1 Introduction
Recently, two-dimensional nanostructured materials, such as nanotubes, nanorods and nanowires, have attracted considerable interest because of their unique physical properties and potential applications in optics, magnetics and electronics [1–3]. Semiconductor nanoparticles have prospective applications in many technological areas, including photo- and electroluminescent devices, light-emitting diodes, and photovoltaic devices [4–6]. Especially, due to a wide-band gap (bulk band gap = 3.6 eV), ZnS nanoparticles are good emitters of light in blue and ultraviolet ranges, so they have found many applications, for instance as phospors, solar cells, and infrared windows, as the properties of nanoparticles are highly dependent on their structure, size and size distribution, and morphology. Studies on the nanoparticle synthesis, growth, and microstructural evolvement play a critical role in controlling the size-dependent properties [7–9]. Recently, various methods have been developed to synthesize different structures of semiconductor nanoparticles, including sol–gel templates, thermolysis of metal complexes, solvothermal, micelle–template interface, reduction route, ion beam synthesis, ultrasonic irradiation in an aqueous solution, etc. [10–15]. Several groups have reported the synthesis of metal sulfide nanoparticles from the thermal decomposition of single source precursors using a conventional heating process [16–18]. The use of single source precursors containing both metal and chalcogenide source (metal–ligand complexes) has been extensively studied as an effective route to the synthesis of nanoparticles. The properties of the ligand in metal complexes used as precursors could be used in the modification of the size and shape of the nanoparticles. For example, a variation of the alkyl groups on the dithiocarbamate ligand was found to give particles with non-spherical morphologies [19].
In this work, we have used three different precursors, Zn(thqdtc)2, Zn(thqdtc)2(py), and Zn(thiqdtc)2(py) complexes as single source precursors for the synthesis of ZnS nanoparticles. This is the first report of the use of pyridine adducts of zinc-heterocyclicdithiocarbamates for the synthesis of ZnS nanoparticles. The single crystal X-ray structure of Zn(thqdtc)2 is also reported.
2 Experimental
2.1 General
1,2,3,4-Tetrahydroquinoline (Alfa Aesar), 1,2,3,4-tetrahydroisoquinoline (Sigma–Aldrich), carbon disulfide (Merck), pyridine (Merck), and solvents (sd fine) were commercially available high-grade materials and were used as received. The wide-angle X-ray diffraction (XRD) was recorded using a Philips X’pert Pro MPD Diffractometer. The diffraction pattern over the 2θ range of 10–80° was recorded at a scan rate of 5 s/step. TEM images were recorded using a Philips CM200 (operating voltages: 20–200 kV, resolution: 2.4 Å). Energy dispersive X-ray spectroscopy (EDAX) measurements were performed using a JEOL model JED-2300 instrument. A Shimadzu UV-1650 PC double beam UV–Visible spectrophotometer was used for recording the electronic spectra. The spectra were recorded in chloroform and the pure solvent was used as the reference. Fluorescence measurements were made using a Jasco FP-550 spectrofluorimeter.
2.2 X-Ray crystallography
Details of the crystal data, data collection and refinement parameters for the Zn(thqdtc)2 complex are summarized in Table 1. Suitable crystals were obtained through slow evaporation of a dichloromethane solution of Zn(thqdtc)2. The intensity data were collected at ambient temperature [293(2) K] on a Bruker AXS kappa apex2 CCD diffractometer using the graphite monochromated Mo Kα radiation (λ = 0.71073 Å). The structure was solved by SIR-92 [20] and refined by full-matrix least-square method with SHELXL-97 [21]. All non-hydrogen atoms were refined anisotropically and hydrogen atoms were refined isotropically. Selected bond distances and angles are given in Table 2.
Crystal data, data collection and refinement parameters for [Zn(thqdtc)2].
Empirical formula | C20H20N2S4Zn |
FW | 481.99 |
Crystal dimensions (mm) | 0.30 × 0.20 × 0.16 |
Crystal system | Triclinic |
Space group | |
a/Å | 7.636(5) |
b/Å | 7.637(4) |
c/Å | 17.681(3) |
α/° | 85.863(4) |
β/° | 83.604(3) |
γ/° | 86.855(7) |
U/Å3 | 1020.8(9) |
Z | 2 |
DC/g cm−3 | 1.568 |
μ/cm−1 | 1.620 |
F(0 0 0) | 4 9 6 |
λ/Å | Mo Kα (0.71073) |
θ range/° | 1.16 − 25.00 |
Index ranges | −9 ≤ h ≤ 9, −9 ≤ k ≤ 9, −21 ≤ l ≤ 20 |
Reflections collected | 3584 |
Observed reflections Fo > 4′O(Fo) | 3253 |
Weighting scheme | Calc. W = 1/(σ2(Fo2) + (10.1061p)2 + 7.548p) where p = (Fo2 + 2Fc2)/3 |
Number of parameters refined | 245 |
Final R, Rw(obs, data) | 0.0257, 0.0302 |
GOOF | 1.122 |
Bond distances (Å) and angles (°) for [Zn(thqdtc)2].
Bond distances (Å) | Bond angles (°) | ||
S1–Zn1 | 2.3024(9) | S1–Zn1–S3 | 128.84(3) |
S2–Zn1 | 2.3530(12) | S1–Zn1–S4 | 127.54(3) |
S3–Zn1 | 2.3316(11) | S3–Zn1–S4 | 77.87(2) |
S4–Zn1 | 2.3450(17) | S1–Zn1–S2 | 78.37(3) |
C19–N2 | 1.436(3) | S3–Zn1–S2 | 123.76(3) |
C20–N2 | 1.327(3) | S4–Zn1–S2 | 128.15(3) |
C20–S3 | 1.720(2) | C10–S2–Zn1 | 81.48(8) |
C20–S4 | 1.720(2) | C20–S3–Zn1 | 82.46(8) |
2.3 Preparation of Zn(thqdtc)2 and its pyridine adduct
2.3.1 Preparation of Zn(thqdtc)2
An aqueous solution of ZnSO4·7 H2O (10 mmol, 2.9 g) was added dropwise to an aqueous solution of Na(thqdtc)·2H2O at the temperature of ice cold water. The reaction mixture was stirred for 1 h and the precipitate was filtered. The product was washed several times with cold water and then dried [22]. The yield was 72%.
2.3.2 Preparation of Zn(thqdtc)2(py)
Zn(thqdtc)2 (1 mmol, 0.48 g) was dissolved in 50 mL of warm pyridine. The yellow solution obtained was filtered and kept for evaporation. After a few days, both powder and single crystals suitable for X-ray structural analysis were obtained [23] (Scheme 1). The yield was 65%.

