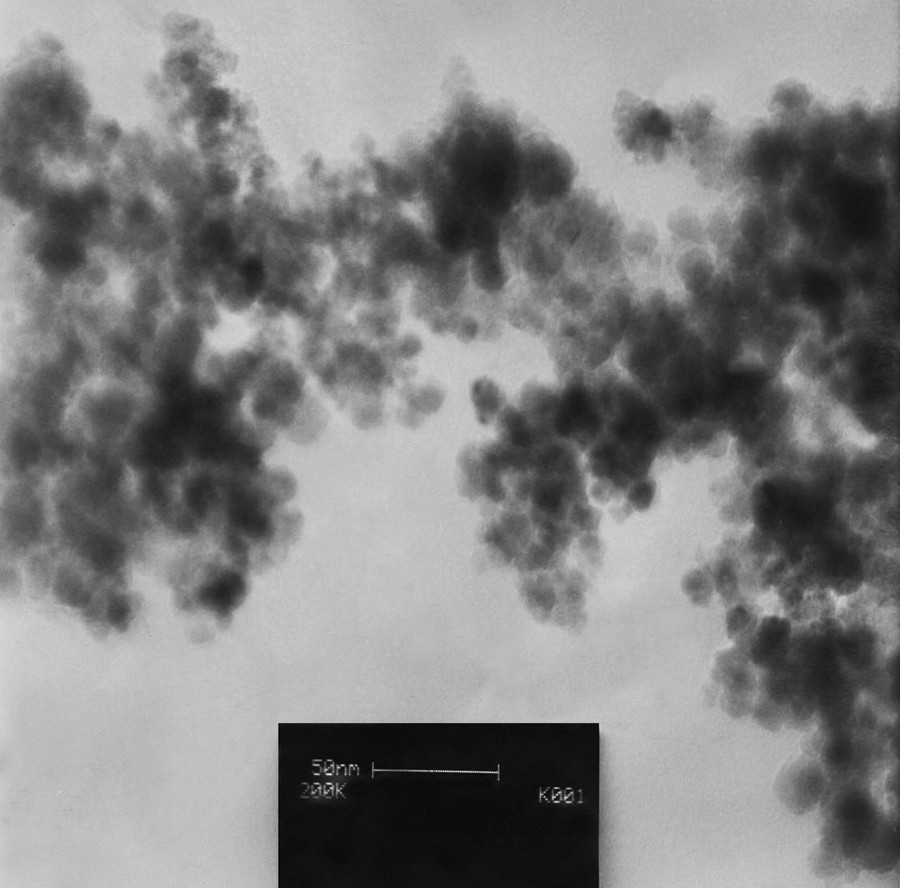
TEM image of NiFe2O4@SiO2 nanoparticles.
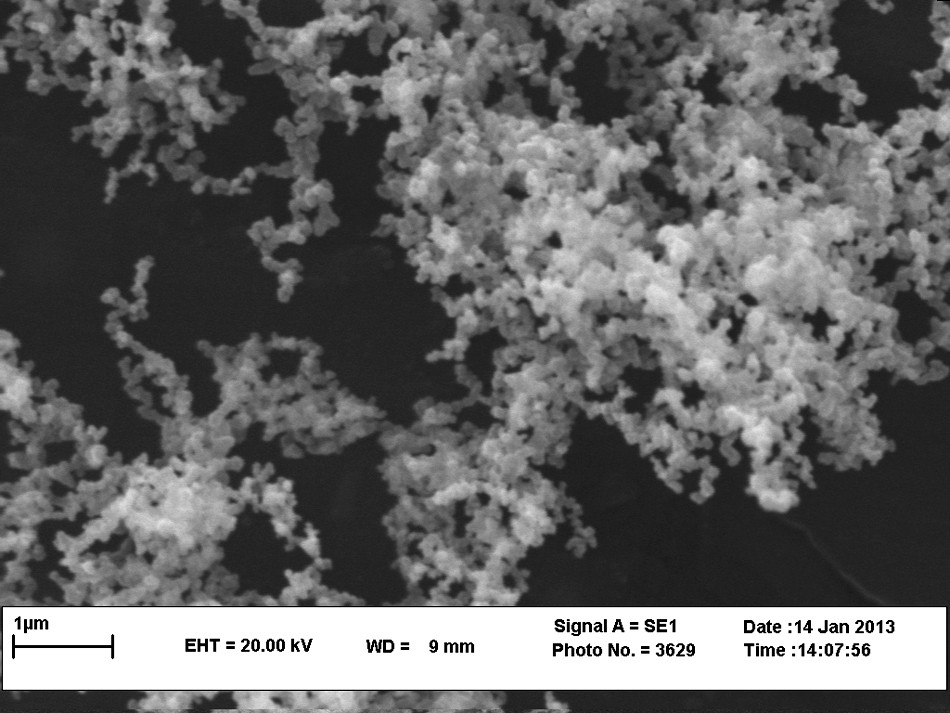
SEM image of NiFe2O4@SiO2 nanoparticles.
