1 Introduction
Nanotechnology is now expanding very rapidly, as a result of the unique physical and chemical properties that nanoparticles exhibit compared to bulk materials. Magnetic Fe3O4 nanoparticles (Fe3O4–MNPs) have attracted much research interest over the recent years because of their inherent properties, such as large surface area and fast response under applied external magnetic field, superparamagnetism properties, high coercivity, and low Curie temperatures [1–8].
Magnetic nanoparticles are usually stabilized by the steric repulsion exerted by polymeric surfactants adsorbed on their surfaces, preventing them from being approached by the neighbouring nanoparticles [9,10]. Oleic acid has been used as a steric stabilizer to form Fe3O4 nanoparticles dispersed in organic medium [11,12]. To explain the stabilizing mechanism of the nanoparticles, it has been proposed that carboxylate groups partition on the surface of the nanoparticles and form a layer of hydrocarbon, sterically, stabilizing the nanoparticles in the dispersion [13,14].
Amphiphilic copolymers as block copolymers are one kind of excellent compounds for water-dispersible magnetite nanoparticles due to the nature of the micelles and their small size [15] Polymeric micelles formed in aqueous phase through the self-association of amphiphilic copolymers have attracted great interest. The micelles, based on amphiphilic block copolymers, are suitable for dispersing magnetite nanoparticles in water phase. An important new class of block copolymer is based on poly(2-alkyl-2-oxazoline) as a biodegradable copolymer. Block copolymers were synthesized based on poly(2-ethyl-2-oxazoline) as the hydrophilic block and poly(l-lactide) or poly(ɛ-caprolactone) as the hydrophobic block [16,17].
Recently, several works have been focused on the preparation of water-dispersible magnetite nanoparticles using polymeric micelles [18,19]. Adeli et al. [20] reported a new method to improve the functionality and water solubility of CNT/γ-Fe2O3 nanoparticles using linear-dendritic copolymers, and CNT/γ-Fe2O3 hybrid nanomaterials were obtained. In this research, poly(citric acid)–polyethylene glycol–poly(citric acid) linear-dendritic copolymers were used to improve the water solubility of CNT/γ-Fe2O3 nanoparticles. Non-covalent interactions between them and CNT/γ-Fe2O3 nanoparticles led to PCA–PEG–PCA/CNT/γ-Fe2O3 NP hybrid nanomaterials with improved water solubility and functionality.
In this work, water-dispersible magnetite nanoparticles are produced by the self-assembly of amphiphilic copolymers containing poly(2-ethyl-2-oxazoline) as the hydrophilic block; poly(ɛ-caprolactone), as the hydrophobic segment, could self-assemble into micelles with oleic acid modified Fe3O4 nanoparticles. Hydrophobic poly(ɛ-caprolactone) (PCL) segments can hypothetically adsorb onto the pre-synthesized Fe3O4 nanoparticles coated with oleic acid, and hydrophilic (poly(2-ethyl-2-oxazoline) POX) blocks can protrude outward from the particle surface to provide steric stabilization and enhance the solubility in water. The presence of Fe3O4 nanoparticles encapsulated by polymeric micelles is confirmed by TEM.
2 Results and discussion
The synthesis and characterization of POX–PCL copolymers were performed following a literature procedure [21]. CH3I was able to initiate the ring opening polymerization of oxazoline, and polymers poly(2-ethyl-2-oxazoline) (POX) were obtained. The polymerization process was quenched using 0.1 N methanolic KOH, and the polymers containing hydroxyl end functional groups (POX–OH) were obtained. ɛ-Caprolactone was then polymerized using the hydroxyl groups of POX, and amphiphilic diblock copolymers containing POX, and PCL blocks were synthesized (Scheme 1).
