1 Introduction
Hydantoin and its derivatives are biologically important compounds of relevance to the chemists, biologists and pharmaceutical researchers. Hydantoins, substituted at C-5, are important medicinal compounds [1]. Numerous applications have been found for hydantoin derivatives due to their antidepressant [2] and antiviral activities [3], the inhibition of HIV binding to lymphocytes [4], as well as their anti-convulsant and cardiac anti-arrhythmic effects [5]. A number of in vivo studies have indicated that some biologically active compounds become more bacteriostatic and carcinostatic upon chelation [6]. From a structural point of view, hydantoins are a fruitful area for investigation since they possess an equal number of H-atom donors and acceptors and thus render a rich variety of intermolecular bonding, as well as different modes for coordination to metal ions. Moreover, it is well established that the type of the substituents at the 5th position in the hydantoin ring is of crucial importance for the pharmacological action of the corresponding compounds. Such interaction of transition metal ions with hydantoin derivatives is of immense biological importance [7]. It has been reported [8] that metal complexes of hydantoin derivatives Schiff bases with transition metals possess anticarcinogenic activity. For example, in contrast to free ligands, silver complexes with hydantoins are strong cytotoxic agents [8]. The structural characteristics of hydantoins and of their metal complexes are interesting with a view to developing novel drugs [9] and to better understanding the structure–activity relationship. Unfortunately, only few metal complexes with hydantoins are known in the literature [8,10–16], whereas no complex with 3-amino-5,5-dimethyl hydantoin of defined stoichiometry has been prepared to date. There are several literature reports devoted to the preparation of hydantoin “complexes” with copper and cobalt [17–19]. However, they do not contain defined biological activity characteristics. This the reason why, in this research, the preparation and the characterization of the physicochemical properties (IR, UV–Vis, voltammetry, and elemental analysis) of Cu(II) and Co(II) complexes with 3-amino-5,5-dimethylhydantoin have been investigated. The cytotoxic effects of these newly synthesized metal complexes were tested against a panel of human tumor cell lines.
2 Results and discussion
2.1 Synthesis
Ligand 3-amino-5,5-dimethylhydantoin was prepared as described previously [20]. This ligand was used for the preparation of two complexes (Scheme 1). In these complexes, the ligand was coordinated in dianionic form. The deprotonation of the ligand is facilitated by the appropriate pH of the reaction medium as a result of the presence of solutions of HCl, NaOH and of an ammonium buffer solution.

(Color online.) Synthesis of the complexes Cu(II)- and Co(II)-aminohydantoin.
The newly synthesized compounds were characterised by IR, UV–Vis spectroscopy and voltammetry (see Experimental section).
2.2 IR spectra
Our IR spectroscopy study confirms the structure of the above-mentioned compounds (Fig. 1). The spectrum of the complexes of Cu(II) with a hydantoin derivative obtained at different pH values are fully identical; this is why we can conclude that at pH 3 and pH 7, the same complex was formed between copper (II) and the hydantoin ligand. The comparative analysis of the IR spectra of the free hydantoin ligand and of the Cu(II) and Co(II) complexes revealed that the energies of ν(4CO) and ν(2CO) vibrations are changed upon coordination (Fig. 1). The absorption band related to the stretching vibrations ν(4CO) is observed at 1735 and 1730 cm−1 for complexes Cu(II)-aminohydantoin and Co(II)-aminohydantoin, respectively. The shift of the bands generated by the ν(CO) mode upon complexing means that they are involved in coordination. The ν(2CO) and ν(4CO) vibrations in 3-amino-5,5-dimetylhydantoin and other hydantoins cause the absorption observed in the ranges 1783–1779 cm−1 and 1740–1720 cm−1, respectively. The low intensities of these bands in Cu(II) and Co(II) complexes are probably caused by the hydrogen bonds between the carbonyl oxygen and the atoms of the water molecules coordinated to the metal ion. In the spectra of complexes 1 and 3, the broad absorption in the frequency range 3505–2980 cm−1 with a multiple structure of the bands is assigned to both the (N–H) and (C–H) stretching vibrations. The bands of complexes Cu(II)-aminohydantoin and Co(II)-aminohydantoin at 3359 cm−1 can be attributed to the ν(O–H) vibration of coordinated water molecules. Additionally, the δ(H2O) peak at ≈ 1607 cm−1 for complexes Co(II)-aminohydantoin and Cu(II)-aminohydantoin suggests the presence of a coordinated water molecule inside the coordination sphere. The presence of a strong band at 850 cm−1 in both complexes can be attributed to the rocking vibration ρ(H2O). The ν(M–O) vibrations are found at 510 and 511 cm−1 for complexes Cu(II)-aminohydantoin and Co(II)-aminohydantoin, respectively.