3.2 The catalytic application of NiFe2O4@SiO2–PPA in N-formylation of amines
At first, the reaction of 4-methylaniline and formic acid was selected as a model reaction at room temperature without any catalyst or solvent to optimize the reaction conditions. No reaction was observed after 5 h of stirring. However, with the addition of catalyst (0.4 mol%) to the reaction mixture, the reaction occurred in 15 min with 58% yield; further study showed the best amount of catalyst was 1 mol%, giving the corresponding N-formylated 4-methylaniline with 93% yield in 10 min (Table 1).
Effect of the amount of NiFe2O4@SiO2–PPA on the model reaction (1 g of the catalyst contains 0.5 mmol of H+)a.
Entry | Catalyst mol% (g) | Time (min) | Yield (%)b |
1 | None | 300 | None |
2 | 0.4 (0.008 g) | 15 | 58 |
3 | 0.5 (0.010 g) | 15 | 69 |
4 | 1 (0.020 g) | 10 | 93 |
5 | 2 (0.040 g) | 10 | 93 |
6 | 4 (0.080 g) | 10 | 94 |
a 4-Methylaniline (1 mmol) and formic acid (1.5 mmol) under neat conditions.
b Isolated yields.
Using the optimized reaction conditions, the scope and efficiency of this approach was demonstrated for the synthesis of a wide variety of N-formylated aniline derivatives and the obtained results are summarized in Table 2. All the reactions on a wide range of aromatic aldehydes bearing both electron-donating and electron-withdrawing substituents afforded good yields of the product. As it can be observed in Table 2, the reaction with aromatic amines carrying halogens or an electron-donating group is fast and proceeds to completion within 10–18 min, leading to the formation of the N-formylated product in 89–93% yields (entries 1–7), while the presence of electron-withdrawing groups, like nitro ones, lengthened the reaction time to 25–30 min (entries 8, 9). The method is also efficient for secondary amines and aliphatic amines (entries 11–13). In all cases, the obtained product was isolated by a very simple work-up.
N-formylation of primary aromatic amines 3a–m using NiFe2O4@SiO2–PPA (0.02 g, 0.01 mmol H+) as a catalysta.
Entry | Amine | Productb | Time (min) | Yields (%)c |
1 | 18 | 92 | ||
2 | 15 | 91 | ||
3 | 10 | 93 | ||
4 | 15 | 92 | ||
5 | 12 | 98 | ||
6 | 15 | 89 | ||
7 | 10 | 93 | ||
8 | 25 | 92 | ||
9 | 30 | 89 | ||
10 | 25 | 86 | ||
11 | 30 | 76 | ||
12 | 25 | 89 | ||
13 | 30 | 85 |
a Aryl or aliphatic amine (1 mmol), formic acid (1.5 mmol), and NiFe2O4@SiO2–PPA (0.02 g) at rt.
b All the products were identified by comparing their melting points and spectral data (IR and 1H NMR) with those of authentic samples.
c Isolated yields.