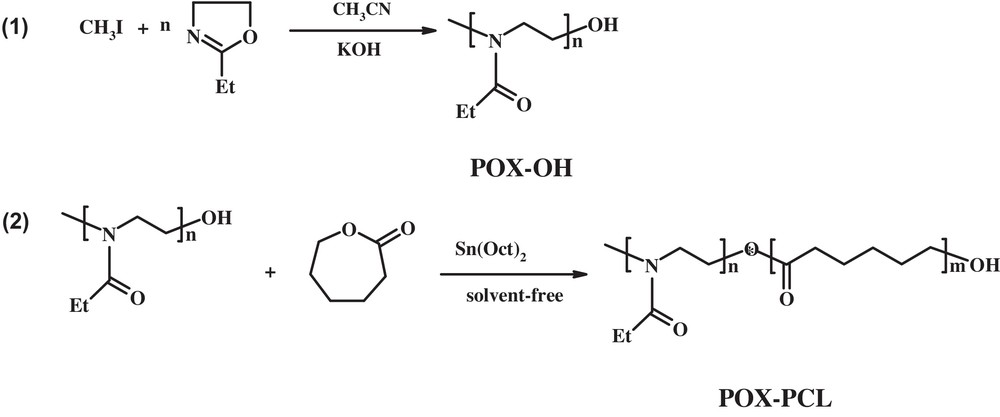
Synthesis of the poly(2-ethyl-2-oxazoline)-poly(ɛ-caprolactone) diblock copolymers.
The molecular weights and block compositions of the amphiphilic copolymers were determined by the analysis of the 1H NMR spectra in Fig. 1. The molar ratios of repeating units in POX were determined by the peak integration ratios of methyl protons CH3I (3 ppm) and COCH2CH3 (1 ppm). The molecular weights of polymers were of 3000 (Fig. 1a). The molar ratios of the repeating units in POX and PCL blocks were determined by the peak integration ratios of methyl protons (1.1 ppm) in POX and CH2OCO (4.05 ppm) in the PCL segment. Molecular weights of copolymers were of 4000 (Fig. 2a). The micelle formation of amphiphilic copolymers in an aqueous phase was confirmed by NMR spectroscopy. Fig. 1 shows the 1H NMR spectra of POX–PCL micelles in CDCl3 (a) and D2O (c) solvents. While the resonance peaks from both POX segment and PCL block were clearly observed in CDCl3, the peaks of the PCL segment disappeared in D2O, which indicated the limited molecular motion of the PCL block surrounded by the hydrophilic POX. This result confirms the formation of a micelle of POX–PCL diblock copolymers in aqueous phase [21].
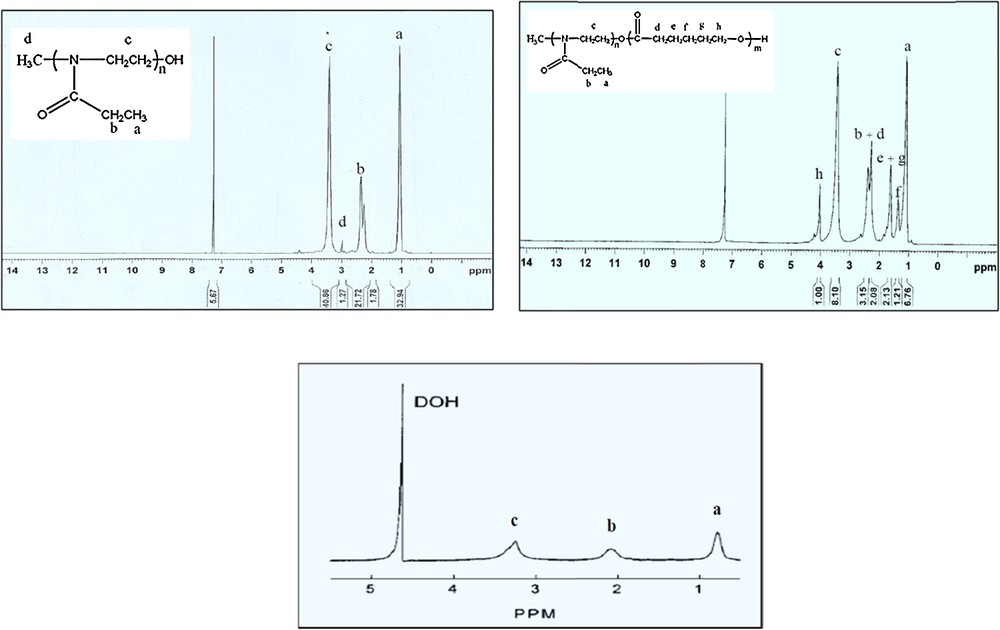
1H NMR spectra of (a) POX polymer, POX–PCL copolymers (b) in CDCl3, and POX–PCL copolymers in D2O.