(Color online.) Comparison of the IR spectra of complexes of Cu(II) (red curve) and Co(II) (green curve) with 3-amino-5,5-dimethylhydantoin with the IR spectra of the free ligand.
2.3 UV–Vis spectra
The UV–Vis spectra of the Co(II)-aminohydantoin and of the free metal ion in water were recorded in the range from 200 to 900 nm (Fig. 2). The pink water solution of CoCl2·6H2O gave one spectral maximum at wavelength 518 nm. As can be seen (Fig. 2), one more spectral maximum at wavelength 480 nm appeared after chelation.
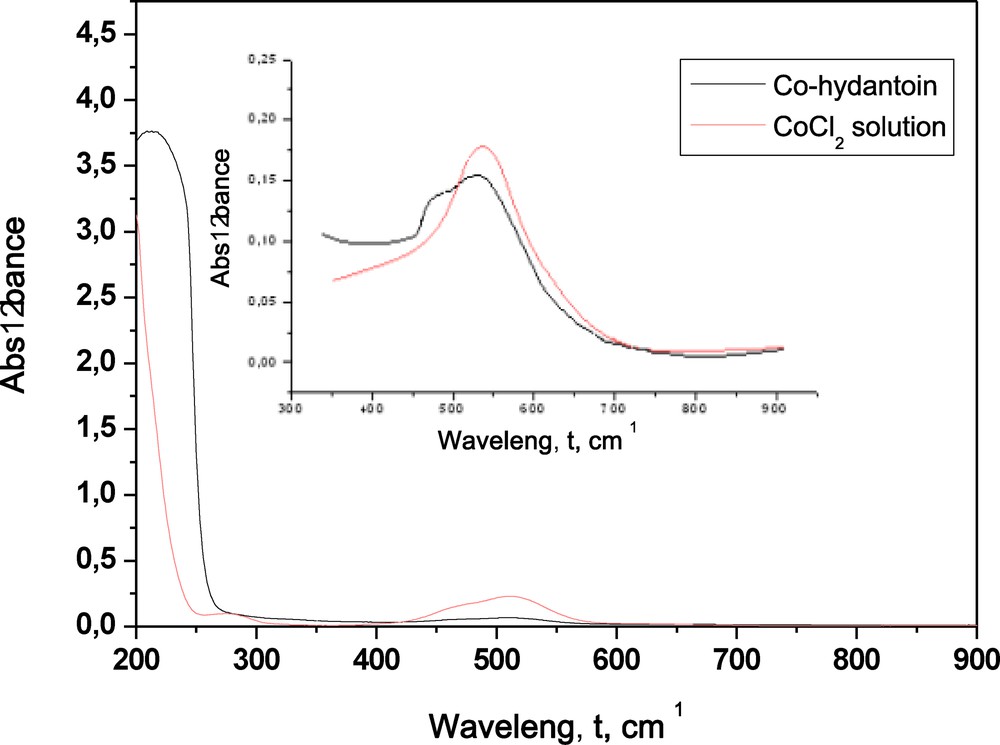
(Color online.) UV–Vis spectra of the water solution of Co(II)-aminohydantoin and the free Co(II).
The Vis spectra of copper (II) complexes with the hydantoin ligand in methanol were recorded in the range from 350 to 830 nm (Fig. 3). The spectral maxima of CuCl2 and of the free hydantoin ligand in methanol were not found in the analyzed wavelength range. One spectral maximum was observed at wavelength 760 nm after complexation of copper (II) with the hydantoin derivative at pH 3 and pH 7. As can be seen (Fig. 3), the spectra of the copper complexes obtained at pH 3 and pH 7 are identical. Moreover, the change in pH from 3 to 7.5 does not cause effects on the absorbtion maxima of the complex of copper (II) with hydantoin (Fig. 3, inset).
