1 Introduction
1,2-Diketones are very important structural moieties in numerous biologically interesting compounds and are broadly utilized for the construction of complex structures in organic synthesis. Among the numerous 1,2-diketones, benzil derivatives have received special attention, because of their practical applications in organic and pharmaceutical industry, such as photosensitive and synthetic reagents [1]. Benzil is extensively used as a substrate in benzylic rearrangements and also acts as a starting material for the synthesis of many heterocyclic compounds [2,3], exhibiting biological activity, such as anticonvulsant derivative dilantin [4,5]. Oxidation of benzoin into benzil is one of the most efficient and practical methods in organic synthesis. In general, the oxidation of benzoins has been accomplished using homogeneous and heterogeneous catalysts. The use of homogeneous reagents for the oxidation of benzoin limits their practical utilizations compared to heterogeneous catalysts in terms of product recovery, product selectivity, and environmental concerns [6,7]. For the oxidation of benzoin to benzil using a supported oxidant, such as ferric oxide–aluminium oxide [8], VOCl3 [9], silica-supported manganese dioxide [10], ammonium chlorochromate adsorbed on alumina [11] or silica [12], and chromium trioxide on Kieselghur [13] were exploited to overcome these difficulties. However, most of these systems show relatively low selectivity toward the desired products, or exhibit low activity. Therefore, the desired product selectivity still remains a challenge for the existing oxidation methods.
Carbon nanotubes (CNTs) with electrical, mechanical, thermal, and optical properties, high surface-to-volume ratio, structural flexibility, and chemical stability were recently expected to be an advanced support for catalysts [14]. More importantly, CNTs can also be used in composites with metallic or oxide nanoparticles to improve the catalytic performance of these particles [14]. CNTs can not only provide a support for anchoring well-dispersed nanoparticles and work as a highly conductive matrix for enabling good contact between them, but it can also effectively prevent the volume expansion/contraction and aggregation of nanoparticles during the catalytic process [15]. On the other hand, among all metal oxides, an extreme interest has been attached to Co3O4 because the carbon framework has a large surface area for finely dispersing Co3O4 nanoparticles, high electrical conductivity for providing electrical pathways, highly developed mesoporosity for facile diffusion of electrolyte, and electrochemical stability for long-term operation [16]. Co3O4 supported on CNTs are attracting significant attention owing to their wide applications as Schottky-junction diode [17], electrochemical capacitors [18], Li-ion battery anode applications [19], and catalyst [20]. To enhance the catalytic performance and high surface area, Co3O4 can be used with carbon nanotubes (CNTs) to form a composite material to be widely used as a catalyst support [18].
In this report, we describe a convenient and efficient selective oxidation of benzoin derivatives to the corresponding benzils using Co3O4–CNT nanocomposites under an air atmosphere. CNT and nanosized Co3O4 were successfully composited to catalyze this oxidation process. CNT serves to support the catalyst and provides a surface for the oxidation reaction to occur. Co3O4–CNTs are expected to show excellent catalytic activity owing to their nanoscale size and large surface area. Therefore, we would like to report, for the first time, the selective oxidation of benzoins to benzils using Co3O4–CNT nanocomposites (Scheme 1).

Oxidation of benzoin to benzil derivatives using the Co3O4–CNT nanocomposite.
2 Experimental
2.1 Materials and instrumentation
Chemicals were purchased from Merck without any further purification. IR spectra were recorded on KBr pellets using a Perkin–Elmer 781 spectrophotometer and an Impact 400 Nicolet FTIR spectrophotometer. 1H NMR (400 MHz) and 13C NMR (100 MHz) spectra were recorded with a Bruker DPX-400 Avance spectrometer. The melting points were determined by a Yanagimoto micro melting point apparatus. The purity determination of the substrates and reaction monitoring were accomplished by thin layer chromatography (TLC) on silica gel polygram SILG/UV 254 plates. MWNTs were purchased from Nanotech Port Co. (Taiwan). These MWNTs were produced via the chemical vapour deposition (CVD, or sometimes called catalytic pyrolysis) method. The outer diameter of CNTs was between 20 and 40 nm. XRD patterns were acquired on a Philips X’Pert, Netherlands, diffractometer equipped with a Cu Kα radiation (λ = 1.5406 Ǻ). Scanning electron microscopy (SEM) was performed on a FEI Quanta 200 SEM operated at a 20-kV accelerating voltage. The samples for SEM were prepared by spreading a small drop containing nanoparticles onto a silicon wafer and dried almost completely in the air at room temperature for 2 h, and then transferred onto SEM conductive tapes.