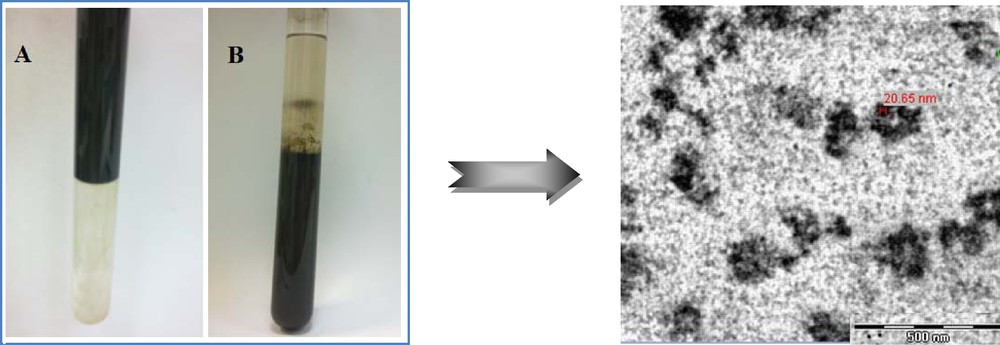
In this work, we report a synthetic method of water dispersible magnetite nanoparticles having oleic acid and poly(2-ethyl-2-oxazoline)-poly(ɛ-caprolactone) diblock copolymer. The Fe3O4 nanoparticles were prepared by coprecipitation and had bilayer surface with hydrophobic inner poly(ɛ-caprolactone) (PCL) layer and hydrophilic corona poly(2-ethyl-2-oxazoline) (POX) blocks.
After co-precipitation of the Fe3O4 nanoparticles, they were stabilized with oleic acid and dispersed in hexane. To transfer oleic acid-coated Fe3O4 nanoparticles to the water phase, a copolymer aqueous solution with a certain concentration was introduced into the magnetite dispersion in hexane. The hexane–water mixture was phase-separated under ultrasound irradiation (Fig. 2). Fig. 2 also shows that the water-dispersible magnetite nanoparticles stayed in the water phase for a week without being released to the organic phase. Hydrophobic PCL blocks aggregated in water and formed a micellar core, while hydrophilic POX segments dispersed in water and formed a micellar corona. Once the hexane–water mixture had been sonicated, the hydrophobic particles were transferred from the hexane phase to the aqueous one and were trapped into the micellar core due to a physisorption interaction between oleic acid and the hydrophobic PCL block [23].
The magnetite nanoparticles’ particle size was also investigated using transmission electron microscopy (TEM). A representative TEM image of a copolymer–magnetite complex dispersed in water with a 1.0 wt% copolymer concentration is displayed in Fig. 3. These nanoparticles with a diameter of 10–20 nm exhibit a spherical shape. However, the micelles formed by POX–PCL diblock copolymers could not be observed by TEM, a technique that was considered to be more sensitive to the electron-rich metal particles than copolymers. Similar phenomena were also observed in previous works [21].