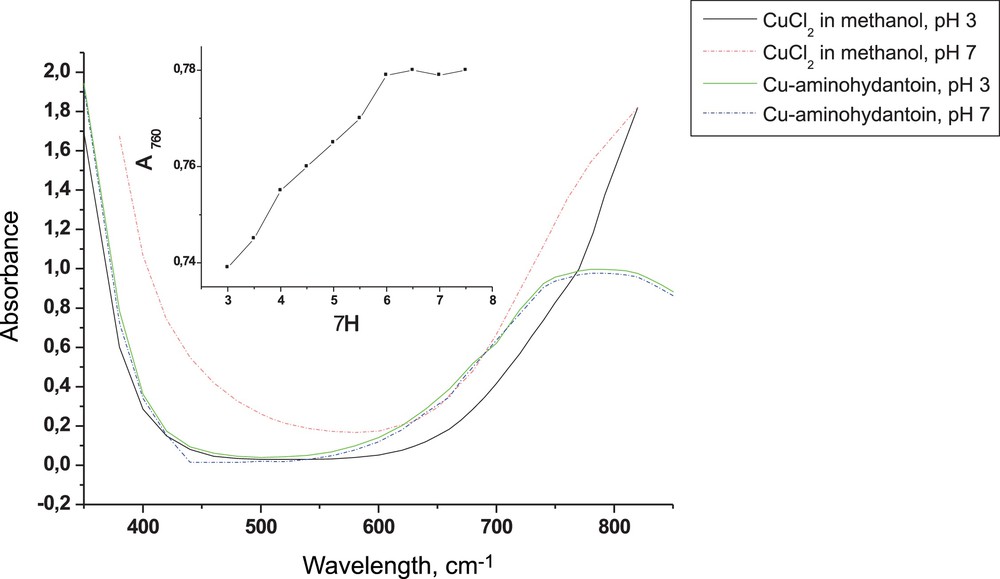
(Color online.) Vis spectra of the methanol solution at pH 3 and 7 of Cu(II)-aminohydantoin and free metal ion Cu(II).
2.4 Voltamperometry
Cyclic voltamperometry was used in the study of the interfacial and redox behavior of complexes Cu(II)-aminohydantoin, Co(II)-aminohydantoin as well as that of the free ligand (3-amino-5,5-dymethylhydantoin). Fig. 4 shows cyclic voltammograms for 1.124 × 10−5 mol·L−1 Cu(II) in 0.1 mol·L−1 sodium acetate acetic buffer (pH = 4.8 ± 0.1) in the presence and the absence (Fig. 4) of 2.248 × 10−5 mol·L−1 of hydantoin ligand. The representative voltammograms of Cu(II) in sodium acetate acetic buffer correspond well to what is known from the literature [21] and the cathodic (

(Color online.) Cyclic voltammograms of 1.124 × 10−5 mol·L−1 Cu(II) in a 0.1 mol·L−1 sodium acetate acetic buffer (blue curve) and in the presence of 2.248 × 10−5 mol·L−1 of the hydantoin ligand (black curve). Scan rate: 0.4 V·s−1. Inserted graphic: cyclic voltammograms of the complex of Cu(II) with 3-amino-5,5-dimethylhydantoin at different scan rates.
A cathodic peak shifted upon chelation of 1.818 × 10−5 mol·L−1 of Co(II) with 4.495 × 10−5 mol·L−1 of hydantoin ligand (nCo(II):nligand = 1:2) was observed at potentials more negative than –1.20 V vs Ag/AgCl in cyclic voltammetry at a scan rate of 0.4 V·s−1, as shown by the blue curve in Fig. 5. The voltammogram reveals that an intermediate cobalt complex, which may intervene during the reduction of [Co(Hyd)2]2–, should readily disproportionate, giving rise to the single irreversible cathodic wave. The black curve in Fig. 5 demonstrates that the cobalt ion in the absence of the hydantoin ligand is reduced at more positive potentials [22], suggesting that hydantoin does not undergo ligand exchange, at least during the course of the voltammetric experiment, and hence, [Co(Hyd)2]2– is sufficiently inert in alkaline solutions. The complex was indeed stable in water and existed for days at pH 8.

(Color online.) Cyclic voltammograms of 1.818 × 10−5 mol·L−1 Co(II) in a 0.08 mol·L−1 ammonium buffer (black curve) and in the presence of 4.495 × 10−5 mol·L−1 of 3-amino-5,5-dimethylhydantoin (blue curve). Scan rate: 0.4 V·s−1.
Cyclic voltammetry was performed on the 3-amino-5,5-dimethylhydantoin solution in the 0.08 mol·L−1 ammonium buffer and in the 0.1 mol·L−1 sodium acetate acetic buffer solution also. The profiles were similar to the blank profiles obtained for the supporting electrolyte solutions (0.08 mol·L−1 ammonium buffer and 0.1 mol·L−1 sodium acetate acetic buffer), with no peak observed (data not shown) in the scanned range of potentials.