Preparation of Zn(thqdtc)2 and Zn(thqdtc)2(py).
2.4 Preparation of Zn(thiqdtc)2 and its pyridine adduct
2.4.1 Preparation of Zn(thiqdtc)2
An aqueous solution of ZnSO4·7 H2O (10 mmol, 2.9 g) was added dropwise to an aqueous solution of Na(thiqdtc)·2 H2O (20 mmol, 5.34 g) at the temperature of ice cold water. The reaction mixture was stirred for 1 h and the precipitate was filtered. The product was washed several times with cold water and then dried [22]. The yield was 68%.
2.4.2 Preparation of Zn(thiqdtc)2(py)
Zn(thiqdtc)2 (1 mmol, 0.48 g) was dissolved in 50 mL of warm pyridine. The yellow solution obtained was filtered and kept for evaporation. After a few days, both powder and single crystals suitable for X-ray structural analysis were obtained [23] (Scheme 2). The yield was 71%.

Preparation of Zn(thiqdtc)2 and Zn(thiqdtc)2(py).
2.5 Preparation of ZnS nanoparticles
2.5.1 Preparation of ZnS1
An amount of 0.5 g of Zn(thqdtc)2 was dissolved in 15 mL of triethylenetetramine in a flask and then heated to reflux (267 °C) and maintained at this temperature for 2 min. The white precipitate obtained was filtered off and washed with ethanol. The yield was 0.075 g.
2.5.2 Preparation of ZnS2
A method similar to that described for the synthesis of ZnS1 was adopted; however, 0.5 g of Zn(thqdtc)2(py) was used instead of Zn(thqdtc)2. A white precipitate of nanoparticles was obtained. The yield was 0.066 g.
2.5.3 Preparation of ZnS3
A method similar to that described for the synthesis of ZnS1 was adopted; however, 0.5 g of Zn(thiqdtc)2(py) was used instead of Zn(thqdtc)2. A white precipitate of nanoparticles was obtained. The yield was 0.070 g.
3 Results and discussion
3.1 Characterization of zinc sulfide nanoparticles
3.1.1 Powder X-ray diffraction analysis
To study the crystalline structures of the products, XRD measurements were carried out at room temperature. The ZnS nanoparticles synthesized from Zn(thqdtc)2, Zn(thqdtc)2(py), and Zn(thiqdtc)2(py) are represented as ZnS1, ZnS2 and ZnS3, respectively. Fig. 1 shows the XRD patterns of the ZnS1, ZnS2 and ZnS3, in which the diffraction peaks marked by “*”, “+” and “#” match well cubic (JCPDS Card No: 05-0566), hexagonal (JCPDS Card No: 12-0688) and rhombohedral ZnS (JCPDS Card No: 83-1700) phases, respectively. By examining the whole X-ray diffraction patterns, it is concluded that these nanoparticles contain a mixture of cubic, hexagonal, and rhombohedral phases.