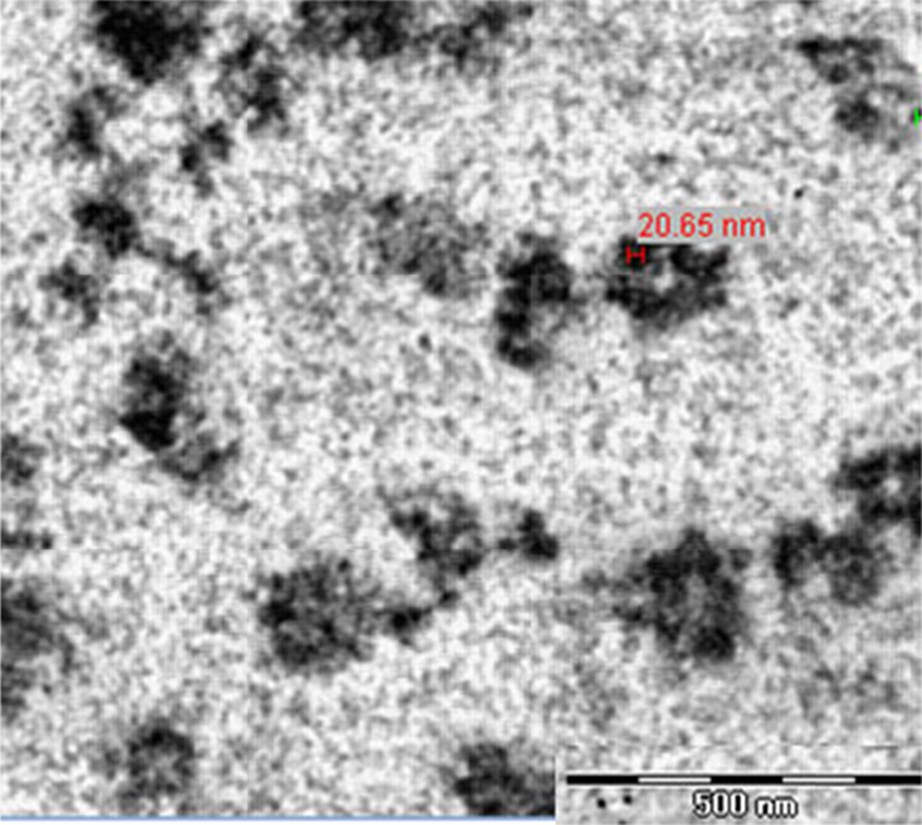
TEM image of the copolymer–magnetite complex in water.
The sonication duration of the water/hexane mixture of magnetite and copolymer also played a key role in the magnetic properties of the particles. Table 1 shows the percent of magnetite in the copolymer–magnetite complex and the Ms of the complex after ultrasonication for 1–4 h. The percent of magnetite in the complex ranged between 65 and 70% and did not significantly increase when ultrasonication durations were extended. This result indicates that the magnetic properties of the nanoparticles were influenced by ultrasonicating time periods; at least 2 h of sonication were required to obtain nanoparticles with a good magnetic response. It is hypothesized that relatively small nanoparticles migrated to the aqueous phase when only 1 h of sonication was applied. The particle size definitely influences their magnetic properties. Extension of the ultrasonication time might further enhance the phase-migration efficiency of the nanoparticles, resulting in the migration of large particles to the aqueous phase, and therefore, particles with high Ms values were obtained [23]. Representative hysteresis curves of dispersions 1 and 3 are displayed in Fig. 4.
Effect of the ultrasonication treatment's duration on the percent of magnetite in the complex and its magnetic properties.
Dispersion | Time of ultrasonicationa (h) | % Fe3O4 in copolymer–magnetite complexb | Msc (emu/g sample) |
1 | 1 | 65 | 20 |
2 | 2 | 68 | 29 |
3 | 3 | 70 | 33 |
4 | 4 | 69 | 31 |
a Ultrasonication duration of the mixture of 20 mL of 1 wt% of the copolymer and 20 mL of hexane, with 10 wt% of oleic acid.
b Determined from the aqueous layer of the water/hexane mixture. %Fe3O4 in the copolymer–magnetite complex determined by AAS.
c Calculated from the magnetic moment of the sample at 10,000 g.