2.2 Preparation of CNT–Co3O4 nanocomposites
CNT–Co3O4 nanocomposites were prepared according to procedures reported in the literature [18]. In short, CNTs were dissolved in a mixture of 98% sulphuric acid and 70% nitric acid (volumetric ratio 3:1) at 50 °C under ultrasonication for 6 h. Then, MWCNTs were centrifuged and washed with distilled water, then dried in an oven at 100 °C for 24 h. After that, 0.5 g of Co(NO3)2·6H2O was dissolved into 40 mL of n-hexanol to form a red solution. Seventy milligrams of acid-treated MWNTs were dispersed in the red solution by sonication for 2 h. Then, this solution was refluxed at 140 °C for 10 h. After cooling to ambient temperature, the products were washed with cyclohexane repeatedly to remove any impurities and dried. The black powder was filtered and washed with ethanol and dried at 100 °C under a vacuum oven to obtain the CNT–Co3O4 composite as a catalyst.
2.3 General procedure for the oxidation of benzoins to benzils
To a mixture of benzoin (1 mmol) and CNT–Co3O4 catalyst (10 mol%) in a 100-mL flask, toluene (5 mL) was added. The reaction mixture was stirred at 100 °C in the atmospheric air. No device was used for pumping the air into the reaction solution. The completion of the reaction was monitored by TLC, visualized with ultraviolet light. After complete disappearance of the benzoin, as monitored by TLC (petroleum ether:ethyl acetate, 9:2), the reaction mixture was filtered while the residue was washed with hot toluene (2 × 5 mL) and water (3 × 15 mL). The product was obtained after removal of the solvent under reduced pressure, followed by crystallization. The catalyst was recovered from the residue after washing with EtOH (10 mL) followed by distilled water (200 mL) and then with acetone (15 mL). It can be re-used after drying at 80 °C for 2 h. The structures of these compounds have been investigated using different methods of spectrometry: IR, 1H NMR, 13C NMR, CHN, and UV.
3 Results and discussion
The oxidative functionalization of CNTs is important for enhancing chemical activity and depositing metal oxide nanoparticles as supports for heterogeneous catalysis applications [21]. It is known that after oxidation with sulphuric acid and nitric acid, the surface of MWNTs possesses a great deal of functional carboxyl groups and becomes negatively charged [18]. The positive cobalt ions in the hydrophobic n-hexanol solution are adsorbed on the surface of the acid-treated CNTs through electrostatic attraction and then in situ decompose into Co3O4 according to the following process [18]:
The formation of the CNT–Co3O4 nanocomposite was confirmed by X-ray diffraction (XRD); data are shown in Fig. 1. The crystalline peaks can be clearly indexed as a typical Co3O4 crystalline phase with a spinel structure (JCPDS card No. 74–1666) in Fig. 1b. The XRD patterns of the nanoparticles show six characteristic peaks, revealing a cubic cobalt oxide phase (2θ = 18.63, 26.22, 35.17, 43.55, 54.22, 60.08). These are related to their corresponding indices (4 1 1), (3 1 1), (4 0 0), (3 3 1), and (4 2 2), respectively. The broad peak located at approximately 2θ = 26.2° is characteristic of a CNT (Fig. 1a and b, JCPDS card No. 75–1621) [22].

XRD patterns of (a) the CNT and (b) the CNT–Co3O4 nanocomposite.