XRD patterns of (a) ZnS1, (b) ZnS2, and (c) ZnS3.
Triethylenetetramine is a strong chelating neutral ligand. It forms a hexacoordinated intermediate complex (Fig. 2) with Zn(thqdtc)2. Zn(thqdtc)2(py) also forms the same intermediate complex (Fig. 2) and gives free pyridine. The intermediate complex formed from Zn(thqdtc)2 and Zn(thqdtc)2(py) depletes at higher temperature (267 °C) when the nucleation process starts. In the powder X-ray diffraction pattern, sharp (0 0 36), (0 0 39), (0 0 42) (rhombohedral phase) peaks are observed for ZnS2 compared to those in ZnS1, indicating that free pyridine in the intermediate stage may bind to the planes of hexagonal and cubic phases of the nucleus, leaving the (0 0 36), (0 0 39), (0 0 42) (rhombohedral phase) more exposed for ZnS deposition in ZnS2.

Proposed structure for the intermediate complex.
3.1.2 Energy dispersive X-ray (EDAX) analysis
Examination by EDAX of ZnS1, ZnS2 and ZnS3 confirms the formation of ZnS. The EDAX spectra of ZnS1, ZnS2 and ZnS3 are shown in Fig. 3. EDAX reveals the presence of zinc and sulfur in all cases. C, N and O signals are also observed in the energy dispersive X-ray spectra of all the as-synthesized zinc sulfides. The presence of C and N peaks in the spectrum evidences the presence of triethylenetetramine in the product. A relatively weak oxygen peak in both spectra probably originates from an unavoidable surface adsorption of oxygen to the zinc sulfide particles when exposed to air during sample processing.

EDAX spectra of (a) ZnS1, (b) ZnS2, and (c) ZnS3.
3.1.3 Transmission electron microscopy (TEM) studies
The dimensions and morphologies of the zinc sulfides were studied by TEM measurements. TEM images of ZnS1, ZnS2 and ZnS3 are shown in Fig. 4. The results of the TEM studies are summarized in Table 3. The TEM images show that two types of morphologies are present in ZnS1 and ZnS2. The TEM images of ZnS1 and ZnS2 show that the particles are sheet- and cylinder-like structures. The TEM image of the ZnS3 sample shows that the nanoparticles are partly interconnected, whereas each individual particle possesses an irregular shape. The length of the sheets and cylinders in ZnS2 are longer than those in ZnS1. This may be due to the presence of pyridine in the precursor (Zn(thqdtc)2(py)). The morphology of ZnS3 is different from those of ZnS1 and ZnS2. This indicates that the dithiocarbamate ligands in metal complex (precursor) affect the shape and size of the nanoparticles.

TEM images of (a) ZnS1, (b) ZnS2, and (c) ZnS3.
Sizes of ZnS1 and ZnS2 nanoparticles estimated from TEM analysis.
ZnS nanoparticles | Sheet | Cylinder | ||
Width (nm) | Length (nm) | Diameter (nm) | Length (nm) | |
ZnS1 | 69–155 | 122–273 | 90–108 | 137–232 |
ZnS2 | 127–200 | 127–339 | 39–138 | 214–465 |
3.1.4 Optical properties
To investigate the optical properties of the obtained ZnS nanoparticles, the room temperature UV–vis absorption and fluorescence spectra were recorded. The UV–visible absorption spectra of ZnS nanoparticles prepared from different precursors are shown in Fig. 5. Absorption maxima were observed at 250 (4.96 eV), 240 (5.17 eV), and 245 nm (5.06 eV) for ZnS1, ZnS2 and ZnS3, respectively. The absorption peaks are significantly blue-shifted relative to bulk ZnS (344 nm) [24], indicating quantum confinement.