2.5 Biological activity
The cytotoxicity of the substances was measured in vitro, using the following cultivated human tumor cell lines. The complexes Cu(II)- and Co(II)-aminohydantoin were evaluated for their cytotoxicity to human liver carcinoma cell line HepG2 (Fig. 6), human colorectal carcinoma cell line HT29 (Fig. 7), breast cancer cells MDA-MB-231 (Fig. 8), cervical cancer cell line HeLa (Fig. 9), and normal human diploid cell Lep3 (Fig. 10) (as controls) by using the MTS – 3-(4,5-dimethylthiazol-2-yl)-5-(3-carboxymethoxyphenyl)-2-(4-sulfo phenyl)-2H-tetrazoliuminner salt – test (see Experimental section). Most interesting in their suppressive activity towards HepG2 cells are the examined compounds (Fig. 6). Although suppressing them at high concentrations, by dilution to 0.0004 mg·mL−1, the already lowered activity rises again and remains further in these values. Maybe for this reason the values of IC50 of Cu(II)-aminohydantoin are unexpected (IC50 = 0). It might be explained either by some peculiarities of the compound structure or by only the greater sensitivity of these cells. There is no ability to measure the vitality and from there – the IC50 of a reference compound, because these substances are newly synthesized ones and comprise only the prime source constituents, which are different. The decrease in the cell vitality at great dilutions suggests that by further lowering concentrations, the IC50 could be somewhere in the very low range. The HT29 cells show a similar tendency in the suppression, but to a lower extent. The IC50 values in this case are better pronounced and this is important for the further use of Cu(II)- and Co(II)-aminohydantoins as therapeutics (Table 1). The MDA-MB-231 cells although suppressed at high concentrations do not show any further reaction after that. They seemed to be much less affected by both compounds (supported by the IC50). The HeLa cells are the strongest (in comparison with all other cell kinds) that can be suppressed by the complex of Cu(II)-aminohydantoin at its highest concentration (IC50 1.57 ± 0.47), but immediately after that they are no more influenced. It is well known that this kind of cells is sensitive to a wide range of chemotherapeutics and that only the proper amount should be selected. The normal Lep3 cells differ not too much in their sensitivity, but the good thing is that their EC50/IC50 are in the lower range, which supposes the stability of these cells at lower concentration (Table 1, Fig. 10). This last finding is very important because the normal cells should not be affected (or weakly affected) by simultaneous treatment in vivo. The fact that the normal Lep3 cells are suppressed by the complexes almost as much as the Hep G2 and Hep G2 cells should convince us that these compounds could not be used as chemotherapeutics against them.

Cytotoxic activity of the tested complexes Cu(II)- and Co(II)-aminohydantoin on a human liver carcinoma cell line HepG2.

Cytotoxic activity of the tested complexes Cu(II)- and Co(II)-aminohydantoin on a human colorectal carcinomacell line HT29.

Cytotoxic activity of the tested complexes Cu(II)- and Co(II)-aminohydantoin on breast cancer cells MDA-MB-231.

Cytotoxic activity of the tested complexes Cu(II)- and Co(II)-aminohydantoin on a cervical cancer cell line HeLa.

Cytotoxic activity of the tested complexes Cu(II)- and Co(II)-aminohydantoin in a normal human diploid cell Lep3.
In vitro cytotoxicity.
IC50 ± SE (μM) | ||
Compounds | ||
Cell lines | Cu(II)-aminohydantoin | Co(II)-aminohydantoin |
HT29 | 1.69 ± 0.98 | 5.23 ± 0.18 |
HepG2 | ND | 2.36 ± 1.8 |
HeLa | 1.57 ± 0.42 | 1.12 ± 0.98 |
MDA-MB-231 | 2.88 ± 0.97 | 1.35 ± 0.92 |
Lep-3 | 1.34 ± 0.90 | 1.33 ± 0.96 |
The MTS measured vitality of the examined cells differs quite a lot depending on the cell type and on the used substance. The HepG2 cells are suppressed by the two compounds at high concentrations, but at very low concentrations a new increase in the suppression ability begins. This is reflected by their EC50/IC50 values. The activity of the two substances on the cells is similar to the HepG2 influence on the cells, but the Co(II)-aminohydantoin is weaker at high concentration, whereas at low concentrations Cu(II)-aminohydantoin is suppressing the cells earlier (with lowering the concentration) than Cu(II)-aminohydantoin. This correlates well with the higher IC50 value of the compound (Cu(II)-aminohydantoin–IC50 = (1.69 ± 0.98) × 10−6; Co(II)-aminohydantoin–IC50 = (5.23 ± 0.18) × 10−6). Concerning the MDA-MB-231 cells, both compounds show a strong suppression effect against the cells only at high concentrations, whereas Co(II)-aminohydantoin shows better activity. The HeLa cells are more sensitive to Cu(II)-aminohydantoin, but both substances suppress the cell vitality only at a dilution of 4 mg·mL−1. The Lep 3 cells are negatively influenced by the compounds again at high concentrations (with prevalence of Co(II)-aminohydantoin).