SEM images, presented in Fig. 2, allow the non-structural characterization of the CNT and Co3O4–carbon nanotube nanocomposite samples. The average diameter of carbon nanotubes was found to be 20 nm, as obvious from Fig. 2a. The size distribution of Co3O4 nanoparticles attached to the CNTs was found to be 48 nm of diameter, which illustrates that it is larger than that of MWNT. SEM images suggest that the Co3O4 nanoparticles are mostly attached to the carbon nanotube. The weight ratio of the CNT to the Co3O4 crystalline phase was 70:30, as determined by inductively coupled plasma atomic emission spectroscopy analysis.
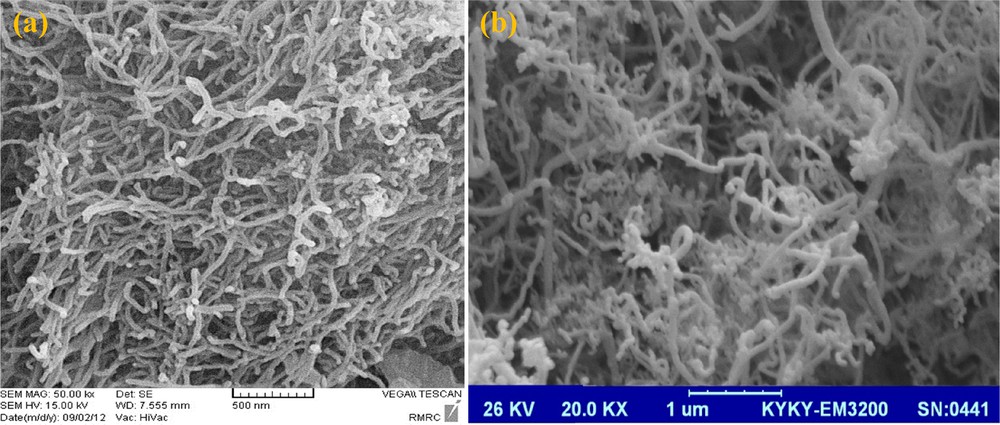
SEM images of the CNTs (a) and the Co3O4–MWCNT nanocomposites.
After the characterization of the nanocatalyst, we focused on the systematic evaluation of different catalysts for examination of catalytic activity in aerobic oxidation of benzoin to benzil. The fact that no conversion was obtained carrying out a blank reaction (i.e. without catalyst) infers that the catalyst is necessary for this transformation. In the course of this study, CNT–Co3O4 was the most effective catalyst (Table 1) with higher activity and better-controlled selectivity because of a large specific surface area on support of CNT.
The effect of the variations of different catalysts for the oxidation of benzoin to benzil in liquid phase catalysisa.
Entry | Catalyst | Time (h) | Yield (%)b |
1 | No catalyst | 3 | – |
2 | MnO2 | 2 | 30 |
3 | Al2O3 | 2 | 20 |
4 | Co3O4 | 2 | 45 |
5 | CNT–Co3O4 | 1 | 65 |
a Reaction conditions: benzoin (1 mmol), catalyst (4 mol%), toluene (5 mL), stirring at 100 °C under an air atmosphere.
b Isolated yields.
To select the appropriate solvent, the reaction with test substrates was carried out in different solvents. The results were summarized in Table 2. The results show that the larger yield of benzil was achieved with toluene. When other solvents were used, no significant improvement in the yield was observed. It seems that in the oxidation procedure, the non-occurrence of reaction in other solvents may be due to the poor solubility of the products in these solvents and the polarity of the latter. As shown in Table 2, the best yield of product was achieved with the catalyst ratio 10 mol% (Table 2, Entry 9).
The effect of amount of catalyst and solvent on the yield of the oxidation of benzoina.