The reusability of the catalyst was also investigated. For this purpose, the same model reaction was again studied under optimized conditions. After the completion of the reaction, ethyl acetate was added to the reaction mixture to dissolve the product. With the aid of an external magnet, the catalyst was held on the sidewall of the reaction vessel, while the solution was decanted. The catalyst was washed with diethyl ether, dried at 60 °C under vacuum for 1 h, and reused for a similar reaction. As shown in Fig. 5, the catalyst could be reused at least five times without significant loss of its activity. According to Davoodnia et al. report, PPA–SiO2 can be hydrolyzed to free H3PO4 only after prolonged heating in water [27]. Therefore, because the model reaction occurred under solvent-free conditions at room temperature, water produced in the condensation reaction as a by-product could not affect the catalyst's reactivity. We believe that PPA is bonded to SiO2 in a similar manner as the compound reported in the literature [28].
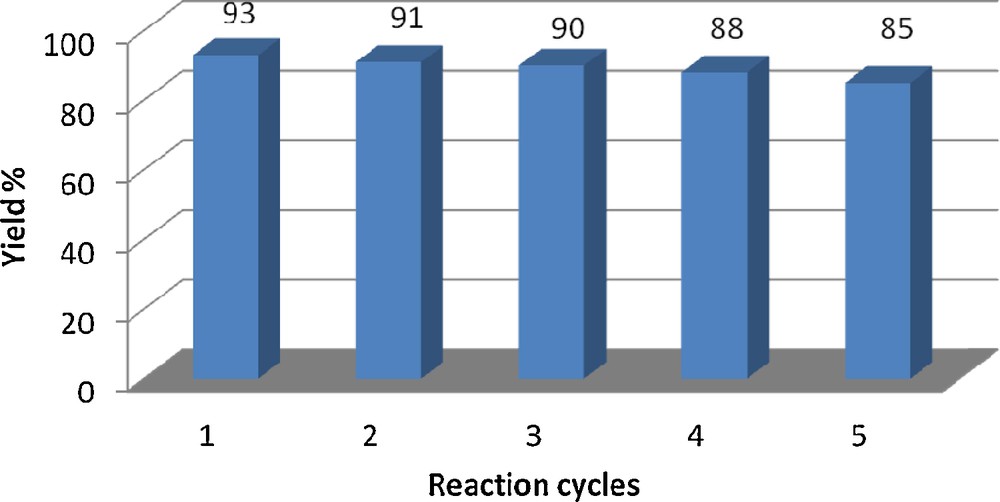
Catalyst reusability test.
To show the merit of the present work, we compared the results of NiFe2O4@SiO2–PPA in the N-formylation of aniline with formic acid to other protic acids immobilized on silica gel, like HClO4–SiO2 [20], H2SO4–SiO2 [29], HBF4–SiO2 [30], and TFA–SiO2 [31]. All the catalysts were tested at the same catalytic loading. As shown in Table 3, NiFe2O4@SiO2–PPA is the more efficient catalyst and gives high yields of product in shorter reaction times. In addition, the advantages of NiFe2O4@SiO2–PPA are its recyclability and its very simple work-up.
Comparison of N-formylation with other silica-supported protic acids.
Entry | Catalyst | Time (min) | Yield (%) |
1 | NiFe2O4@SiO2–PPA | 12 | 98 |
2 | HClO4–SiO2 | 15 | 96 |
3 | H2SO4–SiO2 | 35 | 55 |
4 | HBF4–SiO2 | 40 | 50 |
5 | TFA–SiO2 | 20 | 48 |
4 Conclusion
In conclusion, we report a new, simple and green catalytic method for the N-formylation of aromatic amines with formic acid using polyphosphoric acid supported on silica-coated NiFe2O4 magnetic nanoparticles (NiFe2O4@SiO2–PPA) at room temperature and under solvent-free conditions. The catalyst could simply be recovered with the aid of an external magnet, and reused at least five times without significant loss of its catalytic activity. High yields, short reaction times, easy work-up, and the absence of any volatile and hazardous organic solvents are some advantages of this protocol.
Acknowledgments
The authors are grateful to Ferdowsi University of Mashhad for financial support.