3 Conclusion
Compounds [Cu(H2O)(Hyd)2]Cl2 (at different pH values) and [Co(H2O) (Hyd)2]Cl2·NH3·5H2O were synthesized and characterized. We have demonstrated that the newly synthesized compounds possessed cytotoxic activity against human malignant cell lines. As expected, the substances showed different activities depending on the cell line and the amount of compound used. Pronounced cytotoxic effects against HepG2 cells were observed with Cu(II)-aminohydantoin, as well against MDA-MB-231 cells with Co(II)-aminohydantoin. The most effective agents against HT29 and HeLa cells were Cu(II)-aminohydantoin Cu(II)-aminohydantoin, respectively, at the highest concentrations. Therefore, both molecular complexes demonstrated the most outstanding toxic effect towards all examined human tumour cell lines, although they having no cytotoxicity against the control below 0.4 mg·mL−1. This is not exactly the case with Cu(II)- and Co(II)-aminohydantoin, which are non-toxic to the tumour cells, but suppress the proliferation of the HepG2 cells (proliferation up to 100%). All our results did not allow us to link a specific activity (cytotoxicity or induction of the proliferative response) to the presence or the absence of metal ion in the molecule.
4 Experimental
4.1 General remarks
All other reagents and solvents were analytical grade and were bought from Merck (Germany). The 3-amino-5,5-dimethylimidazolidine-2,4-dione (ligand) was prepared as described previously [20]. The infrared (IR) spectra were recorded in KBr pellets with a PerkinElmer Model 1600 Series FT–IR instrument. A double-beam spectrophotometer Cary (Varian) was used to record the UV–Vis spectra of all compounds using 1-cm-path-length quartz cells. The cyclic voltammograms were recorded with a Metrohm 797 VA trace analyzer and a 797 VA stand. The Ag/AgCl, (3 mol·L−1) KCl electrode was used as a reference electrode, a hanging mercury drop electrode (HMDE) as a working electrode, and a carbon electrode as an auxiliary electrode. Elemental analyses (C, H, and N) were performed by standard micro-methods using the EuroEA Elemental Analyser.
4.2 General procedure for the synthesis of the complexes
4.2.1 Synthesis of the complex [Cu(H2O)(Hyd)2]Cl2 at pH ≈ 3
Crystals of [Cu(H2O)(Hyd)2]Cl2 were prepared as follows: 0.5 mmol (0.08268 g) of CuCl2·L2H2O (Merck, 99.5%) dissolved in 10 mL of methanol was added dropwise to 1 mmol (0.1313 g) of the hydantoin derivative dissolved in a mixture of 10 mL of the same solvent and 0.2 mL of aqua solution of HCl (0.1 mol·L−1). The resulting solution was placed in a vacuum desiccator over anhydrous CaCl2 at room temperature. Within one week, blue crystals precipitated from the solution. They were filtered off, rapidly washed with methanol, water, and ether, and dried in a vacuum desiccator over CaCl2. The crystals are stable at room temperature and soluble in alcohol and water. All chemicals were analytical-grade reagents. Yield: 68%. Anal. calcd. for C10H18Cl2CuN6O5 (Mr = 436.74 g·mol−1): C, 27.50; H, 4.15; Cl, 16.24; Cu, 14.55; N, 19.24; O, 18.32%. Found: C, 27.51; H, 4.74; N, 20.05%.