Entry | Nanocatalyst (mol%) | Solvent | Time (h) | Yield (%)b |
1 | 4 | Toluene | 1 | 65 |
2 | 4 | CCl4 | 3 | 45 |
3 | 4 | CH2Cl2 | 3 | 40 |
4 | 4 | CH3OH | 5 | – |
5 | 4 | EtOH | 5 | – |
6 | 4 | H2O | 5 | – |
7 | 6 | Toluene | 1 | 75 |
8 | 8 | Toluene | 1 | 85 |
9 | 10 | Toluene | 1 | 95c |
10 | 12 | Toluene | 1 | 95 |
a Reaction conditions: benzoin (1 mmol), solvent (5 mL), stirring at 100 °C under air atmosphere.
b Isolated yields (Conversion of benzoin).
c The best yield of product.
This reaction is heterogeneous, taking place at the Co3O4–CNT nanocomposite. On the other hand, the nanocatalyst Co3O4 with high surface area on the CNT can lead to higher performance of the catalyst compared to the other catalysts. Moreover, the high efficiency of the nanoparticle oxides not only is caused by their high surface area but also it prevents agglomeration and therefore enhances their performances. Whatever the amount of catalyst, there is more oxygen adsorbed on the catalyst's surface, and the reaction is carried out efficiently.
Among the various oxidants (H2O2, m-perchlorobenzoic acid, and air), it was concluded that the best activity and selectivity could be achieved by air itself under optimized reaction conditions (Table 3). Moreover, it was found that the air present inside the reaction mixture itself is enough to derive this reaction.
The effect of variation of different oxidant for the oxidation of benzoin to benzila.
Entry | Oxidants | Yield (%)b |
1 | H2O2 | 30 |
2 | m-perchlorobenzoic acid | 50 |
3 | TBHP | 20 |
4 | Air atmosphere | 95 |
a Reaction conditions: benzoin (1 mmol), catalyst (10 mol%), toluene (5 mL), stirring at 100 °C
b Isolated yields (conversion of benzoin).
As shown in Table 4, in this reaction, the oxidation of benzoins by CNT–Co3O4 was carried out in good yield. From the results in Table 4, it seems that the benzoins containing an electron-donating group were found to be more reactive and could be oxidized more easily (compounds b and f). In contrast, the benzoins containing an electron-withdrawing group have shown a lower reactivity (compound j). In this reaction, benzil was obtained as the major product. Small amounts of C–C cleavage products, benzaldehyde, and benzoic acid were also detected.
Selective oxidation of benzoins in the presence of CNT–Co3O4 under an air atmospherea.
Entry | R1 | R2 | Product | Time (h) | Conversion of benzoin (%) | Selectivity of benzil (%) | mprep/mplit. (°C) |
1 | H | H | a | 1 | 95 | 85 | 93–95/94–96 [23] |
2 | 4-OCH3 | 4-OCH3 | b | 0.90 | 92 | 78 | 133–135/132–134 [23] |
3 | 4-NCH3 | H | c | 1.20 | 70 | 60 | 116–118/115–116 [23] |
4 | 4-CH3 | 4-CH3 | d | 0.60 | 85 | 65 | 102–104/101–104 [23] |
5 | 4-OCH3 | H | e | 0.90 | 91 | 75 | 65–67/62–63 [23] |
6 | NC5H5 | NC5H5 | f | 0.85 | 93 | 80 | 119–121 |
7 | 2,4-diOCH3 | 2,4-diOCH3 | g | 1.25 | 85 | 68 | 195–197 |
8 | H | 3-OCH3 | h | 0.90 | 67 | 50 | 133–134/133 [23] |
9 | H | 4-CH3 | i | 1 | 88 | 70 | 27–29/31 [23] |
10 | 4-CH3 | 3-Br | j | 1.20 | 60 | 40 | 141–142 |
a Reaction conditions: benzoin (1 mmol), catalyst (10 mol%), toluene (5 mL), and 100 °C was the reaction temperature.
After completion of the reaction, the catalyst was re-used for the next cycle without any appreciable loss of its activity. Similarly, reusability for sequential reaction was also carried out and the catalyst was found to be reusable for five cycles (Fig. 3).