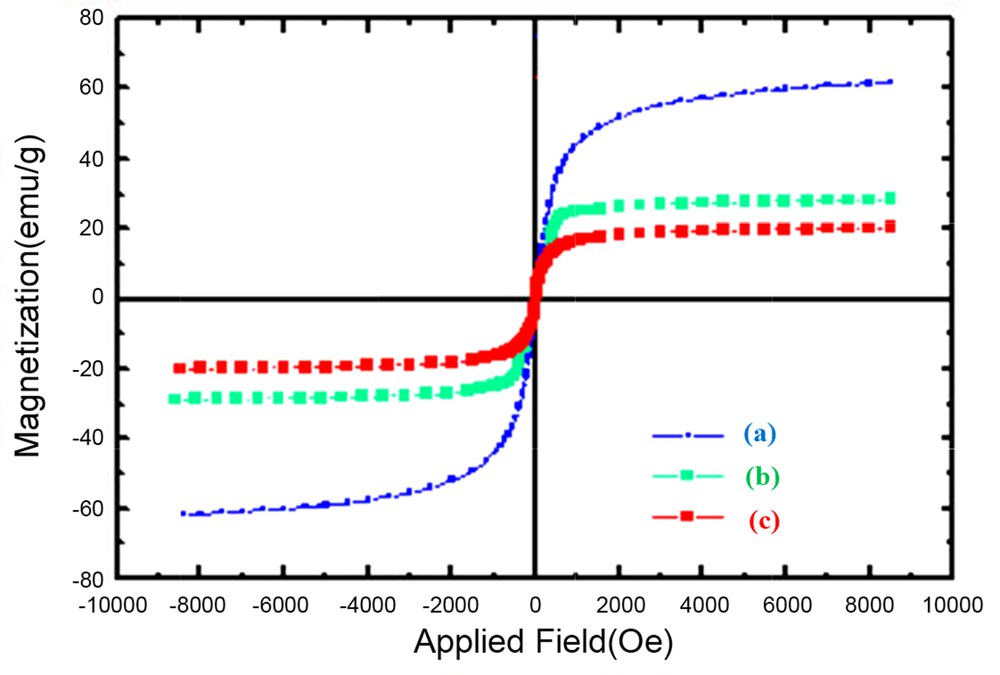
Representative hysteresis curves of (a) Fe3O4 nanoparticles and Fe3O4 nanoparticles of dispersions 3 (b) and 1 (c).
3 Conclusions
In summary, we demonstrated a successful preparation of water-dispersible magnetite nanoparticles using amphiphilic diblock POX–PCL copolymers in aqueous solution for the first time. These copolymers, containing POX and PCL blocks, could self-assemble to form bilayer stabilizers having a hydrophobic inner shell and a hydrophilic corona in aqueous solution. PCL was hypothesized to adsorb onto the particle surface coated with oleic acid, and POX hydrophilic blocks extended to the carrier fluid to provide stabilization. It is important to note that the durations of ultrasonication of the water/hexane mixture of magnetite and diblock copolymer played a key role in the magnetic properties of the particles.
4 Experimental
4.1 Materials
High-purity chemical reagents were purchased from Merck and Sigma. All the materials were of commercial reagent grade. FT–IR spectra were obtained with potassium bromide pellets in the 400–4000 cm−1 range with a PerkinElmer 550 spectrometer. 1H NMR and 13C NMR spectra were recorded with a Bruker DRX-400 spectrometer at 400 and 100 MHz, respectively. NMR spectra were obtained in CDCl3 as a solvent and are reported in parts per million (ppm) downfield from tetramethylsilane as an internal standard. The abbreviations used are: singlet (s), doublet (d), triplet (t), and multiplet (m). The particle size and morphology were investigated using a JEOL JEM-2010 transmission electron microscope (TEM) with an accelerating voltage of 200 kV. Dynamic light-scattering experiments were performed with a commercially available equipment (Zetasizer Nano from Malvern) with a 4-mW He–Ne laser (633 nm wavelength) and a fixed detector angle of 173°. The molecular weight distributions were determined by size exclusion chromatography (SEC) (Agilent 1100) using a PSS GRAL 100 Å column connected with a differential refractometer, with an RI detector with chloroform as the mobile phase at 25 °C. Polystyrene standard samples were used for calibration. GC-mass experiments were recorded using a Shimadzu GCMS-QP 505 A with a DB5 column.