Absorption spectra of (a) ZnS1, (b) ZnS2, and (c) ZnS3.
The fluorescence spectra of the ZnS nanoparticles are shown in Fig. 6. The fluorescence spectra of ZnS1, ZnS2 and ZnS3 nanoparticles exhibit only one broad emission peak through the excitation at 350 nm. The broad emission peak around 440 nm was assigned to the electron–hole recombination from internal vacancies for Zn and S atoms. It is observed that there is a prominent blue shift of around 35 nm of the emission band compared with that of the bulk ZnS [25], which might be due to the quantum size effects of the ZnS nanocrystals.
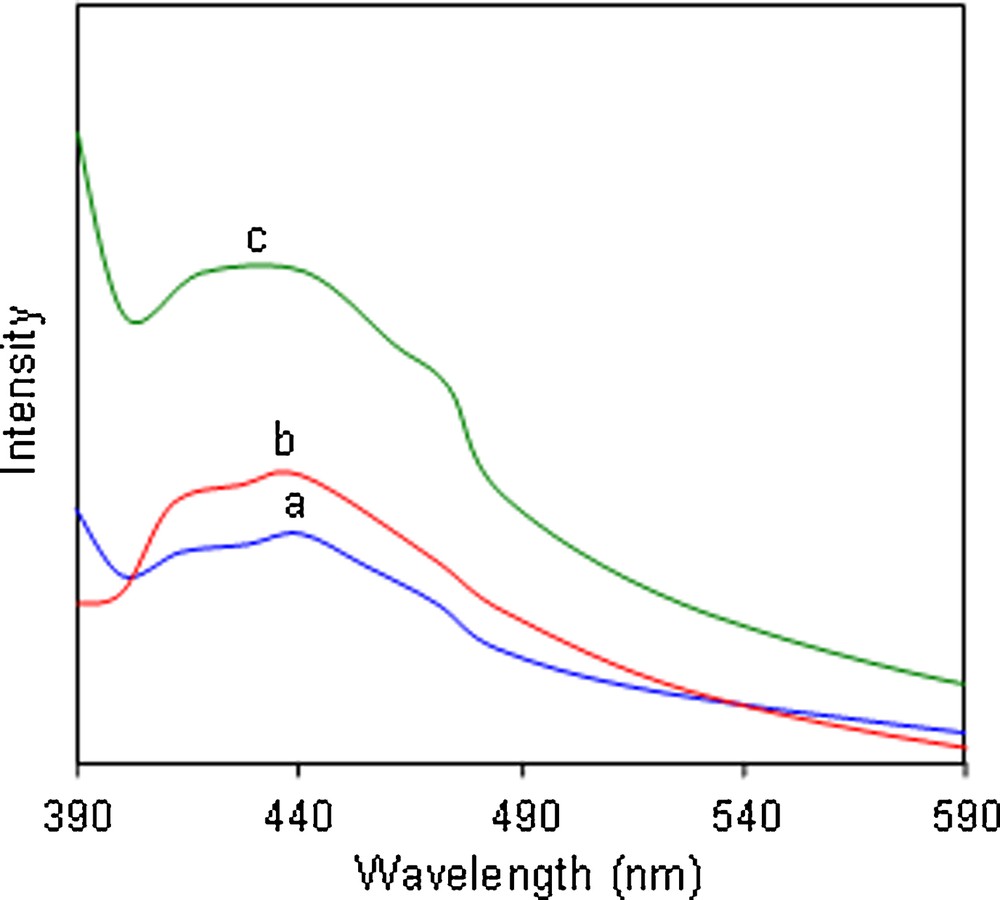
Fluorescence spectra of (λex = 350 nm): (a) ZnS1, (b) ZnS2, and (c) ZnS3.
3.2 Structural analysis of precursor Zn(thqdtc)2
The ORTEP diagram of Zn(thqdtc)2 is shown in Fig. 7. The Zn(thqdtc)2 complex is monomeric with two molecules per unit cell. The molecular structure of Zn(thqdtc)2 features two chelating dithiocarbamate ligands with Zn–S distances relatively narrow at 2.3024(9) to 2.3530(12) Å. The distorted tetrahedral coordination geometry is evidenced around the zinc atom as attested to by the S–Zn–S angles of 77.87(2) to 128.84(3)°. This complex represents an example of a monomeric structure of the zinc dithiocarbamates, which are usually dimeric [26]. The short thioureide C–N distance, 1.327(3) Å, indicates that the π electron density is delocalized over the S2CN moiety and that the bond has a significant double-bond character. The C–S distances [1.721(2) Å] are to a considerable extent shorter than the typical C–S single-bond distance 1.81 Å. Therefore, all the C–S bonds in the present structure possess the partial double bond character observed in most of the dithiocarbamates. The C–C and C–N bond distances associated with the tetrahydroquinoline moiety are normal. Thus, the overall solid-state structure is similar to that of the other known examples of monomeric binary zinc dithiocarbamates, viz., Zn(S2CN(Me)Cy)2 [27] and Zn(S2CN(CH2Ph)2)2 [28].

ORTEP diagram of precursor [Zn(thqdtc)2].
4 Conclusions
We reported here a simple and versatile method for the controlled synthesis of zinc sulfide nanoparticles using three new single source precursors in a single-pot and low-temperature process. Only triethylenetetramine (trien) has been used as a capping agent. The sheet and cylinder shape of ZnS nanoparticles were obtained from Zn(thqdtc)2 and Zn(thqdtc)2(py), whereas shapeless nanoparticles were obtained with Zn(thiqdtc)2(py). It is clear from these reactions that the ligands in the metal complexes affect the shape and size of the nanoparticles. This method may be extended to the fabrication of nanoparticles with various shapes and sizes exhibiting novel morphologies and properties using metal complexes containing various dithiocarbamate ligands as precursors.