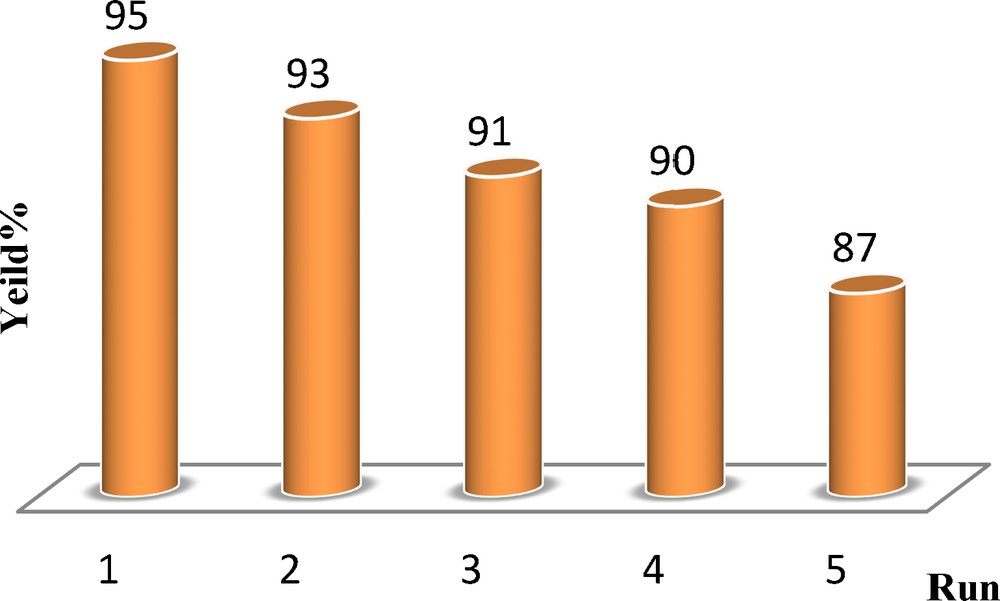
Recyclability of CNT–Co3O4 in the reaction of benzoin (1 mmol), catalyst (10 mol%), toluene (5 mL), under stirring at 100 °C in the air.
4 Spectroscopic data
4.1 Compound (a), benzil
UV (CH3OH) λmax: 224 nm; 1H NMR (CDCl3, 400 MHz) δ (ppm) 7.54 (t, 2H, CH, J = 7.6 Hz), 7.69 (t, 4H, CH, J = 7.6 Hz), 7.98 (d, 4H, CH, J = 7.6 Hz); 13C NMR (CDCl3, 100 MHz) δ (ppm) 128.90 (4CH), 129.70 (4CH), 133 (2CH), 134.93 (2C), 194.30 (2CO); IR (KBr) cm−1 1650 (CO, s), 1450, 1580 (CC, m), 720 (CH, m); Anal. calcd. (%) C, 79.99; H, 4.79; O, 15.22; found C, 79.91; H, 4.73; O, 15.12.
4.2 Compound (b), 4,4′-dimethoxybenzil
UV (CH3OH) λmax: 225 nm; 1H NMR (CDCl3, 400 MHz) δ (ppm) 3.89 (s, 6H, OCH3), 6.97 (d, 4H, CH, J = 8.8 Hz), 7.95 (d, 4H, CH, J = 8.8 Hz); 13C NMR (CDCl3, 100 MHz) δ (ppm) 55.62 (2OCH3), 114.29 (4CH), 126.19 (4CH), 132.30 (2C), 64.86 (2C), 193.54 (2CO); IR (KBr) cm−1 1650 (CO, s), 1586 (CC, m), 769 (CH, m); Anal. calcd. (%): C, 71.10; H, 5.22; O, 23.68; found: C, 70.98; H, 5.19; O, 23.64.