4.2 Preparation of poly(2-ethyl-2-oxazoline) (POX–OH)
Poly(2-ethyl-2-oxazoline) (POX) was prepared by cationic polymerization according to the procedure reported elsewhere [21]. In brief, 2.6 mL (25.6 × 10−3 mol) of 2-ethyl-2-oxazoline and 0.1 mL (1.6 × 10−3 mol) of iodomethane were added to 30 mL of dried acetonitrile. After stirring for 72 h, at 110 °C, the polymerization was stopped by pouring methanolic KOH into the reaction mixture at room temperature, The poly(2-ethyl-2-oxazoline) was purified by precipitation in diethyl ether and then, dried under vacuum at 50 °C for 1 h. The reaction yield was 85%.
4.3 Preparation of poly(2-ethyl-2-oxazoline)-poly(ɛ-caprolactone) copolymers (POX–PCL)
About 1 mL of Sn(Oct)2 in toluene (1 × 10−3 M) was added to a polymerization ampoule equipped with a magnetic stirrer and a vacuum inlet. Toluene was evaporated under vacuum at 60 °C for 30 min. About 1.33 mL of ɛ-caprolactone and 1.42 g of POX–OH as the macroinitiator were added to the polymerization ampoule, which was left under vacuum for 1 h at 60 °C. The polymerization ampoule was sealed and stirred at 120 °C for 12 h. Then, it was cooled and the ampoule's contents were dissolved in chloroform. The solution was filtered and the product was precipitated in diethylether. The yield of reaction was 90%.
4.4 Preparation of the copolymer-stabilized aqueous-based magnetite nanoparticles
Fe3O4 nanoparticles were prepared using the chemical co-precipitation method described in the literature [22]. Typically, 20 mmol of FeCl3·6H2O and 10 mmol of FeCl2·4H2O were dissolved in 75 mL of distilled water in a three-necked round-bottom flask (250 mL) under N2 atmosphere for 30 min. After rapid mechanical stirring, 10 mL of NaOH (10 M) were added into the solution within 30 min with vigorous mechanical stirring and ultrasound treatment under continuous N2 atmosphere bubbling. After being rapidly stirred for 1 h, the resultant black dispersion was heated to 85 °C for 1 h. The dispersion mixture was centrifuged at 5000 rpm for 20 min, and the aqueous supernatant was discarded. The oleic acid solution in hexane (10 wt% in 20 mL of hexane) was then introduced into the magnetite dispersion under stirring under N2 atmosphere. The dispersion was concentrated by evaporating hexane to obtain a black liquid of concentrated magnetite in organic medium. To prepare the nanoparticles stabilized on POX–PCL diblock copolymer, 20 mL of the dispersion in hexane were introduced into 20 mL of aqueous polymer solution (1 wt% of copolymers). Then, the mixtures were ultrasonicated for 1–4 h to transfer the nanoparticles from the hexane top layer to the aqueous bottom layer. Hexane was evaporated and the remaining dispersions were centrifuged at 5000 rpm for 20 min to remove large aggregates. The diblock copolymer–magnetite complex in water phase was dialyzed against deionized water and refreshed twice at 24 h interval to remove excess POX–PCL copolymers from the dispersions. Precise concentrations of magnetite in the dispersions were determined by atomic absorption spectroscopy (AAS).
Acknowledgments
We gratefully acknowledge the financial support from the Research Council of the University of Kashan for supporting this work by Grant No. 256722/II.