4.2.2 Synthesis of the complex [Cu(H2O)(Hyd)2]Cl2 at pH ≈ 7
A Cu(II) complex of 3-amino-5,5-dimethylhydantoin at pH 7 was obtained from methanol solutions of the ligand (0.1662 g) and the corresponding metal chloride (0.08745 g) mixed in metal-to-ligand ratio = 1:2, and then two drops of an aqueous solution of NaOH (0.1 mol·L−1) were added. The blue complex was obtained as a crystal precipitate, which was further filtrated, repeatedly washed with methanol and dried over CaCl2 for two weeks. The crystals are stable at room temperature and soluble in alcohol and water. All chemicals were analytical grade reagents. Yield: 62%. Anal. calcd. for C10H18Cl2CuN6O5 (Mr = 436.74 g·mol−1): C, 27.50; H, 4.15; Cl, 16.24; Cu, 14.55; N, 19.24; O, 18.32%. Found: C, 27.49; H, 4.70; N, 19.85%.
4.2.3 Synthesis of [Co(H2O) (Hyd)2]Cl2·NH3.5H2O
Complex 2 was prepared by dissolving 1 mmol (0.1471 g) of 3-amino-5,5-dimethylhydantoin in 10 mL of a 0.1 mol·L−1 ammonium buffer solution (pH = 8.0 ± 0.1), and mixed with 10 mL of a hot ammonium solution containing 0.5 mmol (0.1208 g) of CoCl2·L6H2O. The resulting solution (pH ≈ 8) was placed in a vacuum desiccator over anhydrous CaCl2 at room temperature. Within one week, a pink precipitate was formed. The product was washed with distilled water and dried in the air. Yield: 58%. Anal. calcd. for C10H31Cl2CoN7O10 (Mr = 539.23 g·L·mol−1): C, 22.27; H, 5.79; Cl, 13.15; Co, 10.93; N, 18.18; O, 29.67%. Found: C, 22.089; H, 5.24; N, 17.57%.
4.3 Voltamperometric determination
4.3.1 Solutions
A 0.1 mol·L−1 sodium acetate acetic buffer solution (pH 4.8 ± 0.1) and 0.08 mol·L−1 ammonium buffer (pH 8.1 ± 0.1) were used as supporting electrolytes. The stock solutions of Co(II) (0.002545 mol·L−1) and 3-amino-5,5-dimethylhydantoin (0.03147 mol·L−1) were prepared by dissolution of CoCl·6H2O and aminohydantoin in water, respectively. The stock solution of Cu(II) (0.01574 mol·L−1) was prepared by dissolution of CuCl·2H2O in methanol. A working solution for copper (II)-hydantoin measuring (0.01236 mol·L−1) was prepared by dissolution of the complex of Cu(II)-aminohydantoin (0.05401 g) in methanol.
4.3.2 Procedure
A 7-ml volume of the supporting electrolytes and 5 μL of Cu(II) and 50 μL of Co(II) stock solutions were pipetted in the electrochemical cell. Oxygen was removed by pure nitrogen bubbling through the solution for 10 minutes. The voltammograms were registered with the the following parameters: working electrode, hanging mercury drop electrode (HMDE); voltage step, 5 mV. The volumes of the stock aminohydantoin solution were introduced in the cell, whereas a 10-μL volume of the Cu(II)-aminohydantoin solution was added in the cell containing the Cu(II) solution. The solutions were purged with nitrogen for 5 min each and the analytical signal was registered again.
4.4 Biological activity
4.4.1 MTS test
The cells were seeded in 96-well flat-bottomed microplates (Orange scientific) at a concentration of 2 × 104 cells/well. After 24 h, the cells were covered with a DMEM medium containing different concentrations of the tested compound. All cells were incubated with 10-fold dilutions of 4 mg/mL (mother solution). Each concentration was applied in three wells. The samples of cells grown in a non-modified medium served as a control. After 24 h of incubation, the MTS colorimetric assay of cell survival was performed as described in the protocol of “Promega”. This consisted of a 24-h incubation with a MTS solution at 37 °C under 5% of carbon dioxide and 95% of air. The absorbance of each well at 490 nm was read by an automatic microplate reader (Absorbance Reader “Tecan”, Austria). Relative cell viability, expressed as a percentage of the untreated control (100% viability), was calculated for each concentration. Concentration–response curves were constructed by Excel for each experiment. All data points represent an average of three independent assays. CellTiter 96 Non-Radioactive Cell proliferation assay, Technical Bulletin #TB112, Promega Corporation USA. Revised 12/99.
4.4.2 Statistical analysis
Statistical deviations were calculated automatically using Excel 2007 and IC50 using Origin PC software.
Acknowledgment
We gratefully acknowledge the financial support of the University of Chemical Technology and Metallurgy, Sofia, Bulgaria (contracts Nos. 11124 and 11097).