4.3 Compound (c), 4-dimethylaminobenzil
UV (CH3OH) λmax: 229 nm; 1H NMR (CDCl3, 400 MHz) δ (ppm) 3.38 (s, 6H, CH3), 6.67 (d, 2H, CH, J = 5.2 Hz), 7.48 (d, 2H, CH, J = 8.1 Hz), 7.61 (d, 2H, CH, J = 8.1 Hz), 7.8 (d, H, CH, J = 8.1 Hz), 8.28 (d, 2H, CH, J = 5.2 Hz); 13C NMR (CDCl3, 100 MHz) δ (ppm) 55.91 (2CH3), 111.20 (2CH), 128.90 (2CH), 129.01 (C), 129.70 (2CH), 135.37 (CH), 135.50 (C), 141.47 (C), 153.76 (C), 196.72 (CO); IR (KBr) cm−1 1650, 1700 (CO, s), 1440, 1620 (CC, m), 705 (CH, m); Anal. calcd. (%) C, 75.87; H, 5.97; N, 5.53; O, 12.63; found C, 75.82; H, 5.95; N, 5.50; O, 12.61.
4.4 Compound (d), 4,4′-dimethylbenzil
UV (CH3OH) λmax: 225 nm; 1H NMR (CDCl3, 400 MHz) δ (ppm) 2.45 (s, 6H, CH3), 7.4 (d, 4H, CH, J = 7.2 Hz), 7.75 (d, 4H, CH, J = 7.6 Hz); 13C NMR (CDCl3, 100 MHz) δ (ppm) 21.95 (2CH3), 129.72 (4CH), 130.03 (4CH), 130.69 (2C), 146.11 (2C), 194.54 (2CO); IR (KBr) cm−11650, (CO, s), 1586 (CC, m), 769 (CH, m); Anal. calcd. (%) C, 80.65; H, 5.92; O, 13.43; found C, 80.63; H, 5.90; O, 13.40.
4.5 Compound (e), 4-methoxybenzil
UV (CH3OH) λmax: 228 nm; 1H NMR (CDCl3, 400 MHz) δ (ppm) 3.76 (s, 3H, OCH3), 6.82 (2H, d), 7.34–7.61 (5H, m) 7.86 (2H, d); 13C NMR (CDCl3, 100 MHz) δ (ppm) 55.19 (CH3), 113.20 (2CH), 128.90 (2CH), 129.70 (2CH), 130.30 (C), 131.37 (CH), 133 (2CH), 133.26 (C), 164.69 (C), 194.30 (CO); IR (KBr) cm−1 1650, (CO, s), 1586 (CC, m), 769 (CH, m); Anal. calcd. (%) C, 74.99; H, 5.03; O, 19.98; found C, 74.91; H, 4.98; O, 19.96.
4.6 Compound (f), 2-pyridil
UV (CH3OH) λmax: 365 nm; 1H NMR (CDCl3, 400 MHz) δ (ppm) 7.49 (t, 2H, CH, J = 8.1 Hz), 7.91 (t, 2H, CH, J = 8.1 Hz), 8.18 (d, 2H, CH, J = 8.1 Hz), 8.56 (d, 2H, CH, J = 8.1 Hz); 13C NMR (CDCl3, 100 MHz) δ (ppm) 122.27 (2CH), 127.99 (2CH), 137.26 (2CH), 149.46 (2CH), 151.65 (2C), 197.02 (2CO); IR (KBr) cm−1 1713 (CO, s), 1274 (CN, s), 1505 (CC, m); Anal. calcd. (%) C, 67.92; H, 3.80; N, 13.20; O, 15.08; found C, 67.90; H, 3.78; N, 13.10; O, 15.04.
4.7 Compound (g), 2,2′,4,4′-tetramethoxybenzil
UV (CH3OH) λmax: 226 nm; 1H NMR (CDCl3, 400 MHz) δ (ppm) 3.88 (s, 3H, OCH3), 3.90 (s, 3H, OCH3), 6.44 (s, 2H, CH), 6.53 (d, 2H, CH, J = 8.4 Hz), 7.81 (d, 2H, CH, J = 8.8 Hz); 13C NMR (CDCl3, 100 MHz) δ (ppm) 55.23 (OCH3), 55.80 (OCH3), 101.58 (2CH), 113.03 (2CH), 116.91 (2C), 131.22 (2CH), 161.83 (2C), 162.93 (2C), 186.91 (2CO); IR (KBr) cm−1 1630–1680 (CO, s), 1470, 1618 (CC, m), 738 (CH, m); Anal. calcd. (%) C, 65.45; H, 5.49; O, 29.06; found C, 65.41; H, 5.43; O, 29.01.
4.8 Compound (h), 3-methoxybenzil
UV (CH3OH) λmax: 234 nm; 1H NMR (CDCl3, 400 MHz) δ (ppm) 3.72 (s, 3H, OCH3), 7.01 (1H, t), 7.1 (1H, s) 7.32 (1H, d), 751 (2H, t), 7.60 (1H, t), 7.72 (2H, d); 13C NMR (CDCl3, 100 MHz) δ (ppm) 54.00 (OCH3), 116.80 (CH), 120.13 (CH), 124.16 (CH), 128.90 (2CH), 129.70 (2CH), 131.05 (CH), 131.37 (CH), 132.88 (C), 135.27 (C), 163.09 (C), 193.41 (CO), 194.30 (CO); IR (KBr) cm−11660, (CO, s), 1396, 1613 (CC, m), 725 (CH, m); Anal. calcd. (%): C, 74.99; H, 5.03; O, 19.98; found C, 74.95; H, 4.98; O, 19.94.
4.9 Compound (i), 4-methylbenzil
UV (CH3OH) λmax: 223 nm; 1H NMR (CDCl3, 400 MHz) δ (ppm) 2.34 (s, 3H, CH3), 7.3 (m, 2H), 7.35 (m, 2H), 7.42 (t, 1H, J = 8 Hz), 7.55 (t, 2H, J = 8, 7.2 Hz), 7.92 (d.d, 2H, J = 7.92, 1.2 Hz); 13C NMR (CDCl3, 100 MHz) δ (ppm) 22.06 (CH3), 122.71 (2CH), 130.12 (2CH), 130.28 (2CH), 131.18 (2CH), 132.54 (2CH), 133.17 (C), 133.83 (C), 147.02 (C), 193.95 (CO), 194.08 (CO); IR (KBr) cm−11640, 1680, (CO, s), 1420, 1630 (CC, m), 724 (CH, m); Anal. calcd. (%) C, 80.34; H, 5.39; O, 14.27; found C, 80.32; H, 5.35; O, 14.24.
4.10 Compound (j), 3-Bromo-4′-methylbenzil
UV (CH3OH) λmax: 227 nm; 1H NMR (CDCl3, 400 MHz) δ (ppm) 2.42 (s, 3H, CH3), 7.34 (t, 1H, CH), 7.61 (d, 1H, CH), 7.74 (d, 3H, 3CH), 8.04 (s, H, CH), 8.59 (s, 1H, CH), 8.65 (s, 1H, CH); 13C NMR (CDCl3, 100 MHz) δ (ppm) 55.65 (CH3), 144.44 (2CH), 123.21 (C), 129.12 (CH), 129.76 (C), 130.47 (2CH), 131.37 (CH), 132.44 (CH), 134.91 (CH), 137.46 (C), 165.18 (C), 192.04 (CO), 193.08 (CO); IR (KBr) cm−1 1640, 1700, (CO, s), 1420, 1620 (CC, m), 705, 730 (CH, m); Anal. calcd. (%) C, 56.45; H, 3.47; Br, 25.04; O, 15.04; found C, 56.43; H, 3.44; Br, 25.01; O, 15.01.
5 Conclusions
In conclusion, the oxidation of benzoin was carried out using the CNT–Co3O4 composite under milder reaction conditions. CNT–Co3O4 is an effective catalyst in the oxidation of benzoin to benzil. Very high activity and selectivity of the product were obtained. Moreover, the mild reaction conditions, high yield of products, ease of work-up, and the selective procedure will make the present method a useful and important addition to the present methodologies for the oxidation of benzoins to the corresponding benzils.
Acknowledgement
We gratefully acknowledge the financial support from the Research Council of the University of Kashan for supporting this work by Grant No. 256722/V.